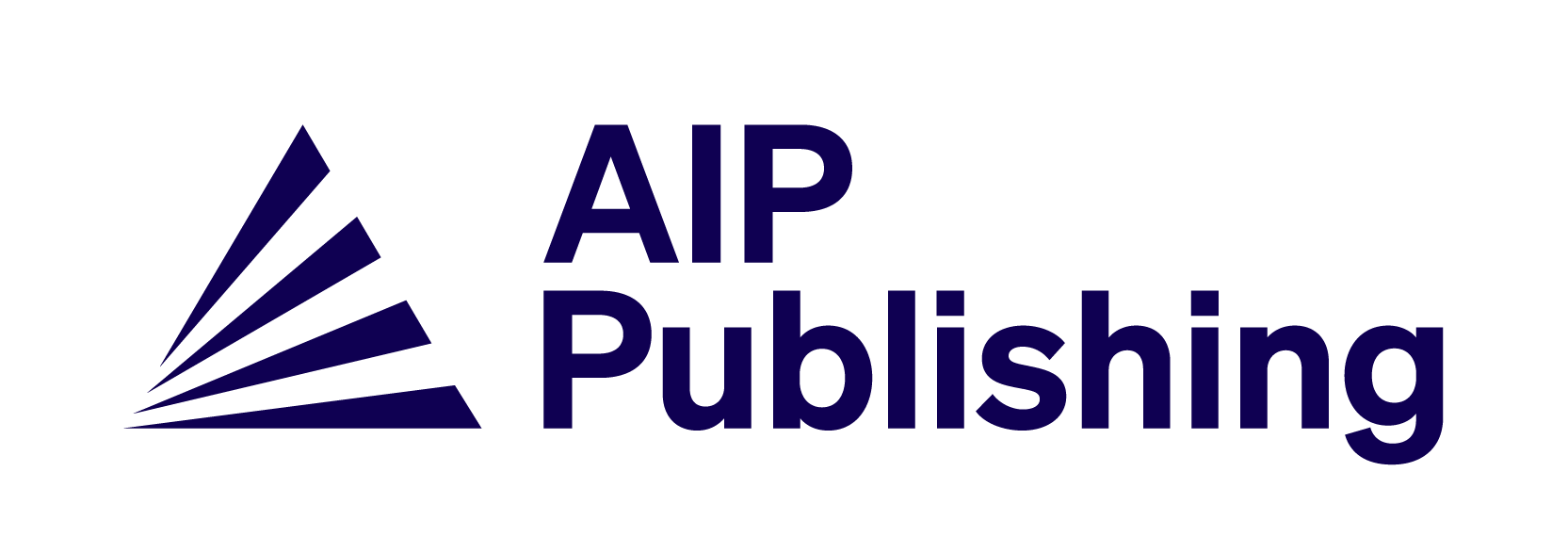
- Previous Article
- Next Article

Convergence of lattice approximations and infinite volume limit in the (λφ 4 −σφ 2 −μφ) 3 field theory
- Article contents
- Figures & tables
- Supplementary Data
- Peer Review
- Reprints and Permissions
- Cite Icon Cite
- Search Site
Yong Moon Park; Convergence of lattice approximations and infinite volume limit in the (λφ 4 −σφ 2 −μφ) 3 field theory. J. Math. Phys. 1 March 1977; 18 (3): 354–366. https://doi.org/10.1063/1.523277
Download citation file:
- Ris (Zotero)
- Reference Manager
By unified method we prove the convergence of the lattice approximation of the (λφ 4 −σφ 2 −ψφ) 3 field model with periodic, Dirichlet and Neumann boundary conditions in a finite box. This then allows us to take the inifinite volume limit of the Dirichlet states by the Nelson’s monotonicity argument. The model under consideration satisfies all the Wightman axioms except possibly the uniqueness of vacuum for μ=0 and the mass gap.
Sign in via your Institution
Citing articles via, submit your article.
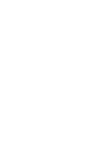
Sign up for alerts

- Online ISSN 1089-7658
- Print ISSN 0022-2488
- For Researchers
- For Librarians
- For Advertisers
- Our Publishing Partners
- Physics Today
- Conference Proceedings
- Special Topics
pubs.aip.org
- Privacy Policy
- Terms of Use
Connect with AIP Publishing
This feature is available to subscribers only.
Sign In or Create an Account
Thank you for visiting nature.com. You are using a browser version with limited support for CSS. To obtain the best experience, we recommend you use a more up to date browser (or turn off compatibility mode in Internet Explorer). In the meantime, to ensure continued support, we are displaying the site without styles and JavaScript.
- View all journals
- My Account Login
- Explore content
- About the journal
- Publish with us
- Sign up for alerts
- Open access
- Published: 17 May 2024
Disentangling the effects of structure and lone-pair electrons in the lattice dynamics of halide perovskites
- Sebastián Caicedo-Dávila ORCID: orcid.org/0000-0001-5135-2979 1 ,
- Adi Cohen 2 ,
- Silvia G. Motti 3 , 4 ,
- Masahiko Isobe ORCID: orcid.org/0000-0002-9253-7256 5 ,
- Kyle M. McCall ORCID: orcid.org/0000-0001-8628-3811 6 , 7 nAff10 ,
- Manuel Grumet 1 ,
- Maksym V. Kovalenko ORCID: orcid.org/0000-0002-6396-8938 6 , 7 ,
- Omer Yaffe 2 ,
- Laura M. Herz ORCID: orcid.org/0000-0001-9621-334X 3 , 8 ,
- Douglas H. Fabini ORCID: orcid.org/0000-0002-2391-1507 5 , 9 &
- David A. Egger ORCID: orcid.org/0000-0001-8424-902X 1
Nature Communications volume 15 , Article number: 4184 ( 2024 ) Cite this article
1 Altmetric
Metrics details
- Materials chemistry
- Materials for energy and catalysis
Halide perovskites show great optoelectronic performance, but their favorable properties are paired with unusually strong anharmonicity. It was proposed that this combination derives from the n s 2 electron configuration of octahedral cations and associated pseudo-Jahn–Teller effect. We show that such cations are not a prerequisite for the strong anharmonicity and low-energy lattice dynamics encountered in these materials. We combine X-ray diffraction, infrared and Raman spectroscopies, and molecular dynamics to contrast the lattice dynamics of CsSrBr 3 with those of CsPbBr 3 , two compounds that are structurally similar but with the former lacking n s 2 cations with the propensity to form electron lone pairs. We exploit low-frequency diffusive Raman scattering, nominally symmetry-forbidden in the cubic phase, as a fingerprint of anharmonicity and reveal that low-frequency tilting occurs irrespective of octahedral cation electron configuration. This highlights the role of structure in perovskite lattice dynamics, providing design rules for the emerging class of soft perovskite semiconductors.
Similar content being viewed by others
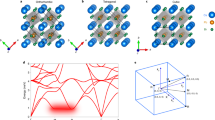
Two-dimensional overdamped fluctuations of the soft perovskite lattice in CsPbBr3
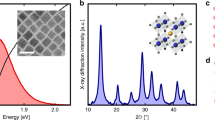
Two-dimensional electronic spectroscopy reveals liquid-like lineshape dynamics in CsPbI3 perovskite nanocrystals
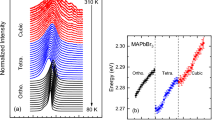
Using pressure to unravel the structure–dynamic-disorder relationship in metal halide perovskites
Introduction.
Halide perovskites (HaPs) with formula AMX 3 generated enormous research interest because of their outstanding performance in optoelectronic devices, most notably in efficient solar cells 1 , 2 , 3 . These compounds are highly unusual among the established semiconductors because they feature an intriguing combination of properties. Strong anharmonic fluctuations 4 , 5 , 6 in these soft materials appear together with optoelectronic characteristics that are favorable for technological applications 7 , 8 . This confluence raised puzzling questions regarding the microscopic characteristics of the materials and the compositional tuning of their properties alike. On the one hand, the soft anharmonic nature of the HaP structure may be beneficial in self-healing mechanisms of the material 9 , 10 , 11 , allowing for low-energy synthesis routes in their fabrication. On the other hand, pairing of anharmonic fluctuations and optoelectronic processes for key quantities of HaPs, e.g ., band gaps 12 , 13 , 14 , 15 , optical absorption profiles 16 , 17 , 18 , and charge-carrier mobilities 8 , 19 , 20 , 21 , 22 , 23 , 24 , 25 , exposed incomplete microscopic rationales for the fundamental physical processes involved in solar-energy conversion. Established materials design rules are now being challenged by these observations, opening a gap in our protocols for making improved compounds.
Significant efforts are now underway to discern the chemical effects giving rise to these remarkable properties of HaPs. Because lattice dynamical and optoelectronic properties appear both to be special and coupled in unusual ways, a common origin in chemical bonding could underlie these phenomena. In this context, an interesting chemical feature is that the octahedral cations in these compounds often bear an n s 2 electron configuration ( e.g ., Pb 2+ with configuration [Xe]6 s 2 ), which is not present in many other semiconductors 26 . This particular aspect of HaPs leads to a strong or weak pseudo-Jahn–Teller (PJT) effect 27 , 28 , 29 , depending on the particulars of cation and anion composition and chemical pressure. The weak case influences local structure 30 , 31 , 32 , lattice dynamics 33 and ionic dielectric responses 26 , 31 , 34 , 35 , while the strong case additionally results in the formation of a stereochemically-expressed electron lone pair and impacts average crystal structures 33 , 36 , 37 , 38 . The weak PJT effect associated with 6 s 2 Pb 2+ coordinated by heavy halides plays a role in optoelectronic properties of these materials: its influence on the dielectric function can modify the Coulomb screening that is relevant for small exciton binding energies, reduced recombination rates and other key properties of HaPs 39 , 40 .
Confluences of the propensity for lone-pair formation with structural and lattice-dynamical properties were investigated in previous work exploring the chemical space of HaPs. Gao et al. 33 found an inverse relationship between the Goldschmidt tolerance factor, t 41 , and anharmonic octahedral tilting motions. Similarly, Huang et al. varied the A-site cation to explore interrelations of chemical, structural, and dynamical effects in HaPs 35 , reporting t -induced modulations of octahedral tiltings and lone-pair stereoactivity. A recent study by several of the present authors found that Cs 2 AgBiBr 6 lacks some expressions of lattice anharmonicity found in other HaP variants 42 . Because every other octahedral cation (Ag + , 4 d 10 ) cannot form a lone pair in this compound, this raised the possibility that changing the electron configuration of the cations may also suppress certain aspects of the lattice dynamics in HaPs. Taken together, previous work assigned a central role of the n s 2 electron configuration and associated PJT effect in the anharmonic lattice dynamics of HaPs in addition to their established effect on the electronic structure and dielectric screening. However, exploring the chemical space of HaPs in this way simultaneously changes their structures. Therefore, isolating the convoluted occurrences of cation lone-pair formation propensity and purely structurally-determined changes in the lattice dynamics of HaPs remained challenging, making an assessment of the precise impact of chemical bonding on anharmonicity in these soft semiconductors largely inaccessible.
Here, we address this issue and show that an n s 2 cation compatible with lone-pair formation is not required for the strong anharmonicity in the low-energy lattice dynamics of soft HaP semiconductors. We disentangle structural and chemical effects in the lattice dynamics of HaPs by comparing the well-known CsPbBr 3 with the far less studied CsSrBr 3 . Both exhibit almost identical geometrical and structural parameters, but CsSrBr 3 exhibits a negligible PJT effect on the octahedral Sr 2+ site, owing to substantially weaker vibronic coupling to degenerate excited states than in the Pb 2+ case (see Supplementary Note 1) , allowing separation of the effects of the n s 2 electron configuration and the geometry on the lattice dynamics in a direct manner. Combining electronic structure and molecular dynamics (MD) calculations with X-ray diffraction (XRD), infrared (IR) and Raman spectroscopies, we assess a key fingerprint of vibrational anharmonicity, i.e ., the Raman central peak, which is a broad peak towards zero frequency in the Raman spectrum resulting from diffusive inelastic scattering 26 , 33 , 34 , 35 , 43 , 44 , 45 . While the electronic structure and dielectric properties of CsPbBr 3 and CsSrBr 3 are very different, their vibrational anharmonicities are found to be remarkably similar. In particular, the crucial dynamic octahedral tiltings giving rise to the Raman central peak are still present even in the absence of n s 2 octahedral cations in CsSrBr 3 . Our results provide microscopic understanding of precisely how the propensity for lone-pair formation influences the anharmonic octahedral tiltings that dynamically break the average cubic symmetry in both compounds, and rule out the weak PJT effect associated with the n s 2 main-group cations as the sole reason for the appearance of such anharmonicity in soft HaPs. These findings are important for chemical tuning of HaPs needed for new materials design.
Electronic structure and bonding
We first investigate the electronic structure and bonding of CsPbBr 3 and CsSrBr 3 using density-functional theory (DFT). Figure 1 shows their band structure, total and projected density of states (DOS), as well as the total and projected crystal-orbital Hamilton population (COHP) of the high-temperature cubic phases of CsPbBr 3 and CsSrBr 3 . The electronic band structure and bonding of CsPbBr 3 were extensively investigated before 46 : the conduction band minimum (CBM) is formed by anti-bonding interactions (positive COHP in Fig. 1 c) between Pb-6 p and Br-4 p /Br-4 s orbitals, while the valence band maximum (VBM) is formed by anti-bonding interactions between Br-4 p and Pb-6 s orbitals.
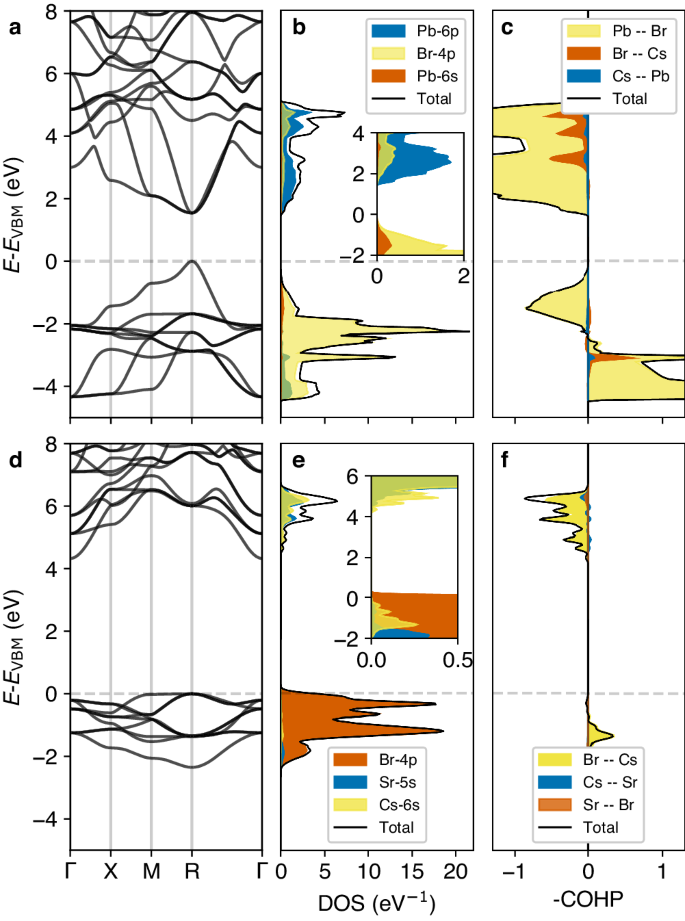
DFT-computed electronic band structure of cubic CsPbBr 3 a and corresponding total and projected density of states (DOS, b ) and crystal-orbital Hamilton population (COHP, c ). Panels d – f show the same data for CsSrBr 3 .
The electronic structure of CsSrBr 3 exhibits entirely different characteristics 26 , 47 , especially a much larger band gap and weaker covalent interactions. Notably, the magnitude of the COHP is significantly reduced with respect to that of CsPbBr 3 , indicating much greater ionicity, and the COHP is almost entirely recovered by interactions between Cs and Br. Importantly, all bands derived from antibonding interactions between Sr-5 s and Br-4 p /Br-4 s are empty due to the electron configuration of Sr 2+ ([Kr]), and there is no potential for lone pair formation on Sr 2+ . A manifestation of the lack of n s 2 cations in CsSrBr 3 is that there is no cross-gap hybridization of the halide valence orbitals. By contrast, Br-4 p orbitals hybridize with Pb-6 p across the gap of CsPbBr 3 (see the pCOHP in Fig. 1 c). This leads to large Born effective charges, i.e ., large changes in the macroscopic polarization upon ionic displacements 48 , 49 , 50 , 51 reported in Table 1 , which for CsPbBr 3 are more than double the formal charge of Pb (+2) and Br (-1) and much larger than the corresponding values for CsSrBr 3 . Similarly, there is also a larger electronic contribution to the dielectric response in CsPbBr 3 and it features a larger value of the dielectric function at the high-frequency limit ( ε ∞ ) compared to CsSrBr 3 .
Structural properties and phase transitions
In spite of the markedly different electronic structure and bonding characteristics, CsSrBr 3 and CsPbBr 3 exhibit the same high-temperature cubic crystal structure ( \(Pm\bar{3}m\) ) and very similar lattice parameters (see Supplementary Note 3) . One can rationalize this through the nearly identical ionic radii of Pb 2+ and Sr 2+ (119 and 118 pm) and the resulting Goldschmidt factors for the compounds (0.862 and 0.865). Furthermore, both materials exhibit the same sequence of structural phase transitions from the high-temperature cubic to the low-temperature orthorhombic phase (with an intermediate tetragonal phase), as shown by temperature-dependent lattice parameters in Fig. 2 that were determined via XRD. The cubic-to-tetragonal phase transition temperature of CsSrBr 3 (~520 K) is noticeably higher than that of CsPbBr 3 (~400 K) 52 , 53 and slightly higher (~10 K) than that reported for Eu-doped CsSrBr 3 :Eu 5% 54 . The volumetric thermal expansion coefficient ( α V ) of CsSrBr 3 ( ~ 1.32 × 10 −4 K −1 at 300 K) is large and similar to that of CsPbBr 3 ( ~ 1.29 × 10 −4 K −1 , see the Supplementary Note 3) , in good agreement with the one reported for CsSrBr 3 :Eu 54 . Just as for other inorganic HaPs, α V of CsSrBr 3 slightly decreases with temperature 55 , 56 . The similarity of geometric factors and structural phase transitions suggests that the octahedral tilting dynamics in CsSrBr 3 might be similar to those in CsPbBr 3 , which contrasts with their markedly different electronic structure, and prompts a deeper investigation of the impact of the n s 2 cations on structural dynamics.
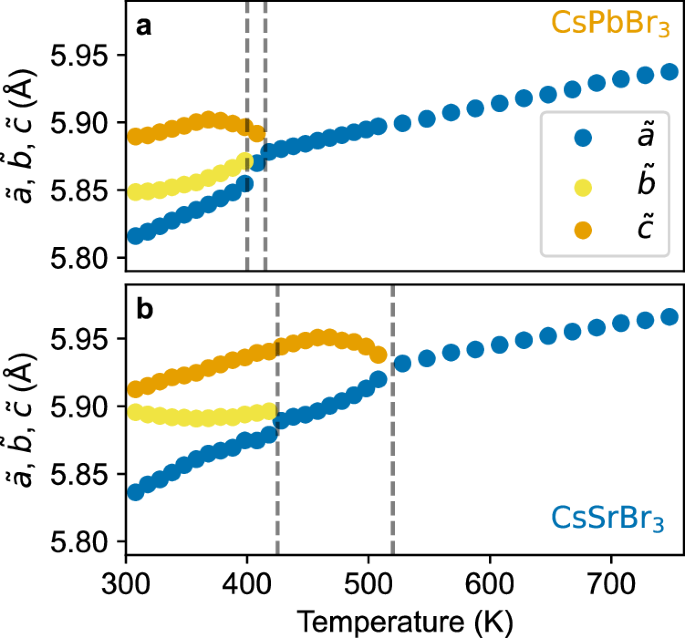
Temperature-dependent lattice parameters of CsPbBr 3 a and CsSrBr 3 b determined by XRD throughout the orthorhombic—tetragonal—cubic phases. We show reduced lattice parameters \(\tilde{a}\) , \(\tilde{b}\) and \(\tilde{c}\) for better visualization, with the orthorhombic phase expressed in the P b n m setting. Dashed vertical lines indicate phase-transition temperatures. Error bars from Pawley fitting are smaller than the markers and are omitted.
Lower-temperature lattice dynamics
We conduct IR and Raman spectroscopy at different temperatures as well as DFT-based harmonic-phonon calculations. The measured IR spectra show that the dominant CsSrBr 3 features are blue-shifted compared to those of CsPbBr 3 (see Fig. 3 a). Indeed, our DFT calculations of IR activities find a significant softening of the infrared-active TO modes in CsPbBr 3 compared to those in CsSrBr 3 (see Fig. 3 b): the most prominent IR-active TO mode in CsPbBr 3 and CsSrBr 3 appears at ~68 and 146 cm −1 , respectively, corresponding to the same irreducible representation ( B 3u ) with similar eigenvectors (see Supplementary Fig. 9) in each system. This is in line with the theory of weak PJT effects in general 29 and expectations for lone pairs in particular, with significant softening of ungerade modes in CsPbBr 3 that would correspond to lone-pair formation in the strong PJT case relative to those in CsSrBr 3 . Notably, this softening is primarily driven by differences in bonding rather than the difference in the atomic masses (see Supplementary Note 7) .
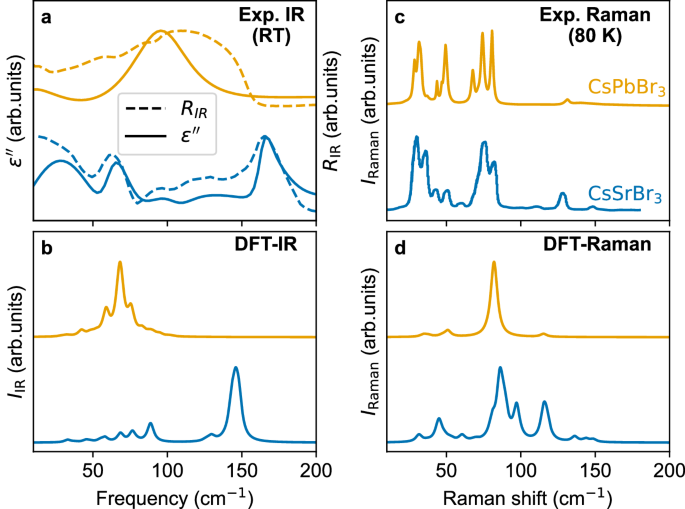
a IR-reflectivity spectra (dashed curves) and fitted imaginary part of the dielectric function (solid curves, see Supplementary Note 4 for details) of CsPbBr 3 and CsSrBr 3 measured at room temperature. b DFT-calculated IR-absorption spectra within the harmonic approximation for the orthorhombic phases. c Raman spectra of orthorhombic CsPbBr 3 and CsSrBr 3 measured at 80 K. d DFT-calculated Raman spectra of both compounds within the harmonic approximation for the orthorhombic phases.
Moreover, the LO/TO splitting is enhanced in CsPbBr 3 compared to in CsSrBr 3 and the LO phonon modes are hardened. Related to this, the CsPbBr 3 IR spectrum exhibits a broad feature which is known as the Reststrahlen band as has been reported before for MA-based HaPs 57 . This particular effect results in near-zero transmission through the material in a frequency range between the TO and LO modes, represented by high IR intensity values, and occurs in polar materials with larger Born-effective charges. Because the TO modes are softened and the LO modes hardened in CsPbBr 3 compared to CsSrBr 3 , and because the latter is less polar ( cf . Table 1 }, the absence of the n s 2 cations leads to a much less pronounced, blue-shifted Reststrahlen band appearing in a smaller frequency window in CsSrBr 3 (see Fig. 3 a, and Supplementary Note 4) .
Figure 3 c shows the 80 K Raman spectra of CsPbBr 3 and CsSrBr 3 , which are in good agreement with the Raman activities calculated for harmonic phonons (Fig. 3 d). Specifically, the experimental spectrum of CsPbBr 3 in Fig. 3 b finds three broader features at frequencies below and one weaker-intensity feature at frequencies above 100 cm −1 . Conversely, CsSrBr 3 exhibits a structured feature around 50 cm −1 , a pronounced signal close to 100 cm −1 , and then a series of weaker intensities between 100 and 150 cm −1 . While the DFT-computed Raman activities calculated in the harmonic approximation are in broad agreement with these findings (see Fig. 3 d), we note a slightly larger deviation of approximately 20 cm −1 for the higher-frequency peak in CsPbBr 3 . These findings lead us to conclude that unlike in IR, the Raman spectrum of CsSrBr 3 exhibits no substantial energy shifts with respect to CsPbBr 3 . Computing the phonon DOS for the orthorhombic phase of both compounds with DFT (see Supplementary Fig. 8) , we find that they exhibit similar phonon DOS below 100 cm −1 , i.e ., in the region of most of the Raman-active modes. The similar phonon DOS and the contributions of the M-site at low frequencies explain the limited shift of the CsSrBr 3 Raman spectrum, which might be surprising at first sight given the different atomic masses of Sr and Pb. Above this range, CsPbBr 3 exhibits few vibrational states while CsSrBr 3 shows its most pronounced phonon DOS peaks, which correspond well with the strongest IR mode calculated from the harmonic approximation.
High-temperature lattice dynamics
A key signature of vibrational anharmonicity in HaPs at higher temperatures is the Raman central peak 26 , 33 , 34 , 35 , 43 , 44 , 45 . We use this feature that is nominally symmetry-forbidden in the cubic phase as a fingerprint to directly investigate how the propensity for cation lone-pair formation or lack thereof determines anharmonicity in these materials, using Raman spectroscopy and DFT-based MD simulations. Interestingly, a central peak also appears in the high-temperature Raman spectrum of CsSrBr 3 (see Fig. 4 and Supplementary Note 5 for full temperature range). We note that differences in Raman intensity imply that the scattering cross-section of CsSrBr 3 is notably weaker than that of CsPbBr 3 , which is due to its significantly higher bandgap and weaker dielectric response at the Raman excitation wavelength (785 nm) and because a powder sample of CsSrBr 3 has been used for which scattering of light in the back-scattering direction is considerably lower. The presence of a central peak in CsSrBr 3 shows that local fluctuations associated with a cation lone-pair are not required for the low-frequency diffusive Raman scattering and anharmonicity to occur. This result, together with the identical phase-transition sequences of both materials (see Fig. 2 ), led us to investigate the role of tilting instabilities in CsSrBr 3 and CsPbBr 3 .
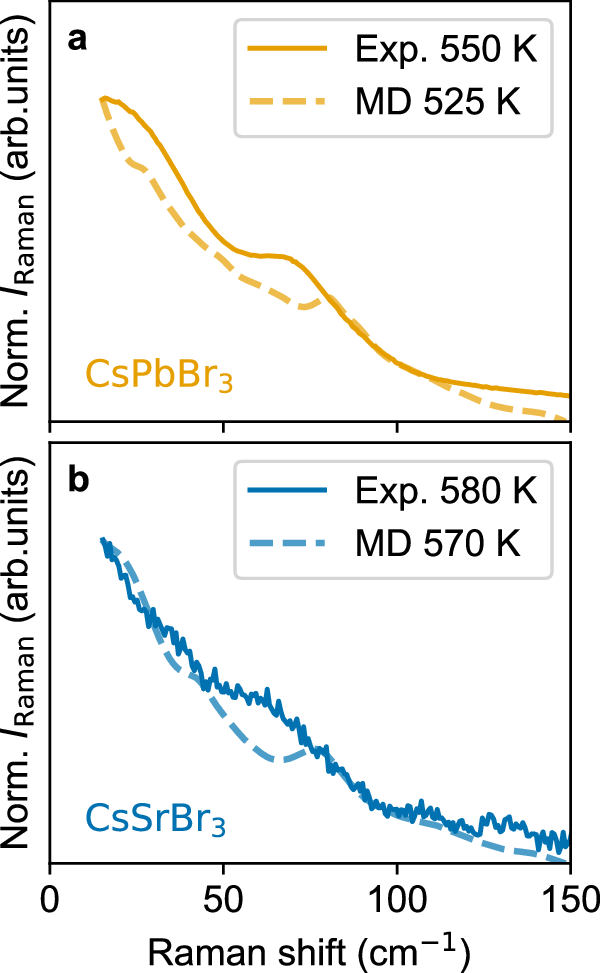
Raman spectra of CsPbBr 3 a and CsSrBr 3 b in the high-temperature cubic phase measured experimentally and calculated using DFT-MD. The central peak appears for both compounds in the experiments and computations despite significant differences in bonding: [PbBr 6 ] 4− is proximate to lone-pair formation ( i.e ., exhibits a weak PJT effect) 29 , while PJT effects associated with [SrBr 6 ] 4− are negligible.
We first calculate the Raman spectrum for both compounds using MD calculations (see Fig. 4 and Methods section). Remarkably, a central peak appears also in the MD-computed high-temperature Raman spectrum of CsPbBr 3 and CsSrBr 3 . We find good agreement between experiment and theory, both showing a feature between 50 and 100 cm −1 in the Raman spectra of the two materials in addition to the central peak.
Next, we compute harmonic phonon dispersions of both compounds (see Fig. 5 ) and find these to be remarkably similar for cubic CsSrBr 3 and CsPbBr 3 in the low frequency region, in line with the aforementioned similarities in the phonon DOS of the orthorhombic phase. Specifically, both compounds exhibit the same dynamic tilting instabilities at the edge of the Brillouin zone (BZ), governed by in-phase (M point) and three degenerate out-of-phase (R point) rotations. These rotation modes are not only active in the phase transitions, but they also have been discussed to drive the dynamic disorder of halide perovskites 4 , 14 , 58 , 59 , 60 , 61 .
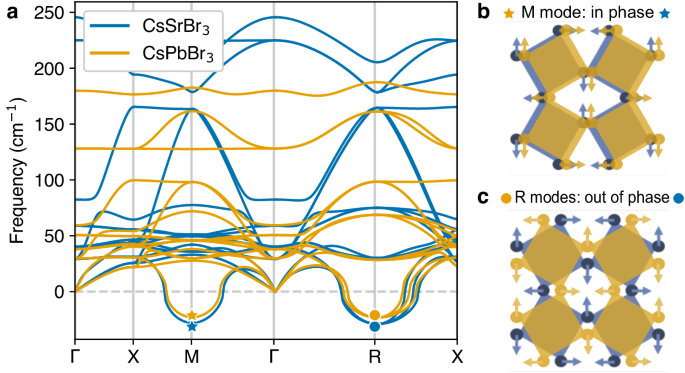
a Harmonic phonon dispersion of cubic CsPbBr 3 and CsSrBr 3 showing the dynamic instabilities in the high-temperature, cubic phase of both compounds. The imaginary modes at the M and R points are the in-phase and out-of-phase tilting depicted in b , c , respectively. The tilting modes are almost identical for CsSrBr 3 and CsPbBr 3 .
Finally, using the MD trajectories of CsPbBr 3 and CsSrBr 3 in the cubic phase, we calculate the frequency-resolved dynamic changes of octahedral rotation angles, Φ α ( ω ) (see Fig. 6 and Eq. ( 1 ) in the Methods Section). Figure 6 b shows Φ α ( ω ) for CsPbBr 3 and CsSrBr 3 and indicates strong low-frequency tilting components in both CsPbBr 3 and CsSrBr 3 . Recently, a phenomenological model for the description of the temperature-dependent Raman spectra of cubic HaPs proposed the inclusion of a low-frequency anharmonic feature, which was associated with transitions between minima of a double-well potential energy surfaces 45 that correspond to different octahedral tiltings 60 , 62 , 63 , 64 . Our results confirm that substantial octahedral dynamics correspond to low-frequency features dynamically breaking the cubic symmetry in CsPbBr 3 and CsSrBr 3 4 , 14 , 44 , 65 , 66 . Interestingly, this low-frequency component appears irrespective of the presence of n s 2 cations and induces the formation of relatively long-lived (tens of ps) structural distortions (see Supplementary Fig. 11) , which strongly deviate from the average cubic symmetry. This suggests that the dynamic deviations from the long-range, crystallographic structure enable the low-frequency Raman response without violating the selection rules.
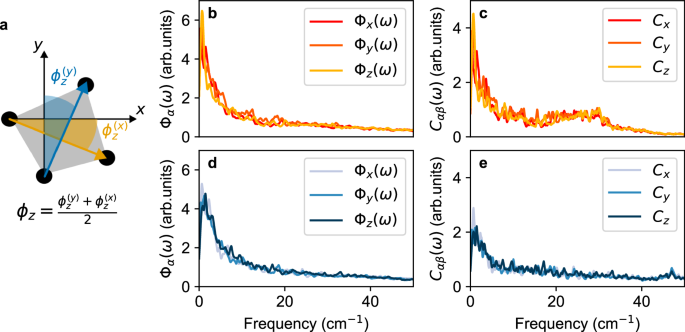
a Schematic representation of the MBr 6 octahedron aligned along the z Cartesian axis. The octahedral rotation angle around z , ϕ z , is defined as the average of the angles formed by the x / y Cartesian axis and the vector connecting two in-plane Br atoms at opposing edges of the octahedron ( \({\phi }_{z}^{(x)}\) in red and \({\phi }_{z}^{(y)}\) in blue). Note that a clockwise rotation is defined as positive and counter-clockwise as negative. Fourier transform of the octahedral rotation angle, Φ α ( ω ), and cross-correlation between rotation angle and M-site displacement, C α β ( ω ), calculated using DFT-MD trajectories of cubic CsPbBr 3 ( b , c , respectively, T = 525 K) and CsSrBr 3 ( d , e , respectively, T = 570 K).
We investigate the impact of the M-site chemistry on octahedral tilting tendencies 33 by computing the Fourier-transform of cross-correlations between rotation angles and M-site displacements, C α β ( ω ) (see Eq. ( 2 ) in the Methods section). Larger values of C α β generally indicate stronger coupling between octahedral rotations and Pb displacements. Absence of the propensity for lone-pair formation becomes evident in the low intensity of C α β ( ω ) for CsSrBr 3 (Fig. 6 c), which is less than half of that of CsPbBr 3 , especially at low-frequencies relevant for the slow, anharmonic, symmetry-breaking rotational features. This suggests that the presence of the n s 2 cations in CsPbBr 3 enhances the low-frequency octahedral tilting, in line with the literature 33 . M-site displacements and octahedral rotations are correlated because the latter is accompanied by changes of the Br-Pb-Br resonant network 17 affecting the charge density in the vicinity of the M-site. While this effect is very weak in CsSrBr 3 (see Supplementary Fig. 12) , the non-zero C α β for this case shows that the presence of n s 2 cations is not necessary to couple octahedral rotations and M-site displacements because the ions are still interacting through other types of interactions, e.g ., electrostatically or due to Pauli repulsion. In CsPbF 3 , the interaction of tilting and M-site displacements is strong enough to drive the adoption of an unusual tilt pattern 37 . We speculate that the lone-pair-enhanced tilting could contribute to the fact that CsPbBr 3 has a lower tetragonal-to-cubic phase transition temperature compared to that of CsSrBr 3 .
We directly disentangled structural and chemical effects in HaPs by comparing CsPbBr 3 and CsSrBr 3 , two compounds with similar ionic radii and structural properties but entirely different orbital interactions that imbue CsPbBr 3 with the weak PJT effect common to technologically-relevant Pb perovskites and CsSrBr 3 with negligible PJT effects. While the n s 2 configuration of the octahedral cations is paramount for the optoelectronic and dielectric properties of these materials, using the Raman central peak at higher temperatures as a fingerprint to detect anharmonicity we found it to appear also for CsSrBr 3 with 5 s 0 cations and to correlate with slow, anharmonic rotations of the octahedra. Notably, the anharmonicity of the tilting motions is different from that of intra-octahedral distortions associated with the PJT effect 29 . Altogether, these findings demonstrate that the perovskite structure allows for anharmonic vibrational dynamics to occur, irrespective of the presence of n s 2 cations with the propensity to form lone pairs, which establishes this somewhat unusual behavior as a generic effect in this material class. We note that recent work by some of the present authors has investigated the commonalities and differences between oxide perovskites and HaPs in this context 45 .
Since octahedral dynamics impact the optoelectronic characteristics of these systems, our results have implications for synthesis of new HaPs with improved properties for technological applications. For instance, Pb-Sr alloying has been proposed as a method to tune the band gap of HaPs for light emission and absorption applications 47 . Our work implies that such Sr alloying for tuning electronic and dielectric properties preserves the strongly anharmonic lattice dynamics. Furthermore, investigating related compounds with distinct electronic configurations on the octahedral cation, such as CsEuBr 3 , may provide further insight about chemical trends in tuning of the HaP properties.
The relevance of these findings for material design strategies of HaP compounds is additionally affirmed when putting our results in the context of previous work discussing anharmonic effects in this class of materials. Specifically, cubic CsPbBr 3 , CsSnBr 3 , CsGeBr 3 , (CH 3 NH 3 ) 0.13 (CH 3 CH 2 NH 3 ) 0.87 PbBr 3 , CH(NH 2 ) 2 PbBr 3 , and, here, CsSrBr 3 are all reported to exhibit dynamic hopping between low symmetry minima on the potential energy surface 33 , 34 , 35 , 67 . By contrast, the high symmetry phase of Cs 2 AgBiBr 6 is anharmonically stabilized and exhibits well-defined normal modes and a soft-mode transition on cooling 42 . Cs 2 SnBr 6 , on the other hand, lacks any phase transitions and similarly exhibits well-defined normal modes 68 . Where previously the strength of the PJT effect associated with n s 2 cations or the density of such cations appeared to be a plausible predictor of broad, nominally symmetry-forbidden Raman scattering resulting in a central peak, our work suggests that instead the differing symmetry in both the structure and the chemical bonding of metal halide perovskites and double-perovskites may be a controlling factor. Notably, CsGeBr 3 , which exhibits no octahedral tilting transitions 69 and a broad Raman central peak in the cubic phase with a mode reflecting persistent pyramidal [GeBr 3 ] − anions 33 , corresponds to the strong PJT 29 case: Stereochemically expressed cation lone pairs are evident in the low temperature average structure 69 and in the local fluctuations of the cubic phase 33 . Dynamic symmetry-breaking giving rise to a broad Raman central peak is thus observed for three distinct bonding regimes with regard to pseudo-Jahn–Teller effects: strong PJT (CsGeBr 3 ) 33 , weak PJT (CsPbBr 3 and others) 34 , and negligible PJT (CsSrBr 3 ).
In conclusion, the n s 2 electron configuration in HaPs that can result in the formation of lone-pairs is crucial to several favorable electronic features 26 , 39 , 46 and gives rise to the elevated ionic dielectric response via enhancement of Born effective charges 39 , 48 . However, we found that presence of a strong or weak PJT effect associated with n s 2 cations is not necessary to produce dynamic symmetry-breaking of the sort that gives rise to broad, intense Raman scattering in the high temperature phases of HaPs and that has been associated with the unique optoelectronic properties in these compounds such as long charge-carrier lifetimes and photoinstabilities. Instead, such dynamic symmetry breaking is common to all cubic bromide and iodide (single-)perovskites thus far studied to the best of our knowledge. These results highlight the key role of structural chemistry in the anharmonic dynamics of halide perovskites, providing an additional criterion for the design of soft optoelectronic semiconductors.
Electronic structure calculations
DFT calculations were performed with Vienna ab-initio simulation package (VASP) code 70 using the projector augmented wave (PAW) method 71 . We employed the Perdew-Burke-Ernzerhof (PBE) exchange-correlation functional 72 and the Tkatchenko-Scheffler (TS) scheme 73 —using an iterative Hirshfeld partitioning of the charge density 74 , 75 – to account for dispersive interactions. This setup has been shown to accurately describe the structure of HaPs, also in regard to the omission of SOC which impacts electronic-structure properties but does not result in significant changes of quantities related to the total energy 76 , 77 . All static calculations used an energy convergence threshold of 10 −6 eV, a plane-wave cutoff of 500 eV, and a Γ-centered k -grid of 6 × 6 × 6 (6 × 4 × 6) for the \(Pm\bar{3}m\) ( P n m a ) structures. Lattice parameters were optimized by a fitting procedure using the Birch-Murnaghan equation of state 78 , 79 The final structures used in all subsequent calculations were obtained by relaxing the ionic degrees of freedom until the maximum residual force was below 10 −4 eV Å −1 . The total and projected electronic DOS and COHP, were calculated by partitioning the DFT-calculated band structure into bonding and antibonding contributions using the LOBSTER code 80 , 81 . For this task, the DFT-computed electronic wave functions were projected onto Slater-type orbitals (basis set name: pbeVaspFit2015) 80 including Cs 6s, 5p and 5s, Pb 6s and 6p, and Br 4p and 4s states. The maximum charge spilling in this procedure was 1.3%. Spin-orbit coupling was not included in our calculations, since it is currently not supported by the LOBSTER code. We emphasize that our focus is on the orbital contributions to the (anti) bonding interactions, rather than on a quantitative descriptions of the energy.
Phonon calculations
Phonon dispersions and DOSs were obtained via the finite displacements method implemented in the phonopy package 82 . For these calculations, we used 2 × 2 × 2 supercells with 40 (160) atoms of the \(Pm\bar{3}m\) ( P n m a ) CsMBr 3 structures reducing k -space sampling accordingly. IR and Raman spectra were computed with the phonopy-spectroscopy package 83 , using zone-center phonon modes, Born-effective charges and polarizabilities, calculated with density functional perturbation theory (DFPT) 84 .
First-principles molecular dynamics
DFT-based MD calculations were performed for 2 × 2 × 2 supercells of the \(Pm\bar{3}m\) structures using a Nosé-Hoover thermostat within the canonical ensemble (NVT), as implemented in VASP 85 . The simulation temperature was set to T =525 and 570 K for CsPbBr 3 and CsSrBr 3 , respectively. An 8 fs timestep, reduced k -grid of 3 × 3 × 3, and energy convergence threshold of 10 −5 eV were used for the 10 ps equilibration and 115 ps production runs.
Raman spectra from molecular dynamics
DFT-based MD calculations were used to compute the high-temperature Raman spectra of CsPbBr 3 and CsSrBr 3 . We calculated Raman intensities from the autocorrelation function of the polarisability, as detailed elsewhere 86 . The polarizabilities were calculated with DFPT 84 on 400 evenly-spaced snapshots every 0.11 ps for a total of 44.8 ps. The k -grid employed for the DFPT calculations was set to 4 × 4 × 4 after testing convergence of the polarisability tensor for several snapshots.
Octahedral rotation dynamics and cross-correlations
We quantified the octahedral dynamics using the rotation angles, ϕ α , around a given Cartesian axis α (see Fig. 6 a). The frequency-resolved rotational dynamics were calculated as the Fourier transform of ϕ α :
where N steps is the number of snapshots. To compute the angles we selected 1000 equally spaced snapshots. We calculated the frequency-resolved cross-correlation between octahedral rotation angles (around a Cartesian direction α ) and the displacements (along a Cartesian direction β ) of the corresponding M-site, \({d}_{\beta }^{{{{{{{{\rm{M}}}}}}}}}(t)\) , as:
Polycrystalline sample preparation
CsBr (Alfa Aesar, 99.9%), anhydrous SrBr 2 (Alfa Aesar, 99%), Cs 2 CO 3 , PbO, and concentrated aqueous HBr were purchased and used as received. Guided by the reported pseudo-binary phase diagram 87 , polycrystalline CsSrBr 3 for X-ray powder diffraction and Raman spectroscopy was prepared by a solid-state reaction at 600 °C. CsBr (5 mmol, 1064 mg) and SrBr 2 (5 mmol, 1237 mg) were ground and pressed into a 5 mm diameter pellet, placed in an alumina crucible, and flame-sealed under ~ 1/3 atmosphere of argon in a fused silica ampoule. The reaction yields a porous, colorless pellet which is easily separated from the crucible and ground in inert atmosphere. Polycrystalline CsPbBr 3 for X-ray powder diffraction was prepared in ambient atmosphere by precipitation from aqueous hydrobromic acid. PbO (2 mmol, 446.4 mg) was dissolved in 2 mL hot concentrated HBr under stirring. Cs 2 CO 3 (1 mmol, 325.8 mg) was added slowly resulting in an immediate bright orange precipitate. 13 mL additional HBr was added and the mixture left to stir. After an hour, stirring was stopped and the mixture allowed to cool to room temperature. Excess solution was decanted, and the remaining mixture was evaporated to dryness on a hotplate and ground. Phase purity of all prepared compounds was established by powder XRD.
Single crystal preparation
Single crystals of CsSrBr 3 were grown by the Bridgman method from a stoichiometric mixture of the binary metal bromides in a 10 mm diameter quartz ampoule. CsSrBr 3 was pulled at 0.5 mm h −1 through an 800 °C hot zone, yielding a multi-crystalline rod from which several-mm single crystal regions could be cleaved. CsSrBr 3 is hygroscopic and all preparation and handling was performed in an inert atmosphere.
The vertical Bridgman method was used to grow large, high-quality single crystals of CsPbBr 3 . After synthesis and purification (see Supplementary Note 2 for details), the ampoule was reset to the hot zone for the Bridgman Growth. The zone 1 temperature was set to 650 °C with a 150 °C h −1 ramp rate, and held for 12 h to ensure a full melt before sample motion occurred. The zone 2 and 3 temperatures were set to 375 °C. These temperatures were held for 350 h while the ampoule was moved through the furnace at a rate of 0.9 mm h −1 under 0.3 rpm rotation. After the motion had ceased, the zone 1 temperature ramped to 375 °C to make the temperature profile in the furnace uniform. The cooling program was set to slow during the phase transitions occurring near 120 and 90 °C, with a 10 °C h −1 cooling rate from 375 °C to 175 °C, a 2.5 °C h −1 slow cooling rate from 175 °C to 75 °C, and a 10 °C h −1 rate to 30 °C. The resulting CsPbBr 3 ingot was orange-red and had large (≥5 mm) transparent single-crystalline domains, though the edges of some portions exhibited twinning.
X-ray diffraction
Polycrystalline samples were ground with silicon powder (as an internal standard and diluent) and packed in borosilicate glass capillaries. Powder XRD patterns were measured in STOE geometry using a STOE Stadi P diffractometer (Mo K α 1 radiation, Ge-(111) monochromator, Mythen 1K Detector) equipped with a furnace. Data were analyzed by sequential Pawley refinement using GSAS-II 88 .
Infrared reflectivity measurements
IR-reflection spectra in the THz range were measured as a combination of THz spectroscopy (TDS) for the low-frequency end and bolometer detection for the higher frequencies. Bolometer spectra were measured using a Bruker 80v Fourier-transform IR spectrometer with a globar source and a bolometer detector cooled to liquid He temperatures. The crystals were mounted for reflection measurements and the instrument was sealed in vacuum. A gold mirror was used as reflection reference. TDS was performed using a Spectra Physics Mai Tai-Empower-Spitfire Pro Ti:Sapphire regenerative amplifier. The amplifier generates 35 fs pulses centered at 800 nm at a repetition rate of 5 kHz. THz pulses were generated by a spintronic emitter, which was composed of 1.8 nm of Co 4 0 Fe 4 0 B 2 0 sandwiched between 2 nm of tungsten and 2 nm of platinum, all supported by a quartz substrate. The THz pulses were detected using electro-optic sampling in a (100)-ZnTe crystal. A gold mirror was used as reflection reference. The sample crystals, THz emitter and THz detector were held under vacuum during the measurements.
TDS offers better signal at low frequency, while bolometer measurements have an advantage over TDS at higher frequencies. Therefore, the spectra were combined and merged at 100 cm −1 . Owing to scattering losses, the absolute intensity of reflected light can not be taken quantitatively. Therefore, the spectra were scaled to the signal level at 100 cm −1 before merging the data. The final reflectivity spectra are given in arbitrary units. The phonon frequencies and overall spectral shape allows for fitting to the dielectric function.
Raman spectroscopy
All the measurements were taken in a home-built back scattering Raman system. For all measurements, the laser was focused with a 50× objective (Zeiss, USA), and the Rayleigh scattering was then filtered with a notch filter (Ondax Inc., USA). The beam was focused into a spectrometer 1 m long (FHR 1000, Horiba) and then on a CCD detector. To get the unpolarized Raman spectrum for the single crystals (CsSrBr 3 and CsPbBr 3 ), two orthogonal angles were measured in parallel and cross configurations (four measurements overall). The unpolarized spectrum is a summation of all four spectra. The samples were cooled below room temperature by a Janis cryostat ST-500 controlled by Lakeshore model 335 and were heated above room temperature by a closed heating system (Linkam Scientific). Due to the sensitivity of CsSrBr 3 to ambient moisture, CsSrBr 3 powder was flame-sealed in a small quartz capillary for the high-temperature measurements, and a single crystal was loaded into a closed cell under an Ar environment for the low temperatures measurements. CsSrBr 3 low temperature measurements were taken with a 2.5 eV CW diode laser (Toptica Inc.). CsSrBr 3 high-temperature measurement and all the CsPbBr 3 measurements were taken with a 1.57 eV CW diode laser (Toptica Inc.). We note that while Raman spectra on quartz show a contribution towards zero frequency 89 , it is narrower in frequency than what we observe. Results from control experiments (see Supplementary Fig. 7) show that the main signals from quartz do not contribute to the measured Raman spectra of CsSrBr 3 .
Data availability
The computational and experimental data generated in this study have been deposited in the Zenodo repository under accession code 10975217 90 .
Snaith, H. J. Perovskites: the emergence of a new era for low-cost, high-efficiency solar cells. J. Phys. Chem. Lett. 4 , 3623–3630 (2013).
Article CAS Google Scholar
Correa-Baena, J.-P. et al. Promises and challenges of perovskite solar cells. Science 358 , 739–744 (2017).
Article ADS CAS PubMed Google Scholar
Nayak, P. K., Mahesh, S., Snaith, H. J. & Cahen, D. Photovoltaic solar cell technologies: analysing the state of the art. Nat. Rev. Mater. 4 , 269–285 (2019).
Article ADS CAS Google Scholar
Beecher, A. N. et al. Direct observation of dynamic symmetry breaking above room temperature in methylammonium lead iodide perovskite. ACS Energy Lett. 1 , 880–887 (2016).
Whalley, L. D., Skelton, J. M., Frost, J. M. & Walsh, A. Phonon anharmonicity, lifetimes, and thermal transport in CH 3 NH 3 PbI 3 from many-body perturbation theory. Phys. Rev. B 94 , 1–5 (2016).
Article Google Scholar
Carignano, M. A., Aravindh, S. A., Roqan, I. S., Even, J. & Katan, C. Critical fluctuations and anharmonicity in lead iodide perovskites from molecular dynamics supercell simulations. J. Mater. Chem. C. 121 , 20729–20738 (2017).
CAS Google Scholar
Brenner, T. M., Egger, D. A., Kronik, L., Hodes, G. & Cahen, D. Hybrid organic—inorganic perovskites: low-cost semiconductors with intriguing charge-transport properties. Nat. Rev. Mater. 1 , 15007 (2016).
Egger, D. A. et al. What remains unexplained about the properties of halide perovskites? Adv. Mater. 30 , 1800691 (2018).
Ceratti, D. R. et al. Self-healing inside APbBr 3 halide perovskite crystals. Adv. Mater. 30 , 1706273 (2018).
Cahen, D., Kronik, L. & Hodes, G. Are defects in lead-halide perovskites healed, tolerated, or both? ACS Energy Lett. 6 , 4108–4114 (2021).
Parida, S. et al. Self-healing and -repair of nanomechanical damages in lead halide perovskites. Adv. Funct. Mater. 33 , 2304278 (2023).
Patrick, C. E., Jacobsen, K. W. & Thygesen, K. S. Anharmonic stabilization and band gap renormalization in the perovskite CsSnI 3 . Phys. Rev. B 92 , 201205 (2015).
Article ADS Google Scholar
Wiktor, J., Rothlisberger, U. & Pasquarello, A. Predictive determination of band gaps of inorganic halide perovskites. J. Phys. Chem. Lett. 8 , 5507–5512 (2017).
Article CAS PubMed Google Scholar
Lanigan-Atkins, T. et al. Two-dimensional overdamped fluctuations of the soft perovskite lattice in CsPbBr 3 . Nat. Mater. 20 , 977–983 (2021).
Seidl, S. A. et al. Anharmonic fluctuations govern the band gap of halide perovskites. Phys. Rev. Mater. 7 , L092401 (2023).
Gehrmann, C. & Egger, D. A. Dynamic shortening of disorder potentials in anharmonic halide perovskites. Nat. Commun. 10 , 3141 (2019).
Article ADS PubMed PubMed Central Google Scholar
Gehrmann, C., Caicedo-Dávila, S., Zhu, X. & Egger, D. A. Transversal halide motion intensifies band-to-band transitions in halide perovskites. Adv. Sci. 9 , 2200706 (2022).
Wu, B. et al. Indirect tail states formation by thermal-induced polar fluctuations in halide perovskites. Nat. Commun. 10 , 1–10 (2019).
ADS Google Scholar
Mayers, M. Z., Tan, L. Z., Egger, D. A., Rappe, A. M. & Reichman, D. R. How lattice and charge fluctuations control carrier dynamics in halide perovskites. Nano Lett. 18 , 8041–8046 (2018).
Lacroix, A., De Laissardière, G. T., Quémerais, P., Julien, J. P. & Mayou, D. Modeling of electronic mobilities in halide perovskites: adiabatic quantum localization scenario. Phys. Rev. Lett. 124 , 1–6 (2020).
Iaru, C. M. et al. Fröhlich interaction dominated by a single phonon mode in CsPbBr 3 . Nat. Commun. 12 , 5844 (2021).
Article ADS CAS PubMed PubMed Central Google Scholar
Schilcher, M. J. et al. The significance of polarons and dynamic disorder in halide perovskites. ACS Energy Lett. 6 , 2162–2173 (2021).
Lai, R. et al. Transient suppression of carrier mobility due to hot optical phonons in lead bromide perovskites. J. Phys. Chem. Lett. 13 , 5488–5494 (2022).
Zhang, K.-C., Shen, C., Zhang, H.-B., Li, Y.-F. & Liu, Y. Effect of quartic anharmonicity on the carrier transport of cubic halide perovskites CsSnI 3 and CsPbI 3 . Phys. Rev. B 106 , 235202 (2022).
Schilcher, M. J. et al. Correlated anharmonicity and dynamic disorder control carrier transport in halide perovskites. Phys. Rev. Mater. 7 , L081601 (2023).
Fabini, D. H., Seshadri, R. & Kanatzidis, M. G. The underappreciated lone pair in halide perovskites underpins their unusual properties. MRS Bull. 45 , 467–477 (2020).
Van der Vorst, C. & Maaskant, W. Stereochemically active (5s) 2 lone pairs in the structures of α -Incl and β -Incl. J. Solid State Chem. 34 , 301–313 (1980).
Maaskant, W. J. A. & Bersuker, I. B. A combined Jahn-Teller and pseudo-Jahn-Teller effect: an exactly solvable model. J. Phys. Condens. Matter 3 , 37–47 (1991).
Bersuker, I. B. Pseudo-Jahn–Teller Effect—A two-state paradigm in formation, deformation, and transformation of molecular systems and solids. Chem. Rev. 113 , 1351–1390 (2013).
Worhatch, R. J., Kim, H., Swainson, I. P., Yonkeu, A. L. & Billinge, S. J. L. Study of local structure in selected organic-inorganic perovskites in the \(Pm\bar{3}m\) phase. Chem. Mater. 20 , 1272–1277 (2008).
Fabini, D. H. et al. Dynamic stereochemical activity of the Sn 2+ lone pair in perovskite CsSnBr 3 . J. Am. Chem. Soc. 138 , 11820–11832 (2016).
Laurita, G., Fabini, D. H., Stoumpos, C. C., Kanatzidis, M. G. & Seshadri, R. Chemical tuning of dynamic cation off-centering in the cubic phases of hybrid tin and lead halide perovskites. Chem. Sci. 8 , 5628–5635 (2017).
Article CAS PubMed PubMed Central Google Scholar
Gao, L. et al. Metal cation s lone-pairs increase octahedral tilting instabilities in halide perovskites. Mater. Adv. 2 , 4610–4616 (2021).
Yaffe, O. et al. Local polar fluctuations in lead halide perovskite crystals. Phys. Rev. Lett. 118 , 1–6 (2017).
Huang, X. et al. Understanding electron–phonon interactions in 3D lead halide perovskites from the stereochemical expression of 6s 2 lone pairs. J. Am. Chem. Soc. 144 , 12247–12260 (2022).
Walsh, A., Payne, D. J., Egdell, R. G. & Watson, G. W. Stereochemistry of post-transition metal oxides: revision of the classical lone pair model. Chem. Soc. Rev. 40 , 4455 (2011).
Smith, E. H., Benedek, N. A. & Fennie, C. J. Interplay of octahedral rotations and lone pair ferroelectricity in CsPbF 3 . Inorg. Chem. 54 , 8536–8543 (2015).
Radha, S. K., Bhandari, C. & Lambrecht, W. R. Distortion modes in halide perovskites: to twist or to stretch, a matter of tolerance and lone pairs. Phys. Rev. Mater. 2 , 1–11 (2018).
Google Scholar
Du, M. H. Efficient carrier transport in halide perovskites: theoretical perspectives. J. Mater. Chem. A 2 , 9091–9098 (2014).
Herz, L. M. How lattice dynamics moderate the electronic properties of metal-halide perovskites. J. Phys. Chem. Lett. 9 , 6853–6863 (2018).
Goldschmidt, V. M. Die Gesetze der Krystallochemie. Naturwissenschaften 14 , 477–485 (1926).
Cohen, A. et al. Diverging expressions of anharmonicity in halide perovskites. Adv. Mater. 34 , 2107932 (2022).
Ferreira, A. C. et al. Direct evidence of weakly dispersed and strongly anharmonic optical phonons in hybrid perovskites. Commun. Phys. 3 , 48 (2020).
Sharma, R. et al. Elucidating the atomistic origin of anharmonicity in tetragonal CH 3 NH 3 PbI 3 with Raman scattering. Phys. Rev. Mater. 4 , 092401 (2020).
Menahem, M. et al. Disorder origin of Raman scattering in perovskite single crystals. Phys. Rev. Mater. 7 , 044602 (2023).
Goesten, M. G. & Hoffmann, R. Mirrors of bonding in metal halide perovskites. J. Am. Chem. Soc. 140 , 12996–13010 (2018).
Straus, D. B. & Cava, R. J. Tuning the band gap in the halide perovskite CsPbBr 3 through Sr substitution. ACS Appl. Mater. Interfaces 30 , 34884–34890 (2022).
Du, M.-H. & Singh, D. J. Enhanced Born charge and proximity to ferroelectricity in thallium halides. Phys. Rev. B 81 , 144114 (2010).
Sun, J. & Singh, D. J. Electronic properties, screening, and efficient carrier transport in NaSbS 2 . Phys. Rev. Appl. 7 , 024015 (2017).
Ran, Z. et al. Bismuth and antimony-based oxyhalides and chalcohalides as potential optoelectronic materials. npj Comput Mater. 4 , 14 (2018).
Kang, B. & Biswas, K. Exploring polaronic, excitonic structures and luminescence in Cs 4 PbBr 6 /CsPbBr 3 . J. Phys. Chem. Lett. 9 , 830–836 (2018).
Rodová, M., Brožek, J. & Nitsch, K. Phase transitions in ternary caesium lead bromide. J. Therm. Anal. 71 , 667–673 (2003).
Stoumpos, C. C. et al. Crystal growth of the perovskite semiconductor CsPbBr 3 : a new material for high-energy radiation detection. Cryst. Growth Des. 13 , 2722–2727 (2013).
Loyd, M. et al. Crystal structure and thermal expansion of CsCaI 3 :Eu and CsSrBr 3 :Eu scintillators. J. Cryst. Growth 481 , 35–39 (2018).
Fabini, D. H. et al. Reentrant structural and optical properties and large positive thermal expansion in perovskite formamidinium lead iodide. Angew. Chem. Int. Ed. 55 , 15392–15396 (2016).
Schueller, E. C. et al. Crystal structure evolution and notable thermal expansion in hybrid perovskites formamidinium tin iodide and formamidinium lead bromide. Inorg. Chem. 57 , 695–701 (2018).
Sendner, M. et al. Optical phonons in methylammonium lead halide perovskites and implications for charge transport. Mater. Horiz. 3 , 613–620 (2016).
Armstrong, R. L. Displacive order—disorder crossover in perovskite and antifluorite crystals undergoing rotational phase transitions. Prog. Nucl. Magn. Reson. Spectrosc. 21 , 151–173 (1989).
Woodward, P. M. Octahedral tilting in perovskites. I. Geometrical considerations. Acta Crystallogr B 53 , 32–43 (1997).
Yang, R. X., Skelton, J. M., Da Silva, E. L., Frost, J. M. & Walsh, A. Spontaneous octahedral tilting in the cubic inorganic cesium halide perovskites CsSnX 3 and CsPbX 3 (X = F, Cl, Br, I). J. Phys. Chem. Lett. 8 , 4720–4726 (2017).
Yang, R. X., Skelton, J. M., da Silva, E. L., Frost, J. M. & Walsh, A. Assessment of dynamic structural instabilities across 24 cubic inorganic halide perovskites. J. Chem. Phys. 152 , 024703 (2020).
Klarbring, J. Low-energy paths for octahedral tilting in inorganic halide perovskites. Phys. Rev. B 99 , 1–7 (2019).
Bechtel, J. S., Thomas, J. C. & Van Der Ven, A. Finite-temperature simulation of anharmonicity and octahedral tilting transitions in halide perovskites. Phys. Rev. Mater. 3 , 113605 (2019).
Zhu, X., Caicedo-Dávila, S., Gehrmann, C. & Egger, D. A. Probing the disorder inside the cubic unit cell of halide perovskites from first-principles. ACS Appl. Mater. Interfaces 14 , 22973–22981 (2022).
Wiktor, J., Fransson, E., Kubicki, D. & Erhart, P. Quantifying Dynamic Tilting in Halide Perovskites: Chemical Trends and Local Correlations. Chem. Mater. 35 , 6737–6744 (2023).
Baldwin, W. J. et al. Dynamic local structure in caesium lead iodide: spatial correlation and transient domains. Small 20 , 2303565 (2023).
Reuveni, G. et al. Static and dynamic disorder in formamidinium lead bromide single crystals. J. Phys. Chem. Lett. 14 , 1288–1293 (2023).
Kaltzoglou, A. et al. Optical-vibrational properties of the Cs 2 SnX 6 (X = Cl, Br, I) defect perovskites and hole-transport efficiency in dye-sensitized solar cells. J. Phys. Chem. C 120 , 11777–11785 (2016).
Thiele, G., Rotter, H. W. & Schmidt, K. D. Kristallstrukturen und Phasentransformationen von Caesiumtrihalogenogermanaten (II) CsGeX3 (X= Cl, Br, I). Z. Anorg. Allg. Chem. 545 , 148–156 (1987).
Kresse, G. & Furthmüller, J. Efficient iterative schemes for ab initio total-energy calculations using a plane-wave basis set. Phys. Rev. B 54 , 11169–11186 (1996).
Kresse, G. & Joubert, D. From ultrasoft pseudopotentials to the projector augmented-wave method. Phys. Rev. B 59 , 1758–1775 (1999).
Perdew, J. P., Burke, K. & Ernzerhof, M. Generalized gradient approximation made simple. Phys. Rev. Lett. 77 , 3865–3868 (1996).
Tkatchenko, A. & Scheffler, M. Accurate molecular Van Der Waals interactions from ground-state electron density and free-atom reference data. Phys. Rev. Lett. 102 , 073005 (2009).
Article ADS PubMed Google Scholar
Bučko, T., Lebègue, S., Hafner, J. & Ángyán, J. G. Improved density dependent correction for the description of London dispersion forces. J. Chem. Theory Comput. 9 , 4293–4299 (2013).
Article PubMed Google Scholar
Bučko, T., Lebègue, S., Ángyán, J. G. & Hafner, J. Extending the applicability of the Tkatchenko-Scheffler dispersion correction via iterative Hirshfeld partitioning. J. Chem. Phys. 141 , 034114 (2014).
Egger, D. A. & Kronik, L. Role of dispersive interactions in determining structural properties of organic-inorganic halide perovskites: Insights from first-principles calculations. J. Phys. Chem. Lett. 5 , 2728–2733 (2014).
Beck, H., Gehrmann, C. & Egger, D. A. Structure and binding in halide perovskites: Analysis of static and dynamic effects from dispersion-corrected density functional theory. APL Mater. 7 , 021108 (2019).
Birch, F. Finite elastic strain of cubic crystals. Phys. Rev. 71 , 809–824 (1947).
Murnaghan, F. D. The Compressibility of Media under Extreme Pressures. Proc. Natl Acad. Sci. USA 30 , 244–247 (1944).
Article ADS MathSciNet CAS PubMed PubMed Central Google Scholar
Maintz, S., Deringer, V. L., Tchougréeff, A. L. & Dronskowski, R. LOBSTER: A tool to extract chemical bonding from plane-wave based DFT. J. Comput. Chem. 37 , 1030–1035 (2016).
Nelson, R. et al. LOBSTER : Local orbital projections, atomic charges, and chemical-bonding analysis from projector-augmented-wave-based density-functional theory. J. Comput. Chem. 41 , 1931–1940 (2020).
Togo, A. & Tanaka, I. First principles phonon calculations in materials science. Scr. Mater. 108 , 1–5 (2015).
Skelton, J. M. et al. Lattice dynamics of the tin sulphides SnS 2 , SnS and Sn 2 S 3 : vibrational spectra and thermal transport. Phys. Chem. Chem. Phys. 19 , 12452–12465 (2017).
Gajdoš, M., Hummer, K., Kresse, G., Furthmüller, J. & Bechstedt, F. Linear optical properties in the projector-augmented wave methodology. Phys. Rev. B 73 , 1–9 (2006).
Kresse, G. & Hafner, J. Ab initio molecular-dynamics simulation of the liquid-metalamorphous- semiconductor transition in germanium. Phys. Rev. B 49 , 14251–14269 (1994).
Thomas, M., Brehm, M., Fligg, R., Vöhringer, P. & Kirchner, B. Computing vibrational spectra from ab initio molecular dynamics. Phys. Chem. Chem. Phys. 15 , 6608–6622 (2013).
Riccardi, R., Sinistri, C., Campari, G. Y. & Magistris, A. Binary systems formed by alkali bromides with barium or strontium bromide. Z. Naturforsch. A 25 , 781–785 (1970).
Toby, B. H. & Dreele, R. B. V. GSAS-II : the genesis of a modern open-source all purpose crystallography software package. J. Appl. Crystallogr. 46 , 544–549 (2013).
Bates, J. B. & Quist, A. S. Polarized Raman spectra of β -Quartz. J. Chem. Phys. 56 , 1528–1533 (1972).
Caicedo-Dávila, S. et al. Disentangling the effects of structure and lone-pair electrons in the lattice dynamics of halide perovskites. https://doi.org/10.5281/zenodo.10975217 (2024).
Download references
Acknowledgements
Funding provided by the Alexander von Humboldt-Foundation in the framework of the Sofja Kovalevskaja Award, endowed by the German Federal Ministry of Education and Research, by the Deutsche Forschungsgemeinschaft (DFG, German Research Foundation) via Germany’s Excellence Strategy - EXC 2089/1-390776260, and by TU Munich - IAS, funded by the German Excellence Initiative and the European Union Seventh Framework Programme under Grant Agreement No. 291763, are gratefully acknowledged. Funding was provided by the Engineering and Physical Sciences Research Council (EPSRC), UK. The Gauss Centre for Supercomputing eV is acknowledged for providing computing time through the John von Neumann Institute for Computing on the GCS Supercomputer JUWELS at Jülich Supercomputing Centre. D.H.F. gratefully acknowledges financial support from the Alexander von Humboldt Foundation and the Max Planck Society. D.H.F. thanks Maximilian A. Plass for assistance with flame-sealing the Raman capillaries. K.M.M. and M.V.K. acknowledge financial support by ETH Zürich through the ETH+ Project SynMatLab Laboratory for Multiscale Materials Synthesis.
Open Access funding enabled and organized by Projekt DEAL.
Author information
Kyle M. McCall
Present address: Department of Materials Science and Engineering, University of Texas at Dallas, Richardson, TX, USA
Authors and Affiliations
Physics Department, TUM School of Natural Sciences, Technical University of Munich, Garching, Germany
Sebastián Caicedo-Dávila, Manuel Grumet & David A. Egger
Department of Chemical and Biological Physics, Weizmann Institute of Science, Rehovot, Israel
Adi Cohen & Omer Yaffe
Clarendon Laboratory, Department of Physics, University of Oxford, Oxford, UK
Silvia G. Motti & Laura M. Herz
School of Physics and Astronomy, Faculty of Engineering and Physical Sciences, University of Southampton, Southampton, UK
Silvia G. Motti
Max Planck Institute for Solid State Research, Stuttgart, Germany
Masahiko Isobe & Douglas H. Fabini
Laboratory of Inorganic Chemistry, Department of Chemistry and Applied Biosciences, ETH Zurich, Zürich, Switzerland
Kyle M. McCall & Maksym V. Kovalenko
Laboratory for Thin Films and Photovoltaics, EMPA - Swiss National Laboratories for Materials and Technology, Dübendorf, Switzerland
TUM Institute for Advanced Study, Technical University of Munich, Garching, Germany
Laura M. Herz
Department of Chemistry, Massachusetts Institute of Technology, Cambridge, MA, USA
Douglas H. Fabini
You can also search for this author in PubMed Google Scholar
Contributions
S.C.-D. performed the theoretical calculations, analyzed the data and wrote the initial manuscript under the supervision of D.A.E and with additional support by M.G. A.C. performed Raman measurements and analyzed data under the supervision of O.Y. S.M. performed IR measurements and analyzed data under the supervision of L.M.H. D.H.F. prepared polycrystalline samples, performed XRD measurements, analyzed data, and modeled the PJT effect. M.I. prepared the CsSrBr 3 single crystals. K.M.M. prepared the CsPbBr 3 single crystals under the supervision of M.V.K. D.H.F. and D.A.E. conceived and supervised the project.
Corresponding authors
Correspondence to Douglas H. Fabini or David A. Egger .
Ethics declarations
Competing interests.
The authors declare no competing interests.

Peer review
Peer review information.
Nature Communications thanks Yongping Fu, Daniel B. Straus and the other anonymous reviewer(s) for their contribution to the peer review of this work. A peer review file is available.
Additional information
Publisher’s note Springer Nature remains neutral with regard to jurisdictional claims in published maps and institutional affiliations.
Supplementary information
Supplementary information, peer review file, rights and permissions.
Open Access This article is licensed under a Creative Commons Attribution 4.0 International License, which permits use, sharing, adaptation, distribution and reproduction in any medium or format, as long as you give appropriate credit to the original author(s) and the source, provide a link to the Creative Commons licence, and indicate if changes were made. The images or other third party material in this article are included in the article’s Creative Commons licence, unless indicated otherwise in a credit line to the material. If material is not included in the article’s Creative Commons licence and your intended use is not permitted by statutory regulation or exceeds the permitted use, you will need to obtain permission directly from the copyright holder. To view a copy of this licence, visit http://creativecommons.org/licenses/by/4.0/ .
Reprints and permissions
About this article
Cite this article.
Caicedo-Dávila, S., Cohen, A., Motti, S.G. et al. Disentangling the effects of structure and lone-pair electrons in the lattice dynamics of halide perovskites. Nat Commun 15 , 4184 (2024). https://doi.org/10.1038/s41467-024-48581-x
Download citation
Received : 05 October 2023
Accepted : 06 May 2024
Published : 17 May 2024
DOI : https://doi.org/10.1038/s41467-024-48581-x
Share this article
Anyone you share the following link with will be able to read this content:
Sorry, a shareable link is not currently available for this article.
Provided by the Springer Nature SharedIt content-sharing initiative
By submitting a comment you agree to abide by our Terms and Community Guidelines . If you find something abusive or that does not comply with our terms or guidelines please flag it as inappropriate.
Quick links
- Explore articles by subject
- Guide to authors
- Editorial policies
Sign up for the Nature Briefing newsletter — what matters in science, free to your inbox daily.

ω-convergence theory of molecular nets in ω-molecular lattices
Ieee account.
- Change Username/Password
- Update Address
Purchase Details
- Payment Options
- Order History
- View Purchased Documents
Profile Information
- Communications Preferences
- Profession and Education
- Technical Interests
- US & Canada: +1 800 678 4333
- Worldwide: +1 732 981 0060
- Contact & Support
- About IEEE Xplore
- Accessibility
- Terms of Use
- Nondiscrimination Policy
- Privacy & Opting Out of Cookies
A not-for-profit organization, IEEE is the world's largest technical professional organization dedicated to advancing technology for the benefit of humanity. © Copyright 2024 IEEE - All rights reserved. Use of this web site signifies your agreement to the terms and conditions.
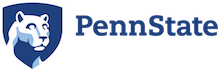
Density Functional Theory and Practice Course
Blog where students post their results, determining the lattice constants for hf.
For Project 1, the lattice constants for Hf are calculated. Since Hf is an hcp metal with c/a=1.58, we need to calculate the energy by changing the lattice constant c while keeping c/a fixed. And the optimized a, c can be obtained by minimizing the free energy.
1. The hcp structure of Hf
Figure 1 shows the unit cell of Hf hcp structure. OA, OB, OC are three lattice vectors. The lengths and angles of the lattices vectors (OA, OB, OC) are a, b, c and α, β, γ, respectively. To keep the symmetry, the constraints for the unit cell are a=b, α=β=90 degrees, γ=120 degrees.

Figure 1 The unit cell of Hf
2. The parameters for the calculations
Some important parameters and inputs for the calculation are listed as following:
Atomic structure for Hf:1s2 2s2 2p6 3s2 3p6 3d10 4s2 4p6 4d10 4f14 5s2 5p6 5d2 6s2
The core configuration of the pseudopotential: 1s2 2s2 2p6 3s2 3p6 3d10 4s2 4p6 4d10
Functional: GGA Perdew Burke Ernzerhof (PBA) functional
Pseudopotential: OTFG ultrasoft
Smearing width: 0.1
Origin shift of K points: 0, 0, 0
The k points grid: investigated in the next section
The energy cutoff: investigated in the next section
3. The convergence of the calculations
Before starting the actual calculation for the lattice constant, it is important to explore the convergence of the calculations with respect to the number of k points and the energy cutoff. So the most accurate and efficient results can be obtained. For the following calculation, the lattice constant is fixed at c= 5.0511Å, a= 3.1946Å, while the size of k point grid and the energy cutoff are varied.
(1) The number of k-points in the irreducible part of the BZ
In this section 3.1, the energy cutoff is fixed at 400 eV, and the size of k point grid changes and Number of k-points in the irreducible part of the BZ (the number of k points) varies from 1 to 162, shown in Table 1. According to Table 1, the relations between Energy/atom, calculation time and the number of k points can be plotted, which are shown in Figure 2. As shown in Figure 2, the energy/atom becomes stable with the increase of k points. However, the calculation time also increases rapidly when the number of k points increases. By combining the results of these two graphs, 9*9*6 k points with 36 k points is chosen to obtain an accurate result with less calculation time.
A little discussion here, since my calculation involves unit cells with different volumes, the k points need to be chosen so the density of k points in reciprocal space is comparable for the different supercells. But as shown in section 4, since the volumes of unit cell change just a little during the calculation, fixing the number of k points will not make much difference. So in section 4, the number of k points is chosen as 36 for all calculations.

Table 1 The calculation of different sizes of k point grid

Figure 2 The plots between Energy/atom, calculation time and the number of k points. The solid lines are just used for guide the eye.
(2) The energy cutoff
In this section, the number of k point is fixed at 36, and the energy cutoff varies from 200 to 800, shown in Table 2. And Figure 3 shows the relation between energy/atom, calculation time and energy cutoff. Although the energy/atom keeps increasing with the increase of energy cut off, the differences between each energy/atom are becoming smaller and smaller. On the other hand, the calculation time increases rapidly with the increase of energy cut off. In order to save the time and keep the accuracy, the energy cutoff is chosen as 435.4 eV.

Table 2 The calculation of different energy cutoff

Figure 3 The plots between energy/atom, calculation time and energy cutoff. The solid lines are used to guide the eye.
4 The calculation of the lattice constants
Using the results from section 3, the k points and the energy cutoff are chosen as 9*9*6 and 435.4 eV. Then lattice constants c and a are changed to obtain the minimum energy, shown in Table 3. As shown in Figure 4, the unit cell and the lattice constants can be predicted. As shown in Figure 4a, the quadratic relation between energy/atom and volume/atom only valid for a small range of lattice constants around the equilibrium value. A small range of volume/atom is chosen and the relation is re-plotted in Figure 4b. According to the fitting equation in Figure 4b, the fitting volume/atom is 22.64 Å^3 and the lattice constant are c=5.0673 Å, a=3.2072 Å. And for the experiment data, the lattice constants are a=3.1964 Å, c=5.0511 Å (1). So the calculation is accurate with an error of 0.3%.

Table 3 The calculation of energy with different lattice constants.

Figure 4 The plots between energy/atom and volume/atom. the solid lines are used to guide the eye. The dashed line is the fitting line.
5. Some discussion and future works
Although the calculation result is accurate with an error of 0.3%, there are still some ways to further increase the accuracy. First, the energy cut off can be increased so the energy/atom can be more precise. Second, we can calculate more points around the value c=5.05 Å and maybe a more accurate result can be obtained. Third, instead of using the quadratic fitting, we can use a higher order polynomial fitting.
For some future works: first, it is worthwhile changing the ratio c/a to determine the optimum value of c/a. Second, it should be interesting to analyze the behavior of energy at small or large lattice constants.
6. Reference:
(1) https://www.webelements.com/hafnium/crystal_structure.html

Leave a Reply Cancel reply
Your email address will not be published. Required fields are marked *
Save my name, email, and website in this browser for the next time I comment.
Identification of nonlinear dynamical system based on adaptive radial basis function neural networks
- Original Article
- Published: 17 May 2024
Cite this article
- Guo Luo 1 ,
- Hu Min ORCID: orcid.org/0000-0002-1382-774X 1 &
- Zhi Yang 1
In this paper, an adaptive radial basis function neural network (RBFNN) is investigated and presented for identifying the nonlinear dynamical system. In traditional RBFNN structure, the selections of the activated radius are decided by the designer’s experience to satisfy the best approximation of nonlinear dynamical function. In order to reduce the experience error, an adaptive RBFNN mechanism that can realize auto-regulation of activated radius is proposed in this paper. Taking the lattice points as the center of activated function, we use Taylor expansion to separate the factor of activated radius in local space. In order to ensure that the identification error has the property of fast convergence, the error conversion function is used for shrinking the gain in the error differential equation. Constructing a Lyapunov function to determine the differential equation of the weights and the activated radius, it is shown that the weights and the activated radius will converge to the neighborhood of its true value and the identification error will converge to neighborhood of zero in a periodic or period-like nonlinear dynamical system. To illustrate the effectiveness of the proposed adaptive RBFNN, Vanderpol and Duffing dynamical system are used as test examples, in comparison with traditional RBFNN and wavelet neural networks (WNN). The results show that the proposed method has best accurate identification and approximating effect in all of test algorithms.
This is a preview of subscription content, log in via an institution to check access.
Access this article
Price includes VAT (Russian Federation)
Instant access to the full article PDF.
Rent this article via DeepDyve
Institutional subscriptions
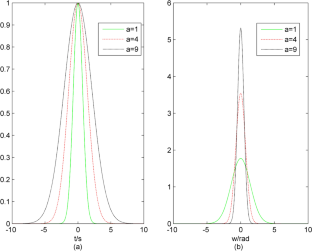
Data availability
The data used to support the findings of this study are included within the article.
Kumar R, Srivastava S, Gupta JRP, Mohindru A (2018) Diagonal recurrent neural network based identification of nonlinear dynamical systems with Lyapunov stability based adaptive learning rates. Neurocomputing 287:102–117
Article Google Scholar
Xu L, Ding F, Yang E (2020) Separable recursive gradient algorithm for dynamical systems based on the impulse response signals. Int J Control Autom Syst 18(12):3167–3177
Denis V, Jossic M, Giraud-Audine C, Chomette B, Renault A, Thomas O (2018) Identification of nonlinear modes using phase-locked-loop experimental continuation and normal form. Mech Syst Signal Process 106:430–452
Zhang S, Wang S, Jing F, Tan M (2019) A sensorless hand guiding scheme based on model identification and control for industrial robot. IEEE Trans Ind Inf 15(9):5204–5213
Gupta V, Mittal M (2020) A novel method of cardiac arrhythmia detection in electrocardiogram signal. Int J Med Eng Inf 12(5):489–499
Google Scholar
Deng M, Wang C, Zheng T (2018) Individual identification using a gait dynamics graph. Pattern Recogn 83:287–298
Alexandridis A, Chondrodima E, Giannopoulos N, Sarimveis H (2016) A fast and efficient method for training categorical radial basis function networks. IEEE Trans Neural Netw Learn Syst 28(11):2831–2836
Rahimilarki R, Gao Z, Zhang A, Binns R (2019) Robust neural network fault estimation approach for nonlinear dynamic systems with applications to wind turbine systems. IEEE Trans Ind Inf 15(12):6302–6312
Fei J, Lu C (2017) Adaptive sliding mode control of dynamic systems using double loop recurrent neural network structure. IEEE Trans Neural Netw Learn Syst 29(4):1275–1286
Puscasu G, Codres B, Stancu A, Murariu G (2009) Nonlinear system identification based on internal recurrent neural networks. Int J Neural Syst 19(02):115–125
Pan Y, Wang J (2011) Model predictive control of unknown nonlinear dynamical systems based on recurrent neural networks. IEEE Trans Ind Electron 59(8):3089–3101
Wu W, Wang C, Yuan C (2019) Deterministic learning from sampling data. Neurocomputing 358:456–466
Kurdila AJ, Narcowich FJ, Ward JD (1995) Persistency of excitation in identification using radial basis function approximants. SIAM J Control Optim 33(2):625–642
Article MathSciNet Google Scholar
Wang C, Hill DJ (2006) Learning from neural control. IEEE Trans Neural Netw 17(1):130–146
Wang C, Hill DJ (2010) Deterministic learning and nonlinear observer design. Asian J Control 12(6):714–724
Sanner RM, Slotine J (1992) Gaussian networks for direct adaptive control. IEEE Trans Neural Netw 3(6):837–863
Zheng T, Wang C (2017) Relationship between persistent excitation levels and RBF network structures, with application to performance analysis of deterministic learning. IEEE Trans Cybern 47(10):3380–3392
Schilling RJ, Carroll JJ, Al-Ajlouni AF (2001) Approximation of nonlinear systems with radial basis function neural networks. IEEE Trans Neural Netw 12(1):1–15
Luo G, Yang Z, Zhan C, Zhang Q (2021) Identification of nonlinear dynamical system based on raised-cosine radial basis function neural networks. Neural Process Lett 53(1):355–374
Abiyev RH, Kaynak O (2008) Fuzzy wavelet neural networks for identification and control of dynamic plants-a novel structure and a comparative study. IEEE Trans Ind Electron 55(8):3133–3140
Luo G, Yang Z, Zhang Q (2021) Identification of autonomous nonlinear dynamical system based on discrete-time multiscale wavelet neural network. Neural Comput Appl 33(22):15191–15203
Zeng W, Yuan C (2021) ECG arrhythmia classification based on variational mode decomposition, Shannon energy envelope and deterministic learning. Int J Mach Learn Cybern 12(10):2963–2988
Masood Z, Majeed K, Samar R, Raja MAZ (2017) Design of Mexican Hat Wavelet neural networks for solving Bratu type nonlinear systems. Neurocomputing 221:1–14
Wirkus S, Rand R (2015) The dynamics of two coupled van der pol oscillators with delay coupling. Nonlinear Dyn 30(3):205–221
Zhang Q, Gao J, Dong H, Mao Y (2018) Wpd and de/bbo-rbfnn for solution of rolling bearing fault diagnosis. Neurocomputing 312(27):27–33
Agarwal V, Zheng X, Balachandran B (2018) Influence of noise on frequency responses of softening Duffing oscillators. Phys Lett A 382(46):3355–3364
Download references
Acknowledgements
The authors would like to thank anonymous peer reviewer.
Author information
Authors and affiliations.
School of Electrics and Computer Engineering, Nanfang College Guangzhou, Guangzhou, China
Guo Luo, Hu Min & Zhi Yang
You can also search for this author in PubMed Google Scholar
Corresponding author
Correspondence to Hu Min .
Ethics declarations
Conflict of interest.
The authors (Guo Luo, Hu Min and Zhi Yang) declare that they have no conflict of financial or non-financial interests in relation to the work in this article.
Additional information
Publisher's note.
Springer Nature remains neutral with regard to jurisdictional claims in published maps and institutional affiliations.
Rights and permissions
Springer Nature or its licensor (e.g. a society or other partner) holds exclusive rights to this article under a publishing agreement with the author(s) or other rightsholder(s); author self-archiving of the accepted manuscript version of this article is solely governed by the terms of such publishing agreement and applicable law.
Reprints and permissions
About this article
Luo, G., Min, H. & Yang, Z. Identification of nonlinear dynamical system based on adaptive radial basis function neural networks. Neural Comput & Applic (2024). https://doi.org/10.1007/s00521-024-09794-9
Download citation
Received : 17 November 2022
Accepted : 25 March 2024
Published : 17 May 2024
DOI : https://doi.org/10.1007/s00521-024-09794-9
Share this article
Anyone you share the following link with will be able to read this content:
Sorry, a shareable link is not currently available for this article.
Provided by the Springer Nature SharedIt content-sharing initiative
- Adaptive radial basis function neural networks
- Identification of nonlinear dynamical system
- Activated radius
- Lyapunov function
- Find a journal
- Publish with us
- Track your research

IMAGES
VIDEO
COMMENTS
functions and order convergence of nets in vector lattices. The theory of convergence structures provides a framework for study-ing more general modes of convergence. It also has one particu-larly striking feature: it is formalized using the language of filters. This paper develops a general theory of convergence in terms of nets.
Yang-Mills theory on lattice15 / 41 lattice action for gauge field configuration U = fU x; g S W(U) = 1 g2N X p tr ... p + U y p ˙ (Wilson). in particular for G= SU(2) S W = 1 2g2 X p tr(1 U p) improved lattice action (Symanzik) S YM S Sy = O(a2))faster convergence to continuum limit a !0 Andreas Wipf (TPI Jena) Lattice Gauge Theories - An ...
Moore-Smith convergence theory is enriched in a fuzzy lattice. The σ-limit point and σ-cluster point of a molecular net and a filter in a fuzzy lattice are defined and their various properties are discussed, and the σ-closure, the σ-interior operators and σ-topological molecular lattices are introduced by the concept of ordered pair of R-neighborhoods.
This chapter gives an introduction to Lattice Gauge Theories (LGT). After an introduction into the history of the subject beginning with Wegner's \(Z_2\) LGT, the three pillars of Euclidean LGT's, namely, (i) the path-integral formulation of QFT's, (ii) Euclideanization and (iii) discretization, are very carefully discussed. The exact mapping between QFT's in D space-time dimensions ...
We define a regularity axiom for lattice-valued convergence spaces where the lattice is a complete Heyting algebra. To this end, we generalize the characterization of regularity by a "dual form" of a diagonal condition. ... In the classical theory of convergence spaces, there is a stronger axiom, (F) (named after Fischer, first published in ...
The theory of convergence structures in [BB02] delivers a promising foundation on which to study general notions of convergence. However, that theory has one striking feature that ... the working mathematician and provide a new angle for studying aspects of vector lattice theory. We demonstrate the value of this approach using order convergence ...
In this paper we continue the study started in [3, 10, 11, 16,17,18,19] were we have extended to the vector lattice setting the problem of approximating a function f by means of Urysohn-type integral operators in the setting of modular convergence.These operators are particularly useful in order to approximate a continuous or analog signal by means of discrete samples, and therefore they are ...
Lattice gauge theory; Heuristics and convergence. Part of the book series: Lecture Notes in Mathematics ( (LNM,volume 1158)) This research was supported in part by N.S.F. Grant MCS 81-02147, in part by the Institute for Mathematics and its Applications at the University of Minnesota and in part by the Institute for Advanced Study in Princeton ...
Convergence of the ί/(l) 4 Lattice Gauge Theory to Its Continuum Limit Bruce K. Driver Institute for Advanced Study, School of Mathematics, E.C.P. Building, Princeton, NJ 08540, USA Abstract. It is shown that in four space-time dimensions the compact U(l) lattice gauge theory with general energy function converges to a renormalized
general background on lattice gauge theory and for a survey of some deep results on convergence of noncompact lattice models by Balaban, Brydges, Frδhlich and Seiler, see [18]. 2. Notation and Statement of Results We consider lattice gauge models determined by a real-valued energy function h on the real line R, satisfying some of the following ...
Moore-Smith convergence theory is enriched in a fuzzy lattice.The σ-limit point and σ-cluster point of a molecular net and a filter in a fuzzy lattice are defined and their various properties are discussed, and the σ-closure, the σ-interior operators and σ-topological molecular lattices are introduced by the concept of ordered pair of R-neighborhoods.
xiii. January 4, 2012 12:34 Lattice Gauge Theories: An Introduction (4th Edition) 11in x 8in b1271-fm xivLattice Gauge Theories. The book is divided in two parts. In the ・〉st part, comprising chapters 1 to 16, I discuss the zero temperature formulation of ・'ld theories on a space-time lattice, and in particular QCD.
The main purpose of this paper is to apply the theory of vector lattices and the related abstract modular convergence to the context of Mellin-type kernels and (non)linear ... abstract convergence in the vector lattice context (see, e.g., [14, Definition 2.1] and [3]).
By unified method we prove the convergence of the lattice approximation of the (λφ 4 −σφ 2 −ψφ) 3 field model with periodic, Dirichlet and Neumann boundary conditions in a finite box. This then allows us to take the inifinite volume limit of the Dirichlet states by the Nelson's monotonicity argument.
Lattice parameters were optimized by a fitting procedure using the Birch-Murnaghan equation of state 78,79 The final structures used in all subsequent calculations were obtained by relaxing the ...
A numerical convergence theory for nonlinear convective-diffusive lattice Boltzmann methods is established. Convergence, consistency, and stability are defined through truncated Hilbert expansions. In this setting it is shown that consistency and stability imply convergence. Monotone lattice Boltzmann methods are defined and shown to be ...
In such cases, gauge symmetry-breaking regulariza-tions become necessary. In this paper, we argue that a broken lattice gauge symmetry is ac-ceptable as long as gauge symmetry is restoredin the continuum limit. We propose a method to construct the continuum limit for a class of lattice-regularized Hamiltonian field theories, where the ...
Moore-Smith convergence theory is enriched in a fuzzy lattice. The σ-limit point and σ-cluster point of a molecular net and a filter in a fuzzy lattice are defined and their various properties are discussed, and the σ-closure, the σ-interior operators and σ-topological molecular lattices are introduced by the concept of ordered pair of <i>R</i>-neighborhoods.
It is shown that in three space-time dimensions the pure U(1) lattice gauge theory with Villain action and fixed coupling constant converges to the free electromagnetic field as the lattice spacing approaches zero. The same holds for the Wilson action on the electric sector.
In this paper, an ω-convergence theory of molecular nets in an ω-molecular lattice is established. By means of the ω-convergence theory, some important characterizations with respective to the ω-closed sets, ωT 2 separation and (ω 1 , ω 2 )-continuous mappings are obtained.
3. The convergence of the calculations Before starting the actual calculation for the lattice constant, it is important to explore the convergence of the calculations with respect to the number of k points and the energy cutoff. So the most accurate and efficient results can be obtained.
Abstract. In this article we prove convergence of Lattice Gauge Theory in the energy norm for electromagnetism, which corresponds to gauge group U (1). This is done by stability analysis and comparison with the classical Yee-scheme which is convergent.
The approximation theory of RBFNN showed that the smaller distance of lattice points can make the better approximation performance in the learning process . However, in Deterministic Learning theory, Zheng [ 17 ] found that choosing distance of lattice points in fixed a value can result in the best approximation and the PE condition.