Genetic Diversity: Sources, Threats, and Conservation
- Reference work entry
- First Online: 25 November 2020
- Cite this reference work entry
- Marina Nonić 6 &
- Mirjana Šijačić-Nikolić 6
Part of the book series: Encyclopedia of the UN Sustainable Development Goals ((ENUNSDG))
811 Accesses
4 Citations
Diversity of genes ; Genetic differences ; Genetic variation ; Genetic variety
Genetic diversity is a fundamental source of biodiversity which has been defined by different authors as “any measure that quantifies the magnitude of genetic variability within a population” (Hughes et al. 2008 ) or “the very makeup of the variation of organisms and species on Earth” (Elliott 2002 ). According to Ennos et al. ( 2000 ), genetic diversity presents “the range and sum of genetic variation within a population or populations,” where the term diversity , which simply means the state of displaying dissimilarities, differences, or variety, has acquired an extended meaning which signifies the sum of the variation .
Each individual species is made up of individuals that possess their genes, which are the source of its own unique features (genes are responsible for both the similarities and the differences between organisms). A species may have different populations, each having different...
This is a preview of subscription content, log in via an institution to check access.

Access this chapter
- Get 10 units per month
- Download Article/Chapter or Ebook
- 1 Unit = 1 Article or 1 Chapter
- Cancel anytime
- Available as PDF
- Read on any device
- Instant download
- Own it forever
- Available as EPUB and PDF
- Durable hardcover edition
- Dispatched in 3 to 5 business days
- Free shipping worldwide - see info
Tax calculation will be finalised at checkout
Purchases are for personal use only
Institutional subscriptions
Abdel-Mawgood AL (2012) DNA based techniques for studying genetic diversity. In: Caliskan M (ed) Genetic diversity in microorganisms. InTech, Rijeka, pp 95–123
Google Scholar
Avise JC (2004) Molecular markers, natural history, and evolution, 2nd edn. Sinauer Associates, Sunderland
Avise JC, Hamrick JL (1996) Conservation genetics: case histories from nature. Chapman and Hall, New York
Book Google Scholar
Baye TM, Abebe T, Wilke RA (2011) Genotype-environment interactions and their translational implications. Per Med 8(1):59–70
Article Google Scholar
Beatty CR, Cox NA, Kuzee ME (2018) Biodiversity guidelines for forest landscape restoration opportunities assessments, 1st edn. IUCN, Gland. v + 43pp
Bošković J, Isajev V (2007) Genetika (Genetics). Megatrend University, Belgrade, 552 pages
Brook BW, Tonkyn DW, O’Grady JJ, Frankham R (2002) Contribution of inbreeding to extinction risk in threatened species. Conserv Ecol 6(1):16. http://www.consecol.org/vol6/iss1/art16
Caliskan M (2012).Genetic diversity in microorganisms. InTech, Rijeka. https://doi.org/10.5772/2641 . ISBN: 978-953-51-0064-5
Candolin U, Heuschele J (2008) Is sexual selection beneficial during adaptation to environmental change? Trends Ecol Evol 23(8):446–452
Charlesworth B (2001) The effect of life-history and mode of inheritance on neutral genetic variability. Genet Res 77:153–166
Article CAS Google Scholar
Charlesworth B (2009) Effective population size and patterns of molecular evolution and variation. Nat Rev Genet 10:195–205
Corl A, Ellegren H (2012) The genomic signature of sexual selection in the genetic diversity of the sex chromosomes and autosomes. Evolution 66(7):2138–2149
Dražić G (2015) Biological resources in the service of ecoremediation. In: Milovanović J, Djordjević S (eds) Conservation and enhancement of biological resources in the service of ecoremediation. Faculty of applied ecology Futura, Singidunum University, Belgrade, pp 13–28. ISBN: 978-86-86859-41-9
Elliott LJ (2002) The effects of the decline of genetic biodiversity on the prosperity and well-being of mankind. In: Genetics in human affairs. https://projects.ncsu.edu/cals/course/gn301/GeneticBiodiversity.html
Ennos RA, Worrell R, Arkle P, Malcolm DC (2000) Genetic diversity and conservation. In: Genetic variation and conservation of British native trees and shrubs current knowledge and policy implications. Forestry commission technical paper 31. Forestry Commission, Edinburgh
Eriksson G, Ekberg I (2001) An introduction to forest genetics. Swedish University of Agricultural Sciences, Uppsala, pp 1–166
Fjeldsa J, Lovett J (1997) Biodiversity and environmental stability. Biodivers Conserv 6:315–323
Freeman WH (2000) Sources of variation. In: Griffiths AJF, Miller JH, Suzuki DT, Lewontin RC, Gelbart WM (eds) An introduction to genetic analysis, 7th edn. W.H. Freeman, New York. ISBN: 10: 0-7167-3520-2. https://www.ncbi.nlm.nih.gov/books/NBK22012/
Galov A (2007) Genetic diversity of bottlenose dolphin ( Tursiops truncatus ) and notes on genetic diversity of other cetacean species in the Adriatic Sea. Doctoral thesis, University of Zagreb-Faculty of Science Department of Biology, 105 pages
Gardner EJ (1964) Principles of genetics, 2nd edn. Wiley, New York, 342 pages
GD (2018) Genetic diversity. http://www.biodiversity.ru/coastlearn/bio-eng/boxes/geneticdiv.html
Gilpin ME, Soule ME (1986) Minimum viable populations: processes of species extinction. In: Soule ME (ed) Conservation biology: the science of scarcity and diversity. Sinauer Associates, Sunderland
Govindaraj M, Vetriventhan M, Srinivasan M (2015) Importance of genetic diversity assessment in crop plants and its recent advances: an overview of its analytical perspectives. Genet Res Int 2015, 1–14, Article ID 431487. https://doi.org/10.1155/2015/431487 . Hindawi Publishing Corporation
Hamrick JL, Godt MJW (1996) Conservation genetics of endemic plant species. In: Avise JC, Hamrick JL (eds) Conservation genetics: case histories from nature. Chapman and Hall, New York, pp 281–304
Chapter Google Scholar
Hartl DL, Ruvolo M (2012) Genetics: analysis of genetics and genomes. Jones & Bartlett, Burlington, 804 pages
Hattemer HH (1991) Measuring genetic variation. In: Müller-Starck G, Ziehe M (eds) Genetic variation in European populations of forest trees. Sauerlander’s Verlag, Frankfurt am Main, pp 2–20
Hughes RA, Brian D, Inouye BD, Marc TJJ, Underwood N, Vellend M (2008) Ecological consequences of genetic diversity. Ecol Lett 11:609–623
IPGRI and CU – International Plant Genetic Resources Institute and Cornell University (2003) Genetic diversity analysis with molecular marker data: learning module. Measures of Genetic Diversity, IPGRI/Cornell University, Maccarese/Ithaca
Isajev V, Šijačić-Nikolić M (2003) Praktikum iz genetike sa oplemenjivanjem biljaka (Auxiliary University textbook: “Practicum in genetics with plant breeding”). Faculty of Forestry-University of Belgrade, Belgrade-Serbia and Faculty of Forestry-University of Banja Luka, Banja Luka-Bosnia and Herzegovina, pp 1–240
Kirkpatrick M, Ryan MJ (1991) The evolution of mating preferences and the paradox of the lek. Nature 350:33–38
Levin SA (2001) Encyclopedia of biodiversity. Academic, San Diego
Maehr DS, Crowley P, Cox JJ, Lacki MJ, Larkin JL, Hoctor TS, Harris LD, Hall PM (2006) Of cats and haruspices: genetic intervention in the Florida panther. Anim Conserv 9:127–132. https://doi.org/10.1111/j.1469-1795.2005.00019.x . Response to Pimm et al
Markert JA, Champlin DM, Gutjahr-Gobell R, Grear JS, Kuhn A, McGreevy TJ, Roth A, Bagley MJ, Nacci DE (2010) Population genetic diversity and fitness in multiple environments. BMC Evol Biol 10:205
Milligan BG, Leebens-Mack J, Strand AE (1994) Conservation genetics: beyond the maintenance of marker diversity. Mol Ecol 12:844–855
Morić M (2016) Genetic diversity of pedunculated oak ( Quercus robur L.) in field trials whit progeny from selected seeds stands. Doctoral thesis, University of Zagreb – Faculty of Forestry, pp 14–19
Nonić M (2016) Improving mass production of leaf-ornamental beech cultivars by grafting. Doctoral thesis, University of Belgrade – Faculty of Forestry, 280 pages
NRC – National Research Council (1993) Livestock. The National Academies Press, Washington, DC. https://doi.org/10.17226/1584
Nunney L (1993) The influence of mating system and overlapping generations on effective population-size. Evolution 47:1329–1341
Oliver TH (2018) Biodiversity generation and loss. Environ Sci. https://doi.org/10.1093/acrefore/9780199389414.013.96 . http://environmentalscience.oxfordre.com/view/10.1093/acrefore/9780199389414.001.0001/acrefore-9780199389414-e-96
Panhuis TM, Butlin R, Zuk M, Tregenza T (2001) Sexual selection and speciation. Trends Ecol Evol 16(7):364–372
Pérez-González J, Costa V, Santos P, Slate J, Carranza J, Fernández-Llario P, Zsolnia A, Monteiro NM, Anton I, Buzgo J, Varga G, Beja-Pereira A (2014) Males and females contribute unequally to offspring genetic diversity in the polygynandrous mating system of wild boar. PLoS One 9(12):e115394
Primack RB, Milić D, Radenković S, Obreht D, Bjelić-Čabrilo O, Vujić A (2015) An introduction to conservation biology (Uvod u konzervacionu biologiju). Faculty of Natural Sciences and Mathematics, Novi Sad, 372 pages
Schaal BA, Leverich WJ, Rogstad SH (1991) Comparison of methods for assessing genetic variation in plant conservation biology. In: Falk DA, Holsinger KE (eds) Genetics and conservation of rare plants. Oxford University Press, New York, pp 123–134
Shanshan L, Chiang TY, Gong X (2006) High genetic diversity vs. low genetic differentiation in Nouelia insignis (Asteraceae), a narrowly distributed and endemic species in China, revealed by ISSR fingerprinting. Ann Bot 98:583–589. https://doi.org/10.1093/aob/mcl129
Šijačić-Nikolić M, Milovanović J (2010) Conservation and directed utilization of forest genetic resources. Faculty of Forestry – University of Belgrade, Planeta Print, Belgrade, pp 1–200
Šijačić-Nikolić M, Milovanović J, Nonić M (2014) Conservation of forest genetic resources. In: Ahuja MR, Ramawat KG (eds) Biotechnology and biodiversity. Series: Sustainable development and biodiversity, vol 4. Springer International Publishing Switzerland, pp 103–129
Todd PM, Miller GF (1997) Biodiversity through sexual selection. In: Langton CG, Shimohara K (eds) Artificial life V – proceedings of the fifth international workshop on the synthesis and simulation of living systems. MIT Press/Bradford Books, Cambridge, MA, pp 289–299
Tucović A (1990) Genetika sa oplemenjivanjem biljaka (Genetics and plant breeding). Naučna knjiga, Beograd (Scientific Book – Belgrade), pp 1–596
Westemeier R, Brawn J, Simpson S, Esker T, Jansen R, Walk J, Kershner E, Bouzat J, Paige K (1998) Tracking the long-term decline and recovery of an isolated population. Science 282:1695–1698. https://doi.org/10.1126/science.282.5394.1695
White TL, Adams WT, Neale DB (2007) Forest genetics. CAB International, Wallingford, 682 pages
Download references
Author information
Authors and affiliations.
Faculty of Forestry, University of Belgrade, Belgrade, Serbia
Marina Nonić & Mirjana Šijačić-Nikolić
You can also search for this author in PubMed Google Scholar
Corresponding author
Correspondence to Marina Nonić .
Editor information
Editors and affiliations.
European School of Sustainability Science and Research, Hamburg University of Applied Sciences, Hamburg, Germany
Walter Leal Filho
Center for Neuroscience and Cell Biology, Institute for Interdisciplinary Research, University of Coimbra, Coimbra, Portugal
Anabela Marisa Azul
Faculty of Engineering and Architecture, Passo Fundo University, Passo Fundo, Brazil
Luciana Brandli
HAW Hamburg, Hamburg, Germany
Amanda Lange Salvia
University of Chester, Chester, UK
Section Editor information
No affiliation provided
Adriana Consorte McCrea
Rights and permissions
Reprints and permissions
Copyright information
© 2021 Springer Nature Switzerland AG
About this entry
Cite this entry.
Nonić, M., Šijačić-Nikolić, M. (2021). Genetic Diversity: Sources, Threats, and Conservation. In: Leal Filho, W., Azul, A.M., Brandli, L., Lange Salvia, A., Wall, T. (eds) Life on Land. Encyclopedia of the UN Sustainable Development Goals. Springer, Cham. https://doi.org/10.1007/978-3-319-95981-8_53
Download citation
DOI : https://doi.org/10.1007/978-3-319-95981-8_53
Published : 25 November 2020
Publisher Name : Springer, Cham
Print ISBN : 978-3-319-95980-1
Online ISBN : 978-3-319-95981-8
eBook Packages : Earth and Environmental Science Reference Module Physical and Materials Science Reference Module Earth and Environmental Sciences
Share this entry
Anyone you share the following link with will be able to read this content:
Sorry, a shareable link is not currently available for this article.
Provided by the Springer Nature SharedIt content-sharing initiative
- Publish with us
Policies and ethics
- Find a journal
- Track your research

An official website of the United States government
The .gov means it’s official. Federal government websites often end in .gov or .mil. Before sharing sensitive information, make sure you’re on a federal government site.
The site is secure. The https:// ensures that you are connecting to the official website and that any information you provide is encrypted and transmitted securely.
- Publications
- Account settings
Preview improvements coming to the PMC website in October 2024. Learn More or Try it out now .
- Advanced Search
- Journal List
- Genes (Basel)

Genetic Diversity, Conservation, and Utilization of Plant Genetic Resources
Romesh kumar salgotra.
1 School of Biotechnology, Sher-e-Kashmir University of Agricultural Sciences and Technology of Jammu, Chatha, Jammu 180009, India
Bhagirath Singh Chauhan
2 Queensland Alliance for Agriculture and Food Innovation (QAAFI), The University of Queensland, Gatton, QLD 4343, Australia
Associated Data
No new data were created or analyzed in this study. Data sharing is not applicable to this article.
Plant genetic resources (PGRs) are the total hereditary material, which includes all the alleles of various genes, present in a crop species and its wild relatives. They are a major resource that humans depend on to increase farming resilience and profit. Hence, the demand for genetic resources will increase as the world population increases. There is a need to conserve and maintain the genetic diversity of these valuable resources for sustainable food security. Due to environmental changes and genetic erosion, some valuable genetic resources have already become extinct. The landraces, wild relatives, wild species, genetic stock, advanced breeding material, and modern varieties are some of the important plant genetic resources. These diverse resources have contributed to maintaining sustainable biodiversity. New crop varieties with desirable traits have been developed using these resources. Novel genes/alleles linked to the trait of interest are transferred into the commercially cultivated varieties using biotechnological tools. Diversity should be maintained as a genetic resource for the sustainable development of new crop varieties. Additionally, advances in biotechnological tools, such as next-generation sequencing, molecular markers, in vitro culture technology, cryopreservation, and gene banks, help in the precise characterization and conservation of rare and endangered species. Genomic tools help in the identification of quantitative trait loci (QTLs) and novel genes in plants that can be transferred through marker-assisted selection and marker-assisted backcrossing breeding approaches. This article focuses on the recent development in maintaining the diversity of genetic resources, their conservation, and their sustainable utilization to secure global food security.
1. Introduction
Genetic diversity is the amount of genetic variability present among individuals of a variety or a population within a species. It is the product of the recombination of genetic material (DNA) during the inheritance process, mutations, gene flow, and genetic drift [ 1 ], and it results in variations in DNA sequence, epigenetic profiles, protein structure or isoenzymes, physiological properties, and morphological properties. The diversity among plant and animal populations is determined by the hereditary material present in the reproducing members of the population. Genetic diversity is the main driving force for the selection and evolution of populations. Within crop species, the selection of individuals can be natural or artificial, depending upon the variation present [ 2 ]. Genetic diversity can be distilled down to the alleles of a gene present in the population, their effects, and their distribution. Genetic diversity is crucial for a healthy population as it maintains different genes that could lead to resistance to pests, diseases, or other stress conditions. It also enables individuals to adapt to various biotic and abiotic stresses. Under environmental changes, different crop varieties survive due to the presence of genetic variation, which enables the varieties to adapt. However, the varieties with little or no genetic diversity could become susceptible to biotic and abiotic stresses. Genetic diversity helps breeders to maintain the crossbred varieties, which leads to sustaining the desirable traits of the varieties, such as quality characteristics and tolerance to various stresses.
In general, plant genetic resources (PGRs) are the total hereditary material, which includes all the alleles of various genes, present in a crop species, including horticulture and medicinal plants, and their wild relatives. They can also be defined as any type of reproductive or vegetative propagating material of the plant species. PGRs include newly developed varieties, cultivated crop varieties, landraces, modern cultivars, obsolete cultivars, breeding stocks, wild forms, weedy forms, wild species of cultivated crops, and genetic stocks, including current breeders’ lines, elite lines, and mutants. These are the building blocks for the genetic improvement of agricultural and industrial crops [ 3 ]. Most of the agro-industry and agro-processing sectors also rely on PGRs. These are the pillars of crop development programs, and world food security depends upon the extent of genetic diversity present in PGRs [ 4 ]. PGRs are used in crop improvement programs, particularly in the varietal developmental programs. These resources are also used in systematic studies, such as evolutionary biology, cytogenetic, biochemical, physiology, phylogenetic, ecological research, pathology, molecular studies, etc. PGRs encompass all cultivated, wild relatives of cultivated species, traditional cultivars, landraces, and advanced breeding lines of plants [ 5 ]. The demand for these PGRs will increase in the future to feed the ever-increasing global population. Moreover, the precedential increase in the world population has resulted in the over-exploitation of PGRs, which has led to the genetic erosion of important germplasm from habitats. Food security issues are of global significance, and genetic resources are being lost at alarming rates due to anthropogenic effects such as genetic erosion, over-exploitation of PGRd, population growth, and climate change. Moreover, with the development and introduction of high-yielding crop varieties, genetic diversity among plant genotypes is declining [ 6 ]. The situation is further exacerbated by the frequent recurrence of biotic and abiotic stresses, resulting in a huge loss of PGRs. To avoid this catastrophic situation, there is a need to protect these valuable resources from genetic erosion and use them judiciously. To meet the current, as well as future, global challenges, PGRs need to be explored, collected, conserved, and utilized sustainably. Additionally, the survey, exploration, collection, preservation, and sustainable utilization of PGRs in an organized way is the responsibility of all nations. Researchers, policymakers, and planners have already begun to plan for the proper conservation and sustainable utilization of PGRs for the benefit of society [ 7 ].
Maintaining diversity in PGRs is vital for the development and genetic improvement of crop varieties. Presently, the plant species extinction rate is skyrocketing, and life on Earth is facing a sixth mass extinction event caused by climate change and anthropogenic activities, which may lead to ecological collapse [ 8 ]. The Leipzig Declaration [ 9 ] emphasized saving the seed and planting material to avoid genetic vulnerability and shortage of food under adverse conditions. The diverse gene pool of plant species, such as wild species, landraces, breeding stock, etc., could hold the tools for survival and adaptation under adverse climatic conditions [ 10 ]. The conservation and sustainable utilization of these valuable resources is crucial to ensure food security for future generations. Genetic resources should be easily available to plant breeders for the continuous development of new crop varieties. PGRs are an important reservoir of disease- and insect/pest-resistant genes, through which improved and immune crop varieties can be developed. In the present scenario of climate change, PGRs have played a significant role in the development of climate-resilient crop varieties to strengthen food security [ 11 ]. By using PGRs, crop varieties are being developed with better yield and quality traits along with resistance to biotic and abiotic stresses, such as diseases, insect pests, flooding, salinity, and drought. Additionally, developing countries rely on PGRs to create more diverse crops. The need for PGRs has risen continually for developing varieties of different crops such as cereals, pulses, vegetables, fruits, and ornamentals [ 11 ].
Today, the conservation and sustainable use of PGRs is a priority of the global community to solve issues surrounding food security and other problems arising from increased population growth. In the future, these resources will completely vanish if proper and stringent PGR conservation practices and policies are not implemented [ 11 ]. This challenge can be overcome by bringing all stakeholders, including farmers, ethnobotanists, indigenous knowledge-holding people, plant breeders, NGOs, seed banks, and policymakers together to share information, create PGR diversity awareness, develop new technologies, and deploy systematic and scientific conservation. Biotechnological techniques, such as cryopreservation, molecular markers, high-throughput sequencing, and genetic engineering, have improved the conservation of endangered and rare PGRs. Priority should also be given to the exploration of local germplasm and underutilized crop species and the maximum utilization of traditionally local landraces, with the involvement of local people. The Convention on Biological Diversity (CBD) and international undertaking on PGRs [ 12 ] are working in harmony for the conservation and sustainable utilization of PGRs under the umbrella of the Earth Summit of the United Nations Conference on Environment and Development (UNCED). A well-planned strategic and forward-looking vision is required for the conservation and sustainable utilization of these genetic resources.
2. Importance of Genetic Diversity in Plant Genetic Resources
Genetic diversity is the genetic base for crop improvement [ 13 ]. Diverse PGRs enable plant breeders to develop or improve crop varieties with desirable qualities. While developing new cultivars, due consideration must be given to the farmers’ preferences, such as high-yielding varieties, quality, and resistance to diseases and insect pests. In ancient times, humans selected desirable genotypes based on natural genetic variability in the population [ 14 ]. The preference for the development of new crop varieties shifts over a period with environmental changes. Plant breeders develop climate-resilient varieties possessing all the desirable traits, including resistance to various biotic and abiotic stresses. Genetic diversity in the form of mutant lines, wild species, breeding stocks, etc., is used for the improvement and development of modern crop varieties [ 13 ]. For the development of climate-resilient varieties, novel genes tolerant to biotic and abiotic stresses need to be conserved for future use in breeding programs. Additionally, the plant genotypes possessing genes for quality traits and aesthetic properties should be preserved in the available germplasm. Genetic diversity within and between plant species allows plant breeders to select superior genotypes, which can then be used for the development of genetic stock for hybridization programs or the release of a crop variety [ 13 ]. Genetic diversity enables PGRs to adapt to varied climatic conditions [ 14 , 15 ]. Moreover, the negative impact of inbreeding in populations can be reduced by enhancing genetic diversity. Higher levels of genetic diversity in PGRs support resilience to adverse environmental changes, integrity, community structure, and ecosystem functions [ 16 , 17 ]. Additionally, it helps plant breeders to utilize genetically diverse parents in a breeding program to improve the productivity of varieties of agriculture and horticulture crops [ 18 ] ( Figure 1 ).

Different sources of genetic diversity and their potential utilization in the development of new crop varieties.
Genetic diversity in plant species depends on the heritable variation present within and between populations. It occurs due to genetic variation in the nucleotide sequence of DNA, chromosome mutations, and recombination during sexual reproduction [ 19 ]. In the sexual reproduction of plant species, the F 1 and advanced generations are developed by crossing two or more diverse parents. The offspring developed from two genetically diverse parents possess genetic variations because of recombination during meiosis. Hence, genetically dissimilar offspring from parents are produced. However, this is the genetic material of individuals underlying the variability within, as well as between, species [ 20 ]. Generally, genetic diversity can be observed at three levels: diversity between species, diversity between populations within one species, and diversity between individuals within one population. It is genetic variability that provides evolutionary flexibility, resilience, and adaptability in plant species [ 21 ]. Before the identification of diverse parents in plant breeding programs, breeders and biotechnologists used a multitude of techniques for the characterization of the germplasm to know the genetic diversity [ 22 ]. For the characterization of the genetic diversity of PGRs and the identification of superior genotypes, various techniques are used, such as phenotypic or morphological traits, biochemical or allozyme techniques, and molecular techniques.
3. Factors Affecting Genetic Diversity
Genetic diversity changes over time owing to several factors. The main factors responsible for changes in genetic diversity are mutation, selection, genetic drift, and gene flow. Over time, natural and artificial selections play a substantial role in the choosing of superior genotypes, which significantly affects the gene and genotypic frequencies of the population [ 23 ]. As per Charles Darwin’s theory of evolution (1859), the desired genotypes are selected for and passed onto subsequent generations [ 24 , 25 , 26 ]. However, the domestication of desirable genotypes results from the superior genotypes being selected by farmers and breeders and neglects other undesirable genotypes. This leads to a reduction in inferior alleles over generations. During evolution, various morphological, physiological, and biochemical changes take place in plant species and can take different directions under domestication depending on the part of the plant used. Some plant species lose their sexual reproduction during selection for large size of the tuber or root, which is associated with selection for polyploid types, resulting in sterility. Some polyploid plant species, such as allohexaploid wheat and potato, show diploidization behavior during sexual reproduction. Some crops have been turned into annuals from their original form of perennials. In the domestication process, the complete genetic transformation of wild species occurs in the development of modern cultivars through natural and artificial selection [ 23 ]. After some time, some domesticated cultivars become susceptible to diseases and pests, which can be improved by incorporating genes from wild plant relatives [ 27 ]. During the process of domestication, desirable traits have been selected by breeders as per their preferences [ 27 , 28 ]. However, plant breeders prefer to choose crop varieties with a high yield, resistance to biotic and abiotic stresses, wide adaptation, non-shattering nature, large-sized seeds, early maturing, good quality traits, etc. [ 29 , 30 ]. The main factors affecting genetic diversity will be addressed in the following subsections.
3.1. Mutation
Mutations are sudden heritable changes that occur due to aberrations in the nucleotide sequence of DNA. A mutation is the source of genetic variation impacting the phenotype in crop species. Genetic diversity caused by mutations can have neutral, positive, and negative impacts on various characteristics of a plant species. Genetic variations caused by mutations in DNA are the principal cause of changes in the allele frequencies in a population besides selection and genetic drift. From the beginning, natural or spontaneous mutations have played a significant role in creating the genetic variation that has led to food security [ 31 ]. Mutations are the ultimate source of plant evolution when they frequently encounter environmental changes. Mutation rates proceed rapidly in response to environmental changes or even changes in the demographical locations related to the socio-economic conditions of the human population in a geographical area. Stress-inducible mutagenesis has been observed because of the use of different external inputs which accelerate adaptive evolution in plants. During mutagenesis, many kinds of genetic changes have been observed such as insertions, deletions, copy number variations, gross chromosomal rearrangements, and the movement of mobile elements. Earlier plant breeders utilized natural mutations as the main source of genetic variation for improving and developing crop varieties. However, modern technologies have accelerated the process by inducing mutation through mutagenesis The concept of mutation breeding was introduced to create more genetic diversity among crop species to improve traits such as disease and insect pest resistance, tolerance to abiotic stresses, and nutritional enhancement in crop varieties [ 32 ].
3.2. Selection
Natural and artificial selections act on the phenotypic characteristics of the plant species. The phenotypic expression of the plant species depends upon the heritable and non-heritable components in which the genotype–environment interaction also plays a significant role. The selection of superior genotypes depends on the availability of genetic variation present in the plant species. Artificial selection is effective only when sufficient genetic variation is present in the population. The genetic improvement of a genotype depends on the magnitude of genetic variability present in the population, as well as the nature of the association between different components. For example, the level of association of yield traits with other characteristics of the plant species enables the selection of various traits at a time [ 33 ]. Plant breeders make effective selection depending on the presence of substantial genetic variation in the population to enhance the maximum genetic yield potential of crop varieties [ 34 ]. It also helps in selecting better parents to be used in hybridization programs. Hence, the effective selection of genotypes in a population also depends on the degree of genetic variation in the population.
3.3. Migration
Migration is the movement of alleles from one species to another or from one population to another. It occurs through the movement of pollen and seed dispersal and planting material such as rhizomes, suckers, and other vegetative propagating materials. The rate of migration is affected by reproduction cycles and the dispersion of seeds and pollens. Migration can also occur through the moving or shifting of the germplasm from one area to another, which results in the mixing of two or more alleles through pollen and seeds [ 35 ].
3.4. Genetic Drift
Genetic drift is a mechanism in which the gene and allele frequencies of a population change due to sampling errors over generations. The sampling error changes the allele frequencies by chance, which ultimately changes the genetic diversity over generations. Every pollen grain has a different combination of alleles and can be carried by insects, wind, humans, or other means for hybridization with compatible flowers, largely determined by chance. Thus, in every reproduction cycle, the genetic diversity in crop species is lost at every generation through these chance events [ 36 ].
4. Factors That Cause Genetic Vulnerability
Over the past century, it has been observed that the genetic diversity in wild populations is declining globally [ 16 , 37 ]. Genetically distinct populations for most species are also declining due to the shrinkage of geographic ranges and lack of proper management and conservation practices [ 38 , 39 , 40 ]. Most genetic diversity is lost due to infrastructure development, climate change, habitat fragmentation, population reduction, overgrazing, and overharvesting [ 41 ]. Besides this, the following subsections describe the major components responsible for the genetic vulnerability of genetic resources.
4.1. Narrow Genetic Base of Crop Varieties
The main reason for genetic erosion and vulnerability is the cultivation of genetically uniform cultivars with a narrow genetic base. Indigenous or traditional crop varieties have a broad genetic base, and these cultivars can tolerate various biotic and abiotic stresses [ 42 ]. Traditional crop varieties have a low genotype–environment interaction, enabling the genotypes to withstand an epidemic of disease, insect pest incidence, and other adverse environmental conditions [ 43 ]. Moreover, pathogen races are less prone to infesting traditional varieties because of the broad genetic base of these varieties compared to the modern released varieties that have common parents. Hybrids have been developed by crossing different genetically uniform inbred lines, which significantly decreased genetic diversity. Additionally, most high-yielding crop varieties have been developed by crossing common parents possessing similar genetic backgrounds, which can significantly reduce the genetic bases of the varieties [ 22 , 44 ].
4.2. Wide Spread of Dominant Varieties
The widespread cultivation of a single crop variety over a large area causes genetic vulnerability. These varieties may perform well for a short period but may become susceptible to several diseases and pests. Vertical resistance occurs due to the presence of oligogenic or monogenic resistance, and horizontal resistance occurs due to the presence of polygenes. Sometimes the vertical resistance present in the modern cultivar may show resistance against a disease, but will become susceptible if the pathogen evolves. In vertical resistance, the race of the pathogen or the insect pest biotype interacts with the host and overcomes the monogenic resistance present in the modern cultivar, and the variety becomes susceptible to a particular pathogen or biotype.
4.3. Unplanned Introduction of New Plant Species
Sometimes, new high-yielding plant species are introduced and used in a breeding program without proper screening for disease and insect pest resistance, which may result in an unpredicted epidemic of diseases. An example of this is the unplanned introduction of the Texas male sterile (TMS) genotype of maize for the development of hybrid maize genotypes in the USA in 1970. Newly developed maize hybrids had all the desirable characteristics and resistance to most of the common maize diseases. The TMS hybrids were widely cultivated in the USA, covering more than 90% of the maize area. However, these hybrids were susceptible to fungal strains and southern corn leaf blight ( Helminthosporium maydis ). The southern corn leaf blight disease colonized and spread widely, and the whole maize crop was wiped out. If the TMS, a source of male sterility, had been tested and screened properly before use in hybrid breeding programs, or if the monoculture of TMS hybrids had been avoided, the spread of this epidemic could have been countered [ 45 ].
5. Conservation of Plant Genetic Resources
Since the beginning of agriculture, for a time, the selection, cultivation, and conservation of seeds of locally acclimated plants, also known as called “landraces”, were practiced [ 46 ]. This process continued until the rediscovery of Gregor Mendel’s work in the 20th century. This work led to the introduction of breeding programs for the development of high-yielding and stress (biotic and abiotic)-tolerant crop varieties. In the middle of the last century, it laid the foundation for the “Green Revolution” and brought about an exponential increase in agricultural production. However, this led to the replacement of landraces and the expansion of the monoculture cropping system. Over 75% of the genetic diversity in PGRs and 90% of the crop varieties were lost and disappeared from farmers’ fields [ 47 ]. Now, it is of paramount importance that the remaining PGRs be conserved to sustain the agricultural production system in this era of climate change, global environmental problems, and booming population growth [ 48 ].
Since the 16th century, more than 80,000 plant species have been collected and preserved in about 3400 gardens across the world [ 49 ]. The main objective of this effort is to conserve the PGR diversity and wild species of crop plants to be used in breeding programs. In the mid-20th century, PGRs for food and agriculture (PGRFA) were preserved ex situ in specialized repositories, often termed gene banks. These gene banks are focused on inter-and intra-specific crop diversity. Presently, more than 17,000 regional, national, and international institutions are dealing with the conservation and sustainable use of PGRFA [ 49 ]. Additionally, 711 gene banks and 16 regional/international institutions/centers are spread over 90 countries, conserving more than 5.4 million accessions from over 7051 genera. The focus is to conserve the crop species, including crops’ wild relatives, landraces, modern cultivars, genetic stock, and breeding materials [ 50 ]. However, various international treaties have been implemented in harmony with the CBD for conservation, sustainable utilization, equity in benefit-sharing, and the safe handling of genetic resources.
5.1. International Treaty on Plant Genetic Resources for Food and Agriculture
The International Treaty on Plant Genetic Resources for Food and Agriculture (ITPGRFA) came into force in 2004. ITPGRFA works in harmony with the CBD for sustainable agriculture and food security. The objective of the treaty is the conservation and sustainable use of plant genetic resources for food and agriculture and the fair and equitable sharing of the benefits arising from their use. The conservation and sustainable use of PGRFA are essential to achieving sustainable agriculture and food security, for present and future generations, and are indispensable for crop genetic improvement in adapting to unpredictable environmental changes and human needs.
5.2. Nagoya Protocol
The Nagoya Protocol, which came into force in 2014, aims to access genetic resources and encourage the fair and equitable sharing of benefits arising from their utilization. The Nagoya Protocol helps in ensuring benefit-sharing, creates incentives to conserve and sustainably use genetic resources, and therefore, enhances the contribution of biodiversity to development and human well-being.
5.3. Svalbard Global Seed Vault
The Svalbard Global Seed Vault situated in Norway safeguards duplicate seed varieties from almost every country in the world. The Seed Vault is owned and run by the Ministry of Agriculture and Food on behalf of the Kingdom of Norway and is established as a service to the world community. The Global Crop Diversity Trust provides support for the ongoing operations of the Seed Vault, as well as funding for the preparation and shipment of seeds from developing countries to the facility. The Nordic Genetic Resource Center (NordGen) operates the facility and maintains a public online database of samples stored in the seed vault. It provides insurance against both incremental and catastrophic loss of crop diversity held in traditional genebanks around the world. The Seed Vault offers long-term protection for one of the most important natural resources on Earth. The main purpose is to backup genebank collections to secure the foundation of our future food supply.
5.4. The Cartagena Protocol on Biosafety
The Cartagena Protocol on Biosafety’s goal is to provide safety in the handling of genetic resources, particularly genetically modified organisms. It is an international agreement that aims to ensure the safe handling, transport, and use of living-modified organisms (LMOs) resulting from modern biotechnology that may have adverse effects on biological diversity, while also taking into account risks to human health.
The ever-increasing demand resulting from the explosive growth rate of the human population worldwide, and global warming, have forced world communities to think about the sustainable use of PGRs. The conservation of PGRs, including landraces, obsolete varieties, breeding material, wild species, and their wild relatives, is of utmost importance to secure future food security [ 44 ]. The vanishing of valuable genetic resources invoked the world’s communities to explore, collect, and preserve PGRs and maintain genetic diversity, as well as sign the CBD event in Rio de Janeiro in 1992. The importance of PGRs and biodiversity conservation was the main international issue discussed at the convention [ 44 ]. The CBD was organized with three main objectives: (i) the conservation of biodiversity, (ii) the sustainable use of its components, and (iii) the equitable sharing of benefits arising from the use of genetic resources. There is an urgent need to conserve genetic resources for the welfare of human beings and future food security, and to avoid the loss of valuable novel genes. Effective policies should be implemented to evade the extinction of valuable PGRs. There are various methods to conserve biodiversity, such as (i) in situ conservation, (ii) ex situ conservation, and (iii) biotechnological strategies/approaches ( Figure 2 ). The genetic diversity in PGRs, in situ or on farms/fields, is creating awareness in society at large about the importance of agrobiodiversity. In situ and ex situ conservation are complementary strategies to prevent the mass erosion of genetic resources. The utilization of crop genetic diversity is necessary for the development and release of new, well-adapted, and improved varieties for global food security.

Different strategies used for in situ and ex situ conservation of plant genetic resources.
5.5. In-Situ Conservation
In in situ conservation, genetic resources are conserved in their natural habitat, and the species are maintained in their original place. The plant species are conserved where they are found and are maintained in their original location [ 51 ]. In in situ conservation, the process of evolution is allowed to occur naturally with minimum interventions from humans. In this system, many wild plant species are conserved, especially forest and wild fruit crops. In situ conservation permits the plant species to evolve so that genetic diversity can be fostered. This process works via two methods: (i) farm/field conservation and (ii) genetic reserve conservation. Though both are concerned with the conservation and maintenance of diversity of genetic resources, on-farm conservation concerns traditional crop varieties or farming systems, while the latter deals with wild species in natural habitats [ 4 , 11 ]. In genetic reserve conservation, the area is defined by a location where genetic diversity has to be maintained through active and long-term conservation, such as a forest reserve area. In on-farm conservation, locally developed landraces are sustainably managed. Additionally, farmers conserve wild relatives and weedy forms within the existing farming system. Farmers select desirable plants for further cultivation; hence, a continuous process of evolution takes place. The in situ method of conservation allows the open pollination of different genotypes, and the resultant population of different genotypes possesses several alleles. However, to avoid natural calamities and the adverse effects of climate change, both in situ and ex situ conservation should be adopted complementarily [ 4 , 11 ].
5.6. Ex-Situ Conservation
Ex situ conservation is the conservation of different genetic resources outside their natural habitat. It involves the conservation of seed gene banks, plant tissue culture, cryopreservation, greenhouses, etc. It is the process of conserving endangered and overexploited genetic resources outside their natural habitat, which otherwise may experience habitat destruction and degradation, and every PGR may go extinct. Therefore, ex situ conservation is an alternate method of conserving valuable genetic resources [ 52 ]. In this method, PGRs are saved from extinction that would result from natural calamities, human interference, climate change, over-exploitation, and overutilization. The collected genetic resources should be well evaluated and characterized to avoid duplication, documented, and conserved under artificial conditions to be safe from external threats [ 4 ]. Among the various techniques of ex situ conservation, the seed storage technique is the most convenient and easiest for the long-term storage of seeds [ 4 , 11 ]. Orthodox seeds of food crops are used for storage as they can tolerate low temperatures and intense dehydration. In ex situ conservation, about 45% of the stored accessions are seed materials of cereal crops such as rice, wheat, maize, oat, triticale, rye, sorghum, and barley, followed by food legumes (15%), forages (9%), and vegetables (7%) [ 46 , 49 ].
Generally, the conservation of collected seeds is carried out in two ways: base collection and active collection. Base collection is the collection and maintenance of seed samples for long-term conservation. In this case, the seed samples are stored for the maximum time of seed viability at −18 to −20 °C [ 53 ]. In the base collection method, the moisture content of the seed to be stored should be between 3% and 7%, depending on the species. In the active collection method, the seed samples are stored for immediate use. Seed samples are stored for 10–20 years and should have at least 65% viability. In the active collection method, the moisture content varies from species to species, i.e., between 7% and 11% for seeds with good storability and between 3% and 8% for seeds with poor storability. It also depends on the temperature under which the seed samples are stored [ 54 ]. However, depending on the storage duration, these are categorized into three basic types: (i) long-term storage: when the seed samples are stored in facilities of base collection and are maintained at −18 to −20 °C; (ii) medium-term storage: when the period of storage is not more than 5 years, and seed samples are stored at a temperature between 0 °C and 10 °C with a relative humidity of 20–30%; and (iii) short-term storage: where the seed samples are stored for between 1 year and 18 months. For the latter, the temperature ranges between 20 °C and 22 °C, and the relative humidity should be 45–50%, where the seed can be stored for up to two years without losing its viability [ 54 ]. For long-term ex situ conservation, seed storage is the most low-cost and widely adopted storage method. It involves the desiccation of seeds and even storage in low-temperature conditions. However, the recalcitrant seeds and vegetatively propagated plant species do not survive under low temperatures like orthodox seeds. This method is significant for the conservation of forest and tree species. Even novel PGRs can be conserved in the home garden for future use in breeding programs. The ex situ conservation method enables the conservation of novel genes/alleles and ensures their sustainable use in crop improvement programs. The ex situ conservation of PGRs was started in the mid-20th century to slow the rapid loss of biodiversity with modern high-yielding crop varieties. The farmers replaced their traditional cultivars with improved ones [ 53 ]. This method is also helpful in the protection and conservation of wild relatives [ 55 , 56 ]. Ex situ conservation methods have been used for conserving important PGRs in several institutes [ 3 , 53 ] ( Table 1 ).
Important research institutes conserving and maintaining PGRs.
S. No. | International Research Institute | Mandate/Crops |
---|---|---|
1. | International Rice Research Institute (IRRI), Los Banos, Philippines | Rice |
2. | Centre International de-Mejoramients de Maize (CIMMYT), Trigo, El Baton, Mexico | Maize and wheat (triticale, barely, sorghum) |
3. | Center International de-agricultural Tropical (CIAT), Palmira, Columbia | Cassava and beans (also maize and rice), in collaboration with CIMMYT and IRRI |
4. | International Institute of Tropical Agriculture (IITA), Ibadan, Nigeria | Grain legumes, roots and tubers, farming systems, cassava, banana, yam |
5. | Centre International de la Papa (CIP), Lima. Peru | Potato, Andean root, and tubers |
6. | International Crops Research Institute, for Semi-Arid Tropics (ICRISAT), Hyderabad, India | Sorghum, groundnut, pearl millet, Bengal gram, red gram |
7. | West African Rice Development Association (WARDA), Monrovia, Liberia | Regional cooperative rice research in collaboration with IITA and IRRI |
8. | International Plant Genetic Research Institute (IPGRI), Rome Italy | Genetic conservation |
9. | National Bureau of Plant Genetic Resources, New Delhi, India | Fruits, tubers, medicinal and aromatic crops, spices, bulbous crops |
10. | The Asian Vegetable Research and Development Center (AVRDC), Taiwan | Tomato, onion, peppers, Chinese cabbage |
11. | International Center for Tropical Agriculture (CIAT) Columbia | Cassava |
12. | The New Zealand Institute for Plant and Food Research Limited, New Zealand | Kiwifruit ( spp.) |
13. | Svalbard Global Seed Vault, Norway | All crops from different countries |
5.7. Biotechnological Approaches
Plant biotechnology tools provide new opportunities for the conservation of genetic resources using various in vitro culture techniques. Various biotechnological tools, such as cell and tissue culture and other micropropagation techniques, have greatly contributed to the storage and transportation of PGRs [ 57 ]. Cell and tissue culture techniques are in use for the mass multiplication and production of PGRs in a short time for further conservation and transportation under aseptic conditions. Apart from this, next-generation sequencing (NGS), cell fusion techniques, recombinant technologies, proteomic structural biology, protein engineering, and genome editing techniques have opened new avenues and options for conserving genetic resources with increased precision. These technologies help in the conservation of rare and endangered species, ornamental species, forest species, medicinal species, and other vegetatively propagating plant materials [ 51 ]. When the biological material of PGRs (such as seeds or organs) cannot be propagated and stored using traditional methods, biotechnological tools, such as in vitro culture, cryopreservation, and molecular biology, can be used. Sometimes, reproductive barrier problems existing in some endangered and rare plant species can be solved via biotechnological interventions [ 58 ]. The following subsections highlight the main biotechnological techniques used for PGR conservation, which are not possible under normal storage systems. These techniques also help in the conservation of elite and pathogen-free plants in the short-, medium- and long-term.
5.7.1. In Vitro Propagation
In vitro gene banks are where PGRs are stored in an artificial nutrient medium. This is an alternative method to conserving the vegetative propagated plant genetic material [ 59 , 60 ]. The in vitro conservation method is well recognized by global agencies such as the International Board for Plant Genetic Resources (IBPGR) for safe transportation under regulated phytosanitary control. The main advantages of this technique are insect- and disease-free material, mass multiplication, no genetic erosion, reduced space and labor requirements, and less time taken to obtain a new plant. This technique helps to scale up the production of quality planting material throughout the year. In in vitro methods, the callus is produced from explants such as seeds, leaves, tubers, shoots, and nodes, from which a whole plant is regenerated. In in vitro techniques, an effective conservation method is required once cultures are established and the plant genetic material is multiplied sufficiently. This can be achieved by regularly subculturing the plants onto fresh media. However, there is a risk that subculturing may lead to microbial contamination and chances of somaclonal variations.
The successful production and propagation of genetically stable plants from cultures are prerequisites for in vitro conservation. Shoots are used for slow-growth storage to avoid somaclonal variations. This slow-growth storage is optimal for the medium-term conservation of PGRs [ 61 , 62 , 63 ]. In this method, the targeted germplasm is stored under plant tissue culture conditions and maintained on nutrient gels for 1 to 15 years with intermittent subculturing. Several techniques are optimized to slow the rate of growth, such as low-intensity light with lower temperatures or a reduced photoperiod. Sometimes, the slow growth of cold-tolerant plant species is maintained by employing a temperature range of 0–5 °C, and for tropical plant species, a temperature range of 15–20 °C. The use of growth retardants in culture media and cutting the supply of oxygen is carried out at different levels to slow down the growth of plantlets [ 64 ].
5.7.2. Cryopreservation
The cryopreservation technique involves the storage of biological plant tissue for conservation at ultra-low temperatures (−196 °C), mostly using liquid nitrogen. In cryopreservation, the plant species can be stored for a long period as all the activities, such as cellular metabolism and cell division in recalcitrant seeds and vegetatively propagated plant material, stop. In this approach, no sub-culturing is required, and the chances of somaclonal variations are also reduced [ 65 , 66 ]. The cryopreservation technique ensures cost-effective and safe long-term conservation of plant species; a wide range of plant species can be stored using this technique. In cryopreservation, a cryotherapy technique is also applied to eradicate systemic plant pathogens. In this technique, only meristem cultures or shoot apices are recommended because of their high rate of viability following an extended storage time and because they are virus-free plant materials [ 67 ]. In the cryopreservation technique, the first step is to remove all freezable water content from tissues using osmotic dehydration or a physical approach, followed by ultra-rapid freezing [ 68 ]. The freezable water content can be removed using freeze-induced dehydration and vitrification methods. In vitrification, crystalized ice formation is avoided, and the liquid phase is directly converted into an amorphous phase [ 69 ].
5.7.3. DNA Banks
Advances in molecular biology have made the conservation of endangered and rare species complementary. Genetic resource conservation through DNA is a cost-effective form of conserving PGRs. In biodiversity, many species are difficult to conserve and are at the stage of extinction. DNA storage may be one of the best alternatives to conserve the genetic diversity of these resources, which could possess novel genes/alleles that could aid in future food security. In a gene bank, genomic fragments consisting of individual genes or entire genotypes are conserved in a gene library or a library of DNA samples. Genetic information can be stored in the form of DNA, RNA, and cDNA. These libraries are the primary source of important germplasm for future scientific research worldwide. DNA conservation is an alternative method of conserving PGRs, where the genetic materials are difficult to conserve or threatened because of wild populations or climate change [ 70 ]. The genetic material, in the form of DNA, can be stored at −20 °C for up to 2 years for short- and mid-durations. However, for long-term storage, the genetic material can be stored at −70 °C with the help of liquid nitrogen. For the preservation of DNA, there are some DNA banks, such as the Australian Plant DNA Bank of Southern Cross University, the Royal Botanic Garden (UK), the Leslie Hill Molecular Systematics Laboratory, and the US Missouri Botanical Garden. Among these, The Royal Botanic Garden (UK) is the oldest and the most comprehensive DNA bank, encompassing more than 20,000 DNA samples of all plant families. Like other techniques of PGR conservation, DNA conservation can neither constitute the whole plant from conserved DNA nor recover the original genotypes. In these techniques, conserved DNA in the bank is first artificially introduced into the somatic cells, and then, the whole plant is regenerated using in vitro tissue culture techniques [ 51 ].
5.7.4. Digital Sequence Information
Digitized molecular data are vital to numerous aspects of scientific research and genetic resource use. Substantial advances in DNA sequencing over the last decades hold great potential to enhance food security and the sustainable use of global biodiversity, benefiting the world’s poorest people. Digital Sequence Information (DSI) plays a crucial role in catalyzing research applications that can contribute to international societal and biodiversity conservation targets. There are concerns over access to genetic resources and the absence of benefit sharing by provider countries. Open access to DSI might exacerbate this, which is leading to increasing policy interventions and restricted access to genetic resources and DSI. However, benefit sharing related to DSI is difficult to identify and hindered by the lack of clear international governance and legislation, which, in turn, has led to a reluctance to make DSI publicly and freely available.
6. Utilization of Plant Genetic Resources in Crop Improvement
Before modern cultivated crop varieties, landraces had more genetic diversity. Modern varieties are developed for specific traits, such as high yield, disease resistance, insect pest resistance, stress tolerance, and the improvement of nutritional characteristics. The plant breeders select diverse parents from PGRs in crossing programs to develop new crop varieties [ 71 ]. New crop varieties take at least 8–11 years to develop and may last for 5–6 years under cultivation. However, these varieties can be improved further by incorporating novel genes/alleles from wild relatives or wild species. The wild relatives and landraces are rich sources of novel genes resistant to biotic and abiotic stresses, and these are easily crossable with the cultivated crop varieties [ 72 ]. PGRs can be used in breeding programs in four ways: (i) the development of pre-breeding materials to be used in traditional breeding methods, (ii) the development of genetic stock as a source of resistance to various biotic and abiotic stresses and quality traits, (iii) the characterization and identification of PGRs for male sterility for the development of hybrids [ 73 ], and (iv) the development of modern cultivars by transferring the gene of interest from different genetic resources to popular crop varieties. PGRs are also used to increase genetic variation in the breeding population, incorporate genes to reduce the bottlenecks of the varieties, and develop hybrids, i.e., composites or synthetics.
Genomic Tools for Efficient Use of Plant Genetic Resources
With the advent of modern biotechnological techniques, the efficiency of plant breeders has significantly increased in the development and improvement of crop varieties. Next-generation sequencing (NGS), high-throughput sequencing (HTS), and high-throughput phenotypic (HTP) techniques enable more efficient use of PGRs. Among the various available techniques, DNA-based techniques are more reliable and widely used in crop improvement programs. Unlike other markers, molecular markers are not influenced by the environmental changes and developmental stages of plants [ 74 ]. Molecular markers—such as restriction fragment length polymorphisms (RFLPs), random amplified polymorphic DNA (RAPD), amplified fragment length polymorphisms (AFLPs), inter-simple sequence repeats (ISSRs), diversity array technology (DArT), simple sequence repeats (SSRs), single nucleotide polymorphisms (SNPs), etc.—are widely used in the molecular characterization of genetic diversity present within and between plant populations. Among the various molecular markers, SSR markers are widely used to characterize genotypes [ 22 , 75 ]. DNA-based markers are more efficient in the evaluation of the genetic diversity of endangered and rare species. The main advantages of molecular markers are that any small sample of plant material can be used for genetic diversity analysis. Molecular markers have been widely used to study genetic diversity, and different core collections of PGR accessions have been developed in crops such as rice [ 66 , 76 , 77 ], wheat [ 78 ], mungbean [ 79 ], soybean [ 80 ], common bean [ 81 , 82 ], pigeon pea [ 83 ], chilli [ 84 ], potato [ 85 ], carrot [ 86 ], tomato [ 87 ], oil palm [ 88 ], cotton [ 89 ], mulberry [ 90 ], barnyard [ 91 ], legume crops [ 92 ], and other vegetable and horticulture crops [ 93 ].
With the advances in high-throughput sequencing techniques, SNP markers are preferred for use in crop improvement programs. Various QTLs have been identified by developing a biparental population using PGR populations [ 94 , 95 , 96 ]. Besides the molecular characterization of the germplasm of PGRs for genetic diversity studies, molecular markers are widely used in plant breeding approaches, such as (i) the molecular marker-assisted testing of breeding materials for parental selection, assessing the level of genetic diversity, studying heterosis, the identification of genomic regions under selection, and the assessment of cultivar purity and cultivar identity [ 35 , 97 , 98 , 99 , 100 , 101 , 102 ]; (ii) marker-assisted recurrent selection (MARS) [ 103 ]; (iii) marker-assisted backcross breeding (MABB) [ 104 ]; and (iv) marker-assisted gene pyramiding [ 105 ] and genomic selection for complex traits [ 106 ]. Important crops have had biotic and abiotic resistance and quality traits introgressed through MAS and MABC/MABB approaches [ 66 , 75 , 92 ] ( Table 2 ).
Improvement of various crops using biotechnological tools.
Crop | Molecular Breeding Approaches | Trait(s) Improved | Reference |
---|---|---|---|
Rice | MABB | Bacterial blight resistance | [ ] |
MABB | Semi-dwarf and bacterial blight resistance | [ , ] | |
MABB | Blast resistance | [ ] | |
Wheat | MABB | Stripe rust resistance | [ ] |
MABB | Stem rust resistance | [ , ] | |
MAS | Leaf rust resistance | [ ] | |
Maize | MABB | Quality improvement | [ ] |
Cowpea | MABC | Mosaic virus (CpMV) resistance | [ ] |
Common bean | MABB | Improved drought adaptation | [ ] |
MABC | Anthracnose resistance | [ ] | |
Soybean | MAS and MABC | Several soybean cyst nematodes and multiple disease-resistant genotypes | [ ] |
MABB | Powdery mildew diseases resistance | [ ] | |
MABC | Soybean mosaic virus (SMV) resistance | [ ] | |
MABC | Free Kunitz trypsin inhibitor | [ ] | |
MABC | Elimination of lipoxygenase-2, | [ ] | |
Peanut | MABC | Introgression lines showing higher yield and increased rust resistance | [ ] |
MABC | Resistance to nematode | [ , ] | |
MABC | Enhanced oleic acid | [ , ] | |
Chickpea | MABC | Resistance to fusarium wilt | [ ] |
MABC | Resistance to Ascochyta blight | [ ] | |
MABC | Drought tolerance | [ ] | |
MABC | Elimination of lipoxygenase-2, | [ ] |
MAS—marker-assisted selection; MABB—marker-assisted backcross breeding; MABC—marker-assisted backcross.
Biotechnological tools have been efficiently used for the improvement of susceptible crop varieties. However, for the sustainable utilization of genetic resources, advanced techniques, such as NGS, HPG, and HTP, should be used to develop new crop varieties to ensure food security in the near future.

7. Conclusions
To meet the ever-increasing demand for food production, crop diversification, climate-resilient farming, etc., PGRs should be used for sustainability for future food security. However, the efficient use of PGRs can help to meet these needs, and one of the major challenges in the PGR community is to improve access to PGR collections by increasing the amount of information available about collections, through conservation, by participating in pre-breeding activities, etc. A great challenge in the PGR community is the increased demand for PGRs in the wider farming and breeding community. Maintaining genetic diversity, the conservation of PGRs, and sustainable utilization should be the priority of national and international communities. The proper monitoring of genetic erosion and genetic diversity vulnerability is crucial to protecting rare and endangered plant species. As no single technique of conservation is perfect, there is a need to practice in situ and ex situ conservation complementarily. Technical as well as financial support should be provided to farmers and local people for the proper conservation of plant genetic resources. For the management and sustainable use of PGRs, the capacities of local communities, indigenous people, farmers, breeders, extension workers, and other stakeholders, including entrepreneurs and small-scale enterprises, should be strengthened. The proper evaluation, characterization, and documentation of endemic plant species and their exact habitats should be prioritized. More frameworks and policies should be implemented for the sustainable conservation of landraces and their wild relatives. Biotechnological tools should be used for the characterization of plant genetic resources, conservation, and their utilization in breeding programs. Allele/gene mining for important traits in wild species and wild relatives of crops should be given more importance. The effective utilization of plant genetic resources would contribute to solving constraints that limit crop productivity. High-throughput genotypic and phenotypic techniques should be used for the sustainable utilization of genetic resources for future food security.
Funding Statement
This research received no external funding.
Author Contributions
Conceptualization, R.K.S.; Writing—Original Draft Preparation, R.K.S.; Writing—Review and Editing, B.S.C.; Supervision R.K.S.; Funding Acquisition, B.S.C. All authors have read and agreed to the published version of the manuscript.
Institutional Review Board Statement
Not applicable.
Informed Consent Statement
Data availability statement, conflicts of interest.
The authors declare no conflict of interest.
Disclaimer/Publisher’s Note: The statements, opinions and data contained in all publications are solely those of the individual author(s) and contributor(s) and not of MDPI and/or the editor(s). MDPI and/or the editor(s) disclaim responsibility for any injury to people or property resulting from any ideas, methods, instructions or products referred to in the content.
Frontiers for Young Minds
- Download PDF
What Is Genetic Diversity and Why Does it Matter?
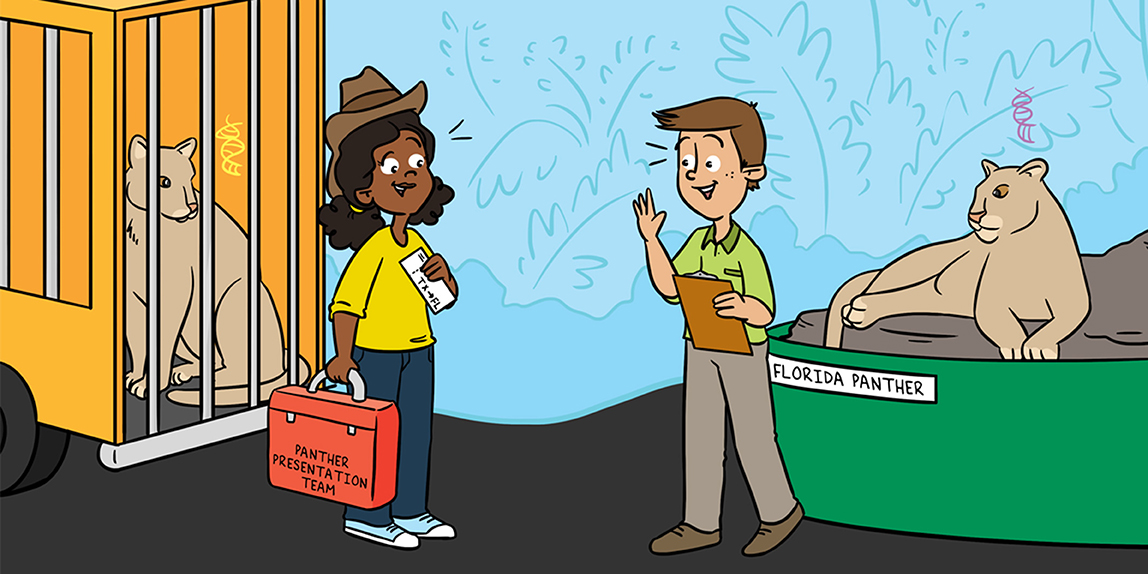
All living things on Earth contain a unique code within them, called DNA. DNA is organised into genes, similar to the way letters are organised into words. Genes give our bodies instructions on how to function. However, the exact DNA code is different even between individuals within the same species. We call this genetic diversity. Genetic diversity causes differences in the shape of bird beaks, in the flavours of tomatoes, and even in the colour of your hair! Genetic diversity is important because it gives species a better chance of survival. However, genetic diversity can be lost when populations get smaller and isolated, which decreases a species’ ability to adapt and survive. In this article, we explore the importance of genetic diversity, discuss how it is formed and maintained in wild populations, how it is lost and why that is dangerous, and what we can do to conserve it.
Why is Everything and Everyone A Little Bit Different?
Earth contains millions of different species that all look different from one another. While some species look more similar to each other than others, like lions and tigers, they will still have differences between them. Even within each species, individuals look similar to each other but they are not identical. These differences and similarities are because of many small differences between individuals’ genes . All organisms have DNA and each individual’s DNA is organised into genes. These contain the instructions to build our bodies. This is similar to the way that letters are combined to make words that then make a story. DNA can be seen as the letters, genes the words, and their instructions are the story. Small differences in DNA might change blue eyes to green, or a butterfly’s wings from black to white, like how a word can change when you replace a letter.
The combined differences in the DNA of all individuals in a species make up the genetic diversity of that species. Genetic diversity causes individuals to have different characteristics, which we can see even in our groceries. Although all tomatoes belong to the same species, the tomatoes we eat are hugely diverse, ranging from giant beefeater tomatoes to tiny cherry tomatoes. There are also hundreds of apple varieties ( Figure 1 ), that range from red to green, tart to sweet, and some apples even have pink flesh inside! Genetic diversity is what makes these types of tomatoes and apples look so different [ 1 ]. Genetic diversity is also seen in animals. For example, dogs can be large enough to pull sledges or small enough to sit nicely on your lap. All dogs are from the same species, but they look different because of genetic diversity! Though often more difficult to see, genetic diversity is also extremely important in wild animals and plants.
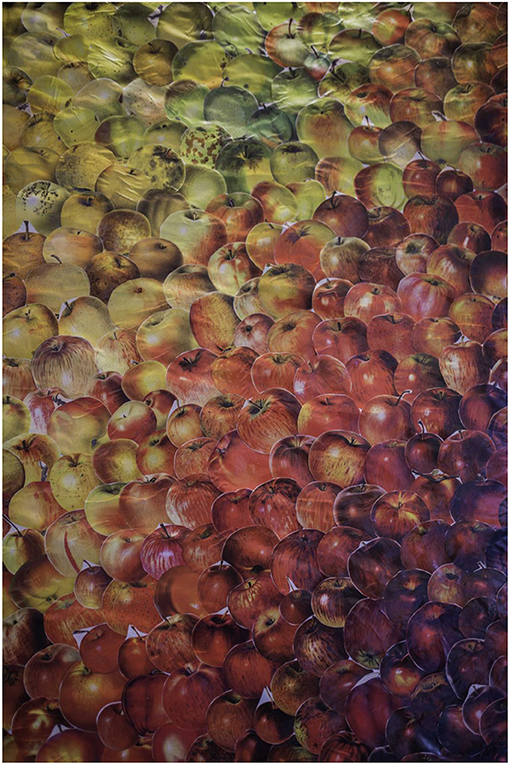
- Figure 1 - An example of genetic diversity in the food we eat.
- All these apples are one species. Different alleles of the genes that control their colour cause the apples to be green, yellow, red, or almost purple. Differences in the alleles that control flavour make each type taste different.
How is Genetic Diversity Generated?
Changes to an individual’s DNA are called mutations ( Figure 2 ). Mutations can arise when mistakes are made while cells are copying DNA, like making a spelling mistake when copying a word. These mutations make up a species’ genetic diversity. Over generations, more and more mistakes are made, leading to more mutations. Most mutations are either harmful or have no impact at all, but sometimes these mutations can cause changes that are helpful for a species. The individuals that have these helpful mutations might have greater chances of survival, and have more babies as a result [ 2 ]. This is adaptation . When a mother and a father have babies, the DNA of their baby is a mix of the parents’ DNA. Babies have two copies of every gene in their DNA, one from each parent. Copies of the same gene with different mutations are called alleles . When parents make a sperm or an egg, alleles in each parent are shuffled and recombined, and only one allele of a gene ends up in each sperm or egg cell. When the reshuffled alleles from a mother and a father are combined when sperm and eggs join, new mixes of alleles are created in the babies [ 2 , 3 ]. The mixing of alleles allows for new combinations of mutations and characteristics, adding to a species’ genetic diversity ( Figure 2 ).
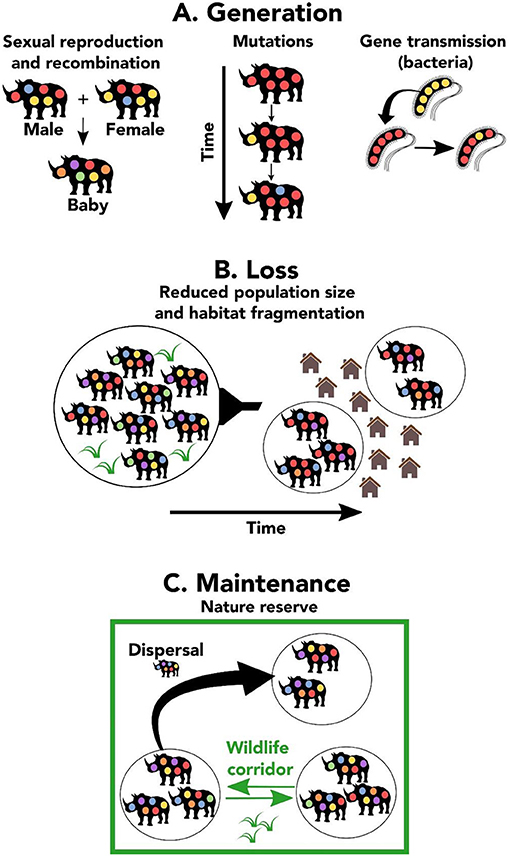
- Figure 2 - (A) Genetic diversity is generated when mutations create new alleles over time.
- Mixing alleles from parents creates new combinations of alleles in their babies. Organisms that can clone themselves, like bacteria, can pass alleles to each other. Each coloured dot represents a different allele. (B) Genetic diversity can be lost when habitat loss divides populations or when buildings or highways isolate populations. (C) Creating protected areas where individuals from different populations can migrate and spread their genes can help a species to maintain its genetic diversity.
Not all species need a mother and a father to make a baby. Bacteria can clone themselves ( Figure 2 ) and directly pass their alleles from a parent to its identical clone [ 3 ]. Any mistakes in the parent’s DNA will be passed on to the clone. Amazingly, bacteria can also give alleles to each other, even if they are not related! This is a unique way simple species like bacteria can increase their genetic diversity, without relying on the mixing of alleles between a mother and a father [ 4 ].
Why is Genetic Diversity Important?
When a species has a lot of differences in its DNA, we say that genetic diversity is high [ 2 ]. In species with high genetic diversity, there are lots of mutations in the DNA, which cause differences in the way individuals look as well as differences in important traits that we cannot see [ 2 ]. This is called adaptation . For example, some types of apples can grow better in hotter environments, thanks to their genes. The variety of characteristics in species with high genetic diversity means they are more likely to successfully cope with changes in their environment. A great example of this is seen in the peppered moths during the industrial revolution [ 4 ]. Natural genetic diversity in peppered moths produced different wing colours, ranging from light to dark. Before the Industrial Revolution, peppered moths with light wings were more common because they had the best camouflage on white tree trunks. The Industrial Revolution caused a lot of air pollution that started to cover tree trunks, making them black. Light-winged moths were no longer camouflaged and were easy prey for birds. But dark-winged individuals were now hidden! This meant that dark moths had an advantage and were more likely to live long enough to have babies. The babies of dark moths were also dark because of the alleles they inherited from their parents, so they were also more likely to survive. The dark moths had higher fitness and became more common as a result [ 4 ].
What Happens When Genetic Diversity is Low?
When few mutations are found in the DNA of a species, genetic diversity is said to be low [ 2 ]. Low genetic diversity means that there is a limited variety of alleles for genes within that species and so there are not many differences between individuals. This can mean that there are fewer opportunities to adapt to environmental changes. Low genetic diversity often occurs due to habitat loss. For example, when a species’ habitat is destroyed or broken up into small pieces, populations become small. Small, fragmented populations can lead to loss of genetic diversity because fewer individuals can survive in the remaining habitat so fewer individuals breed to pass on their alleles. In small populations, the choice of mates is also limited. Over time, individuals will all become related and will be forced to mate with relatives. This is inbreeding . Inbred animals often have two identical alleles for their genes because the same gene was passed on from both parents. If this allele has harmful mutations, an inbred baby can be unhealthy. This is called inbreeding depression [ 2 ].
If genetic diversity gets too low, species can go extinct and be lost forever. This is due to the combined effects of inbreeding depression and failure to adapt to change. In such cases, the introduction of new alleles can save a population. This is called genetic rescue [ 2 ]. In the 1990s conservation scientists had to use genetic rescue to save the Florida panther, which was threatened by extinction due to low genetic diversity ( Figure 3 ) [ 5 ]. Very few Florida panthers remained and their genetic diversity was extremely low. Many Florida panther babies were sick because of inbreeding depression. A closely related panther with high genetic diversity was present in Texas. Texan panthers were moved to Florida to have babies with the Florida panthers. This increased genetic diversity because of the mixing of alleles we spoke about before. Soon after the Texan panthers arrived, many healthy kittens were born [ 5 ].
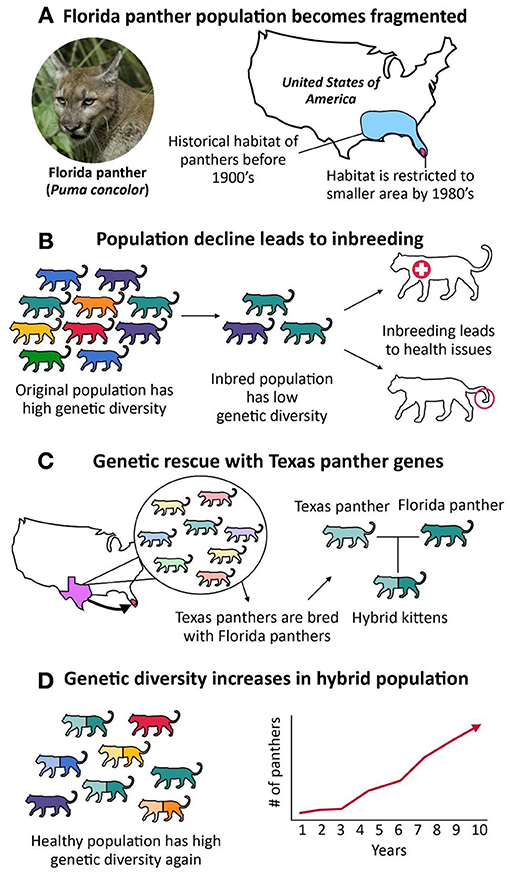
- Figure 3 - (A) The Florida panther was once widespread, with high genetic diversity.
- (B) Hunting and habitat loss reduced population size and resulted in very low genetic diversity and inbreeding. (C) Eight female panthers from Texas were moved to Florida to breed with Florida panthers. (D) When the Texas and Florida panthers bred, new alleles were introduced into the population, helping the Florida panther population become bigger and healthier over time.
What’s Happening to Genetic Diversity Around the World?
We hear a lot about the loss of species in the world, but we are also seeing a loss of genetic diversity within species. The increasing number of people on Earth and our increasing use of natural resources has reduced space and resources for wild species. Over time, many wild animal and plant populations have become smaller or more isolated. Many species have also gone through local extinctions. This has led to a global loss of genetic diversity. Scientists think that the genetic diversity within species may have declined by as much as 6% globally since the Industrial Revolution [ 6 ]. This means that many species are less able to adapt when facing new challenges, like climate change, pollution, and new diseases. If too much genetic diversity is lost, more and more species could become unhealthy and in need of conservation actions similar to the Florida panther. However, there are steps we can take to conserve and restore genetic diversity across many species.
How Do We Stop Genetic Diversity Loss?
We must preserve and protect genetic diversity. This can be done through the conservation of our remaining wild populations [ 2 ]. We can use nature reserves and wildlife bridges to reconnect wild populations that have become separated by our cities and highways. We can also restore habitats, because this will allow wild populations to get bigger. Sometimes we can even remove harmful stressors and pests so that populations can naturally regrow. We can also reintroduce species that have been lost from habitats they used to live in. Taken together, these strategies can help stop genetic diversity loss. It is important to protect genetic diversity because it is the foundation for healthy species. Healthy species are necessary for human health and for the health of the whole planet!
Gene : ↑ A section of DNA that contains the instructions for a trait.
Genetic Diversity : ↑ The overall diversity in the DNA between the individuals of a species.
Mutation : ↑ A change in an organism’s DNA. This can be a change of a single letter or a much bigger change of hundreds of letters at once.
Adaptation : ↑ The process of a species changing in order to better survive in its environment.
Alleles : ↑ Different variations of a gene caused by mutations. Many species have two alleles for every gene, one copy from each parent.
Inbreeding : ↑ Breeding between closely related individuals. Inbreeding often happens when populations are small and there are few options for mating. Inbred individuals are usually less healthy.
Inbreeding Depression : ↑ Inbred individuals share ancestors and are more likely to have identical copies of genes. If these genes contain harmful mutations, they will be expressed and cause lower health of inbred individuals.
Genetic Rescue : ↑ A conservation strategy, new individuals are moved into a population to increase genetic diversity and improve population health.
Conflict of Interest
The authors declare that the research was conducted in the absence of any commercial or financial relationships that could be construed as a potential conflict of interest.
[1] ↑ Meyer, R., and Purugganan, M. 2013. Evolution of crop species: genetics of domestication and diversification. Nat. Rev. Genet. 14:840–52. doi: 10.1038/nrg3605
[2] ↑ Frankham, R., Ballou, J. D., and Briscoe, D. A. 2002. Introduction to Conservation Genetics. Cambridge: Cambridge University Press. p. 617.
[3] ↑ Emamalipour M., Seidi K., Zununi V. S., Jahanban-Esfahlan A., Jaymand M., Majdi H., et al. 2020. Horizontal gene transfer: from evolutionary flexibility to disease progression. Front. Cell. Dev. Biol. 8:229. doi: 10.3389/fcell.2020.00229
[4] ↑ Cook, L. M., and Saccheri, I. J. 2013. The peppered moth and industrial melanism: evolution of a natural selection case study. Heredity 110:207–12. doi: 10.1038/hdy.2012.92
[5] ↑ Johnson, W. E., Onorato, D. P., Roelke, M. E., Land, E. D., Cunningham, M., Belden, R. C., et al. 2010. Genetic restoration of the Florida panther. Science . 329:1641–5. doi: 10.1126/science.1192891
[6] ↑ Leigh, D. M., Hendry, A. P., Vázquez-Domínguez, E., and Friesen, V. L. 2019. Estimated six per cent loss of genetic variation in wild populations since the industrial revolution. Evol. Appl. 12:1505–12. doi: 10.1111/eva.12810
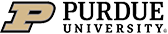
GENETIC DIVERSITY OF SPOTTED TURTLE (CLEMMYS GUTTATA) POPULATIONS IN A FRAGMENTED LANDSCAPE
Turtle species are facing losses to genetic variation caused by habitat fragmentation and large-scale landscape changes caused by humans. These losses to genetic variation disproportionally effect species that have become rare due to human interaction. Spotted Turtle (Clemmys guttata) is one such species that has become rare across a large portion of its natural geographic range including the Midwest. I examined genetic variation in spotted turtles in Indiana and Ohio to delineate populations and assess levels of genetic variation within populations. Eight microsatellite loci were used to genotype 94 individuals, which originated from four sites in Indiana and two sites in Ohio. Bayesian and ordination based cluster analyses identified three genetic clusters within the sample. Individuals from one Ohio locality were largely clustered alone and had a significant degree of differentiation from all of the Indiana sites. A majority of the Indiana sites had individuals that were clustered into both of the two remaining clusters with the exception of IN-1 which only had individuals grouped into the first inferred cluster. The two sites geographically closest to one another IN-2 and IN-4, both had individuals grouped into the first and third inferred clusters. Differentiation statistics disagreed on the level of structure between these two populations. Two of the six sites had detectable bottlenecks, even though levels of genetic allelic diversity and heterozygosity were relatively high and comparable to populations investigated in other studies. Overall, population structure was identified among localities in Indiana and Ohio that may warrant independent management. Populations where the bottlenecks were detected may be of special concern in the future as they continue to be subjected to isolation.
Degree Type
- Master of Science
- Biological Sciences
Campus location
Advisor/supervisor/committee chair, additional committee member 2, additional committee member 3, usage metrics.
- Plant cell and molecular biology
- Animal cell and molecular biology
- Population ecology
- Anthropological genetics
- Genetics not elsewhere classified
- Behavioural genetics

Global Journal of Agricultural Research (GJAR)
- +44(0)1634 560711
- [email protected]
- 124 City Road, London, EC1V 2NX. UK

Genetic Diversity and Its Impact in Enhancement of Crop Plants
() , , (), –
Citation: Temesgen Begna, Hayilu Gichile and Werkissa Yali (2022) Genetic Diversity and Its Impact in Enhancement of Crop Plants, Global Journal of Agricultural Research , Vol.10, No.2, pp.13-25
Plant breeding is a science that focuses on the development of new plant varieties in a systematic and ongoing method. It takes advantage of genetic variation among individuals within a plant species and combines desired traits to produce new and improved varieties. Plant breeding depends on genetic variety, and new variation is critical for introducing interesting characteristics into breeding programs. Genetic erosion is a term used to describe the loss of variation in crops as a result of agricultural modernization. Plant breeding has had a significant impact on food production and will continue to play an important role in ensuring global food security. Plant breeding can be broadly described as changes in plants caused by human use, ranging from unintended modifications generated by the introduction of agriculture through the use of molecular tools for precision breeding. Plant breeding based on observed variation by selection of plants based on natural variants appearing in nature or within traditional varieties; plant breeding based on controlled mating by selection of plants presenting recombination of desirable genes from different parents; and plant breeding based on monitored recombination by selection of specific genes or marker profiles. Continuous use of traditional breeding methods in a given species may reduce the gene pool from which cultivars are derived, making crops more susceptible to biotic and abiotic challenges and impeding future advancement. Plant breeding’s primary objectives are ‘high yield, high quality, and quantity, extension of climate and soil adaptability ability, and tolerance or resistance to pests and diseases.’ Plant breeders achieve these goals by exploiting genetic differences between plants. The range of the genetic base, as measured by genetic diversity, is a limiting factor in successful adaptation to environmental conditions and plant breeding. Many challenges in plant breeding require genetic variation, which can be found in the biodiversity of plant genetic resources such as breeding lines, landraces, primitive forms, wilds and wild relatives, and weed races.
Keywords: genetic diversity; adaptation; molecular marker; diversity analysis; gene pyramiding
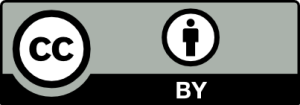
This work by European American Journals is licensed under a Creative Commons Attribution-NonCommercial-NoDerivs 4.0 Unported License
Recent Publications
Determinants affecting input-output relationship in yam production in ikom agricultural zone of cross river state, nigeria, profitability and determinants of output of rice production in anambra state, nigeria, agricultural productivity and postharvest loss among cassava farmers, in anambra state, nigeria, physicochemical soil analysis of rejuvenated cocoa plantation farms in obaagun area of osun state.
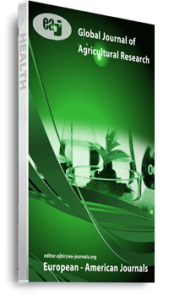
Author Guidelines Submit Papers Review Status
British Journal of Marketing Studies (BJMS) European Journal of Accounting, Auditing and Finance Research (EJAAFR) European Journal of Business and Innovation Research (EJBIR) European Journal of Hospitality and Tourism Research (EJHTR) European Journal of Logistics, Purchasing and Supply Chain Management (EJLPSCM) Global Journal of Human Resource Management (GJHRM) International Journal of Business and Management Review (IJBMR) International Journal of Community and Cooperative Studies (IJCCS) International Journal of Management Technology (IJMT) International Journal of Small Business and Entrepreneurship Research (IJSBER)
British Journal of Education (BJE) European Journal of Training and Development Studies (EJTDS) International Journal of Education, Learning and Development (IJELD) International Journal of Interdisciplinary Research Methods (IJIRM) International Journal of Quantitative and Qualitative Research Methods (IJQQRM) International Journal of Vocational and Technical Education Research (IJVTER)
British Journal of Earth Sciences Research (BJESR) British Journal of Environmental Sciences (BJES) European Journal of Computer Science and Information Technology (EJCSIT) European Journal of Material Sciences (EJMS) European Journal of Mechanical Engineering Research (EJMER) European Journal of Statistics and Probability (EJSP) Global Journal of Pure and Applied Chemistry Research (GJPACR) International Journal of Civil Engineering, Construction and Estate Management (IJCECEM) International Journal of Electrical and Electronics Engineering Studies (IJEEES) International Journal of Energy and Environmental Research (IJEER) International Journal of Engineering and Advanced Technology Studies (IJEATS) International Journal of Environment and Pollution Research (IJEPR) International Journal of Manufacturing, Material and Mechanical Engineering Research (IJMMMER) International Journal of Mathematics and Statistics Studies (IJMSS) International Journal of Network and Communication Research (IJNCR) International Research Journal of Natural Sciences (IRJNS) International Research Journal of Pure and Applied Physics (IRJPAP)
British Journal of English Linguistics (BJEL) European Journal of English Language and Literature Studies (EJELLS) International Journal of African Society, Cultures and Traditions (IJASCT) International Journal of Asian History, Culture and Tradition (IJAHCT) International Journal of Developing and Emerging Economies (IJDEE) International Journal of English Language and Linguistics Research (IJELLR) International Journal of English Language Teaching (IJELT)
International Journal of Agricultural Extension and Rural Development Studies (IJAERDS) International Journal of Animal Health and Livestock Production Research (IJAHLPR) International Journal of Cancer, Clinical Inventions and Experimental Oncology (IJCCEO) International Journal of Cell, Animal Biology and Genetics (IJCABG) International Journal of Dentistry, Diabetes, Endocrinology and Oral Hygiene (IJDDEOH) International Journal of Ebola, AIDS, HIV and Infectious Diseases and Immunity (IJEAHII) International Journal of Entomology and Nematology Research (IJENR) International Journal of Environmental Chemistry and Ecotoxicology Research (IJECER) International Journal of Fisheries and Aquaculture Research (IJFAR) International Journal of Horticulture and Forestry Research (IJHFR) International Journal of Micro Biology, Genetics and Monocular Biology Research (IJMGMR) International Journal of Nursing, Midwife and Health Related Cases (IJNMH) International Journal of Nutrition and Metabolism Research (IJNMR) International Journal of Public Health, Pharmacy and Pharmacology (IJPHPP) International Journal of Weather, Climate Change and Conservation Research (IJWCCCR) International Journal Water Resources Management and Irrigation Engineering Research (IJWEMIER)
British Journal of Psychology Research (BJPR) European Journal of Agriculture and Forestry Research (EJAFR) European Journal of Biology and Medical Science Research (EJBMSR) European Journal of Botany, Plant Sciences and Phytology (EJBPSP) European Journal of Educational and Development Psychology (EJEDP) European Journal of Food Science and Technology (EJFST) Global Journal of Agricultural Research (GJAR) International Journal of Health and Psychology Research (IJHPR)
Global Journal of Arts, Humanities and Social Sciences (GJAHSS) Global Journal of Political Science and Administration (GJPSA) Global Journal of Politics and Law Research (GJPLR) International Journal of Development and Economic Sustainability (IJDES) International Journal of History and Philosophical Research (IJHPHR) International Journal of International Relations, Media and Mass Communication Studies (IJIRMMCS) International Journal of Music Studies (IJMS) International Journal of Non-Governmental Organizations (NGOs) and Essays (IJNGOE) International Journal of Physical and Human Geography (IJPHG) International Journal of Sociology and Anthropology Research (IJSAR)
International Journal of Biochemistry, Bioinformatics and Biotechnology Studies (IJBBBS) International Journal of Coal, Geology and Mining Research (IJCGMR) International Journal of Geography and Regional Planning Research (IJGRPR) International Journal of Library and Information Science Studies (IJLISS) International Journal of Petroleum and Gas Engineering Research (IJPGER) International Journal of Petroleum and Gas Exploration Management (IJPGEM) International Journal of Physical Sciences Research (IJPSR) International Journal of Scientific Research in Essays and Case Studies (IJSRECS)
Don't miss any Call For Paper update from EA Journals
Fill up the form below and get notified everytime we call for new submissions for our journals.
Thank you for visiting nature.com. You are using a browser version with limited support for CSS. To obtain the best experience, we recommend you use a more up to date browser (or turn off compatibility mode in Internet Explorer). In the meantime, to ensure continued support, we are displaying the site without styles and JavaScript.
- View all journals
- Explore content
- About the journal
- Publish with us
- Sign up for alerts
- Perspective
- Published: 24 June 2024
A proposal for an inclusive working definition of genetic discrimination to promote a more coherent debate
- Beatrice Kaiser 1 ,
- Diya Uberoi 1 ,
- Maili C. Raven-Adams 2 ,
- Katherine Cheung ORCID: orcid.org/0009-0003-3529-4109 1 ,
- Andreas Bruns 3 ,
- Subhashini Chandrasekharan 4 ,
- Margaret Otlowski 5 ,
- Anya E. R. Prince 6 ,
- Jane Tiller ORCID: orcid.org/0000-0003-3906-6632 7 ,
- Arzoo Ahmed 8 ,
- Yvonne Bombard ORCID: orcid.org/0000-0002-9516-4539 9 , 10 ,
- Charles Dupras 11 ,
- Palmira Granados Moreno 1 ,
- Rosalyn Ryan 12 ,
- Augusto Valderrama-Aguirre ORCID: orcid.org/0000-0002-5255-1440 13 &
- Yann Joly ORCID: orcid.org/0000-0002-8775-2322 1
Nature Genetics ( 2024 ) Cite this article
148 Accesses
4 Altmetric
Metrics details
- Health care
Genetic discrimination is an evolving phenomenon that impacts fundamental human rights such as dignity, justice and equity. Although, in the past, various definitions to better conceptualize genetic discrimination have been proposed, these have been unable to capture several key facets of the phenomenon. In this Perspective, we explore definitions of genetic discrimination across disciplines, consider criticisms of such definitions and show how other forms of discrimination and stigmatization can compound genetic discrimination in a way that affects individuals, groups and systems. We propose a nuanced and inclusive definition of genetic discrimination, which reflects its multifaceted impact that should remain relevant in the face of an evolving social context and advancing science. We argue that our definition should be adopted as a guiding academic framework to facilitate scientific and policy discussions about genetic discrimination and support the development of laws and industry policies seeking to address the phenomenon.
This is a preview of subscription content, access via your institution
Access options
Access Nature and 54 other Nature Portfolio journals
Get Nature+, our best-value online-access subscription
24,99 € / 30 days
cancel any time
Subscribe to this journal
Receive 12 print issues and online access
195,33 € per year
only 16,28 € per issue
Buy this article
- Purchase on Springer Link
- Instant access to full article PDF
Prices may be subject to local taxes which are calculated during checkout
Similar content being viewed by others
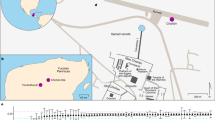
Ancient genomes reveal insights into ritual life at Chichén Itzá

Next-generation data filtering in the genomics era
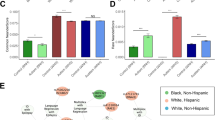
Enrichment of a subset of Neanderthal polymorphisms in autistic probands and siblings
Otlowski, M., Taylor, S. & Bombard, Y. Genetic discrimination: international perspectives. Annu. Rev. Genomics Hum. Genet. 13 , 433–454 (2012).
CAS Google Scholar
Bombard, Y. et al. Perceptions of genetic discrimination among people at risk for Huntington’s disease: a cross sectional survey. BMJ 338 , b2175 (2009).
Article PubMed PubMed Central Google Scholar
Chapman, C. R., Mehta, K. S., Parent, B. & Caplan, A. L. Genetic discrimination: emerging ethical challenges in the context of advancing technology. J. Law Biosci. 7 , lsz016 (2020).
Google Scholar
Granados Moreno, P., Ngueng Feze, I. & Joly, Y. Does the end justify the means? A comparative study of the use of DNA testing in the context of family reunification. J. Law Biosci. 4 , 250–281 (2017).
Joly, Y. & Dalpe, G. Genetic discrimination still casts a large shadow in 2022. Eur. J. Hum. Genet. 30 , 1320–1322 (2022).
Rothstein, M. A. & Anderlik, M. R. What is genetic discrimination, and when and how can it be prevented? Genet. Med. 3 , 354–358 (2001).
Article CAS PubMed Google Scholar
Dupras, C. et al. Potential (mis)use of epigenetic age estimators by private companies and public agencies: human rights law should provide ethical guidance. Environ. Epigenetics 5 , dvz018 (2019).
Ct, W. & Morris, J. R. Genes, genetics, and epigenetics: a correspondence. Science 293 , 1103–1105 (2001).
Article Google Scholar
Knoppers, B. M., Bernier, A., Granados Moreno, P. & Pashayan, N. Of screening, stratification, and scores. J. Pers. Med. 11 , 736 (2021).
Polygenic Risk Score Task Force of the International Common Disease Alliance. Responsible use of polygenic risk scores in the clinic: potential benefits, risks and gaps. Nat. Med. 27 , 1876–1884 (2021).
Article CAS Google Scholar
Martin, A. R. et al. Clinical use of current polygenic risk scores may exacerbate health disparities. Nat. Genet . 51 , 584–591 (2019).
Article CAS PubMed PubMed Central Google Scholar
General Conference of the United Nations Educational, Scientific and Cultural Organization (UNESCO). Universal Declaration on the Human Genome and Human Rights . https://www.unesco.org/en/legal-affairs/universal-declaration-human-genome-and-human-rights (1997).
Joly, Y., Dupras, C., Pinkesz, M., Tovino, S. A. & Rothstein, M. A. Looking beyond GINA: policy approaches to address genetic discrimination. Annu. Rev. Genomics Hum. Genet. 21 , 491–507 (2020).
Wauters, A. & Van Hoyweghen, I. Global trends on fears and concerns of genetic discrimination: a systematic literature review. J. Hum. Genet. 61 , 275–282 (2016).
US Equal Employment Opportunity Commission (EEOC). The Genetic Information Nondiscrimination Act of 2008. https://www.eeoc.gov/statutes/genetic-information-nondiscrimination-act-2008 (2008).
Government of Canada. Genetic Non-Discrimination Act (S.C. 2017). https://laws-lois.justice.gc.ca/eng/acts/g-2.5/page-1.html (2017).
House of Commons, Parliament of Canada. Debates (Hansard), no. 77 (42-1). https://www.ourcommons.ca/DocumentViewer/en/42-1/house/sitting-77/hansard#9041916 (2016).
Spector-Bagdady, K., Prince, A. E. R., Yu, J.-H. & Appelbaum, P. S. Analysis of state laws on informed consent for clinical genetic testing in the era of genomic sequencing. Am. J. Med. Genet. C Semin. Med. Genet. 178 , 81–88 (2018).
Somerville, M. ‘Law, marching with medicine but in the rear and limping a little’: ethics as ‘first aid’ for law. In Ethik und Recht — Die Ethisierung des Rechts/Ethics and Law — The Ethicalization of Law (eds Vöneky, S. et al.) 67–102 (Springer, 2013).
Genetic Discrimination Observatory. Approaches to Prevent Genetic Discrimination. https://gdo.global/en/gdo-map-approaches (2019).
Tiller, J., Lacaze, P. & Otlowski, M. The Australian moratorium on genetics and life insurance: evaluating policy compared to parliamentary recommendations regarding genetic discrimination. Public Health Res. Pract. 32 , 3242235 (2022).
Article PubMed Google Scholar
Dupras, C., Song, L., Saulnier, K. M. & Joly, Y. Epigenetic discrimination: emerging applications of epigenetics pointing to the limitations of policies against genetic discrimination. Front. Genet. 9 , 202 (2018).
Sud, A. et al. Realistic expectations are key to realising the benefits of polygenic scores. BMJ 380 , e073149 (2023).
Plümecke, T. Genes, symptoms, and the ‘asymptomatic ill’: toward a broader understanding of genetic discrimination. New Genet. Soc. 35 , 124–148 (2016).
Wolf, S. M. Beyond “genetic discrimination”: Toward the broader harm of geneticism. J. Law Med. Ethics 23 , 345–353 (1995).
Taylor, S., Treloar, S., Barlow-Stewart, K., Stranger, M. & Otlowski, M. Investigating genetic discrimination in Australia: a large-scale survey of clinical genetics clients. Clin. Genet. 74 , 20–30 (2008).
Billings, P. R. et al. Discrimination as a consequence of genetic testing. Am. J. Hum. Genet. 50 , 476–482 (1992).
CAS PubMed PubMed Central Google Scholar
Bombard, Y. et al. Beyond the patient: the broader impact of genetic discrimination among individuals at risk of Huntington disease. Am. J. Med. Genet. B Neuropsychiatr. Genet. 159B , 217–226 (2012).
Halliday, J. L., Collins, V. R., Aitken, M. A., Richards, M. P. M. & Olsson, C. A. Genetics and public health—evolution, or revolution? J. Epidemiol. Community Health 58 , 894–899 (2004).
Epps, P. G. Policy before practice. Am. J. Pharmacogenomics 3 , 405–418 (2003).
Lemke, T. Perspectives on Genetic Discrimination . (Routledge, United Kingdom, 2013).
Book Google Scholar
Rothstein, M. A. GINA, the ADA, and genetic discrimination in employment. J. Law Med. Ethics 36 , 837–840 (2008).
Prince, A. E. R. & Berkman, B. E. When does an illness begin: genetic discrimination and disease manifestation. J. Law Med. Ethics 40 , 655–664 (2012).
Murray, T. H. Is genetic exceptionalism past its sell-by date? On genomic diaries, context, and content. Am. J. Bioeth. 19 , 13–15 (2019).
Knoppers, B. M. & Beauvais, M. J. S. Basta con il biolaw: what about knowledge and trust? BioLaw J. https://doi.org/10.15168/2284-4503-769 (2021).
Murray, T. Genetic exceptionalism and ‘future diaries’: is genetic information different from other medical information? In Genetic Secrets: Protecting Privacy and Confidentiality in the Genetic Era 60–73 (Yale University, 1997).
Joly, Y. et al. The Genetic Discrimination Observatory: Confronting novel issues in genetic discrimination. Trends Genet. 37 , 951–954 (2021).
Iltis, A. S., Rolf, L., Yaeger, L., Goodman, M. S. & DuBois, J. M. Attitudes and beliefs regarding race-targeted genetic testing of Black people: A systematic review. J. Genet. Couns. 32 , 435–461 (2023).
Hausman, D. M. Group risks, risks to groups, and group engagement in genetics research. Kennedy Inst. Ethics J. 17 , 351–369 (2007).
Hindorff, L. A. et al. Prioritizing diversity in human genomics research. Nat. Rev. Genet. 19 , 175–185 (2018).
National Academies of Sciences, Engineering, and Medicine. Using Population Descriptors in Genetics and Genomics Research: a New Framework for an Evolving Field https://doi.org/10.17226/26902 (National Academies, 2023).
Berghs, M. & Dyson, S. M. Intersectionality and employment in the United Kingdom: where are all the Black disabled people? Disabil. Soc. 37 , 543–566 (2022).
Lobo, I. Genetic Testing Spotlight. Nature https://www.nature.com/scitable/spotlight/genetic-testing-13782065/ (2014).
Perbal, L. The ‘warrior gene’ and the Mãori people: the responsibility of the geneticists. Bioethics 27 , 382–387 (2013).
Forzano, F., Genuardi, M. & Moreau, Y. ESHG warns against misuses of genetic tests and biobanks for discrimination purposes. Eur. J. Hum. Genet. 29 , 894–896 (2021).
Reid, P., Cormack, D. & Paine, S.-J. Colonial histories, racism and health—the experience of Māori and Indigenous peoples. Public Health 172 , 119–124 (2019).
Burnett-Hartman, A. N. et al. Return of research-related genetic test results and genetic discrimination concerns: facilitators and barriers of genetic research participation in diverse groups. Public Health Genomics 23 , 59–68 (2020).
Borrell, L. N. et al. Race and genetic ancestry in medicine — a time for reckoning with racism. N. Engl. J. Med. 384 , 474–480 (2021).
Walajahi, H., Wilson, D. R. & Hull, S. C. Constructing identities: the implications of DTC ancestry testing for tribal communities. Genet. Med. 21 , 1744–1750 (2019).
Garrison, N. A. Genetic ancestry testing with tribes: ethics, identity & health implications. Daedalus 147 , 60–69 (2018).
Brandt-Rauf, S. I., Raveis, V. H., Drummond, N. F., Conte, J. A. & Rothman, S. M. Ashkenazi Jews and breast cancer: the consequences of linking ethnic identity to genetic disease. Am. J. Public Health 96 , 1979–1988 (2006).
Link, B. G. & Phelan, J. C. Conceptualizing stigma. Annu. Rev. Sociol. 27 , 363–385 (2001).
Lapham, E. V., Kozma, C. & Weiss, J. O. Genetic discrimination: perspectives of consumers. Science 274 , 621–624 (1996).
Geller, L. N. et al. Individual, family, and societal dimensions of genetic discrimination: a case study analysis. Sci. Eng. Ethics 2 , 71–88 (1996).
Otlowski, M. F. A., Stranger, M. J. A., Taylor, S., Barlow-Stewart, K. & Trealoar, S. Investigating genetic discrimination in Australia: perceptions and experiences of clinical genetics service clients regarding coercion to test, insurance and employment. Aust. J. Emerg. Tech. Soc. 5 , 63–83 (2007).
Erwin, C. et al. Perception, experience, and response to genetic discrimination in Huntington disease: the international RESPOND-HD study. Am. J. Med. Genet. B Neuropsychiatr. Genet. 153B , 1081–1093 (2010).
Ajunwa, I. Genetic testing meets big data: torts and contract law issues. SSRN https://papers.ssrn.com/abstract=2460891 (2014).
Federal Ministry of Justice. German Genetic Diagnostics Act https://www.medgen-mainz.de/en/for-physicians/request-forms/german-genetic-diagnostics-act/ (2020).
MOH. Moratorium on Genetic Testing and Insurance https://www.moh.gov.sg/resources-statistics/moratorium-on-genetic-testing-and-insurance (2024).
Government of the United Kingdom. Code on genetic testing and insurance. GOV.UK https://www.gov.uk/government/publications/code-on-genetic-testing-and-insurance (2022).
Financial Services Council, Australia. FSC Announces Moratorium on Genetic Tests for Life Insurance to Start in July 2019 https://fsc.org.au/resources (2018).
Royal Society of New ZealandTe Apārangi. Code of Professional Standards and Ethics in Science, Technology, and the Humanities. https://www.royalsociety.org.nz/who-we-are/our-rules-and-codes/code-of-professional-standards-and-ethics/ (2019).
Ministry of Science and Technology. National Research Ethics Review Guideline (Fifth Edition) https://healthresearchwebafrica.org.za/en/ethiopia/ethics_2124 (2014).
Indian Council of Medical Research. National Ethical Guidelines for Biomedical and Health Research Involving Human Participants https://ethics.ncdirindia.org/asset/pdf/ICMR_National_Ethical_Guidelines.pdf (2017).
Saba. Discrimination in health insurance against individuals based on their genetic disposition, is unconstitutional. SCC Times https://www.scconline.com/blog/post/2018/03/01/discrimination-health-insurance-individuals-based-genetic-disposition-unconstitutional/ (1 March 2018).
Download references
Acknowledgements
All authors are members of the GA4GH and/or the GDO. We thank the members of the GDO and the GA4GH genetic discrimination group for their contributions to discussions on genetic discrimination. We also thank P. Ayyappaswamy, N. Palmour and E. Kondrup from the McGill Centre of Genomics and Policy for editorial assistance with the manuscript. We are grateful for the financial support of Supporting Canadian Leadership in International Genomic Data Sharing through the GA4GH funded by Genome Canada and Génome Québec. Participation of A.V.-A. in this work was supported by the FAPA project (PVI0122029) from the Universidad de Los Andes. Participation of Y.B. in this work was supported by the Canada Research Chair in Genomics Health Services and Policy.
Author information
Authors and affiliations.
Centre of Genomics and Policy, McGill University, Montreal, Quebec, Canada
Beatrice Kaiser, Diya Uberoi, Katherine Cheung, Palmira Granados Moreno & Yann Joly
Global Alliance for Genomics & Health, London, UK
Maili C. Raven-Adams
The German Human Genome–Phenome Archive, University Hospital, Heidelberg, Germany
Andreas Bruns
National Institutes of Health, Bethesda, MD, USA
Subhashini Chandrasekharan
Centre for Health, Law and Emerging Technologies, University of Oxford, Oxford, UK
Margaret Otlowski
The University of Iowa College of Law, Iowa City, IA, USA
Anya E. R. Prince
Monash University, Parkville, Victoria, Australia
Jane Tiller
Genomics England, London, UK
Arzoo Ahmed
Institute of Health Policy, Management and Evaluation, University of Toronto, Toronto, Ontario, Canada
Yvonne Bombard
Genomics Health Services Research Program, St. Michael’s Hospital, Unity Health Toronto, Toronto, Canada
University of Montreal, Montreal, Quebec, Canada
Charles Dupras
Healthfox US Inc., Chicago, IL, USA
Rosalyn Ryan
Department of Biological Sciences, Universidad de Los Andes, Bogotá DC, Colombia
Augusto Valderrama-Aguirre
You can also search for this author in PubMed Google Scholar
Contributions
Conceptualization: B.K., M.C.R.-A. and Y.J. Analysis: B.K., M.C.R.-A. and K.C. Writing: B.K., D.U., M.C.R.-A., K.C., A.B., S.C., M.O., A.E.R.P., J.T. and Y.J. Manuscript review: B.K., D.U., M.C.R.-A., K.C., A.B., S.C., M.O., A.E.R.P., J.T., A.A., Y.B., C.D., P.G.M., R.R., A.V.-A. and Y.J.
Corresponding author
Correspondence to Yann Joly .
Ethics declarations
Competing interests.
Y.J. is the Director of the GDO and the Co-Lead of the Global Alliance for Genomics and Health (GA4GH) Regulatory and Ethics Work Stream. Y.B. is the cofounder and CEO of Genetics Adviser. All other authors declare no competing interests.
Peer review
Peer review information.
Nature Genetics thanks Carolyn Chapman and the other, anonymous, reviewer(s) for their contribution to the peer review of this work.
Additional information
Publisher’s note Springer Nature remains neutral with regard to jurisdictional claims in published maps and institutional affiliations.
Rights and permissions
Springer Nature or its licensor (e.g. a society or other partner) holds exclusive rights to this article under a publishing agreement with the author(s) or other rightsholder(s); author self-archiving of the accepted manuscript version of this article is solely governed by the terms of such publishing agreement and applicable law.
Reprints and permissions
About this article
Cite this article.
Kaiser, B., Uberoi, D., Raven-Adams, M.C. et al. A proposal for an inclusive working definition of genetic discrimination to promote a more coherent debate. Nat Genet (2024). https://doi.org/10.1038/s41588-024-01786-8
Download citation
Received : 29 August 2023
Accepted : 03 May 2024
Published : 24 June 2024
DOI : https://doi.org/10.1038/s41588-024-01786-8
Share this article
Anyone you share the following link with will be able to read this content:
Sorry, a shareable link is not currently available for this article.
Provided by the Springer Nature SharedIt content-sharing initiative
Quick links
- Explore articles by subject
- Guide to authors
- Editorial policies
Sign up for the Nature Briefing newsletter — what matters in science, free to your inbox daily.

- Share full article
Advertisement
Supported by
The Last Stand of the Woolly Mammoths
The species survived on an island north of Siberia for thousands of years, scientists reported, but were most likely plagued by genetic abnormalities.

By Carl Zimmer
For millions of years, mammoths lumbered across Europe, Asia and North America. Starting roughly 15,000 years ago, the giant animals began to vanish from their vast range until they survived on only a few islands.
Eventually they disappeared from those refuges, too, with one exception: Wrangel Island, a land mass the size of Delaware over 80 miles north of the coast of Siberia. There, mammoths held on for thousands of years — they were still alive when the Great Pyramids were built in Egypt.
When the Wrangel Island mammoths disappeared 4,000 years ago, mammoths became extinct for good.
For two decades, Love Dalén, a geneticist at Stockholm University, and his colleagues have been extracting bits of DNA from fossils on Wrangel Island. In recent years, they have gathered entire mammoth genomes. On Thursday, they published a reconstruction of the genetic history of these enigmatic animals.
The scientists concluded that the island’s population was founded about 10,000 years ago by a tiny herd made up of fewer than 10 animals. The colony survived for 6,000 years, but the mammoths suffered from a host of genetic disorders.
Oliver Ryder, the director of conservation genetics at San Diego Zoo Wildlife Alliance, said that the study held important lessons for trying to save species from extinction today. It shows that inbreeding could cause long-term harm.
“The mammoth study allows one to examine that process over thousands of years,” said Dr. Ryder, who was not involved in the new study. “We don’t have data like that for the species we are trying to save now.”
We are having trouble retrieving the article content.
Please enable JavaScript in your browser settings.
Thank you for your patience while we verify access. If you are in Reader mode please exit and log into your Times account, or subscribe for all of The Times.
Thank you for your patience while we verify access.
Already a subscriber? Log in .
Want all of The Times? Subscribe .

IMAGES
VIDEO
COMMENTS
Romiguier, J. et al. Comparative population genomics in animals uncovers the determinants of genetic diversity. Nature 515, 261-263 (2014). This study shows a comparative analysis of patterns of ...
editing, commenting, and offering advice on my thesis. A huge thank you to Dave Rowe and Nate Nye, employees of the Wisconsin Department of Natural Resources (WIDNR), for being so instrumental in designing this project, helping carry ... reduced genetic diversity and effective population sizes (Yamamoto et al. 2004; Blanchet et al. 2010 ...
1. Introduction. Genetic diversity (GD) is essential for populations persistence, increasing their evolutionary potential (Lavergne and Molofsky, 2007; Laikre et al., 2020).Populations with high GD tend to have a higher adaptive potential to cope with changing environments (Markert et al., 2010; Ellegren and Galtier, 2016), which is becoming increasingly important in the context of global ...
Genetic diversity helps to adapt to environmental variability. Organisms live in complex environment that vary in spatial and temporal scale and. is characterized by several factors such as ...
Perhaps the most widely cited statistic about human genetic diversity is that any two humans differ, on average, at about 1 in 1,000 DNA base pairs (0.1%). Human genetic diversity is substantially lower than that of many other species, including our nearest evolutionary relative, the chimpanzee. Genetic diversity is a function of a population's
A rectangular data set was first created from the SSR data in Microsoft. Excel (Microsoft Corp.) with the alleles as the rows, and the accessions as the columns, using "1" for presence of an allele and "0" for absence. Dice coefficient for similarity and UPGMA clustering were chosen to create a dendrogram of the data.
Genetic diversity is a fundamental source of biodiversity which has been defined by different authors as "any measure that quantifies the ... Nonić M (2016) Improving mass production of leaf-ornamental beech cultivars by grafting. Doctoral thesis, University of Belgrade - Faculty of Forestry, 280 pages. Google Scholar NRC - National ...
Genetic Diversity Analysis of Sorghum [Sorghum bicolor (L.) Moench] in Ethiopia based on Quantitative Traits and Microsatellite Markers A Thesis Submitted to the Institute of Biotechnology, Addis Ababa University in Partial Fulfillment of the Requirements for the Degree of Master of Science in Biotechnology By Wubshet Mamo Umie July 2021
Genetic diversity helps breeders to maintain the crossbred varieties, which leads to sustaining the desirable traits of the varieties, such as quality characteristics and tolerance to various stresses. In general, plant genetic resources (PGRs) are the total hereditary material, which includes all the alleles of various genes, present in a crop ...
Thesis. Full-text available. Oct 2020; Achyuta Basak; ... Genetic diversity analysis is an important component in conventional and marker-assisted breeding. The objective of this study was to ...
2 THE UNIVERSITY OF ARIZONA GRADUATE COLLEGE As members of the Master's Committee, we certify that we have read the document prepared by Rebecca Coleman, titled Diversity, Equity, Inclusion, and Justice in Genetic Counseling: a Focus on Indigenous Peoples and recommend that it be accepted as fulfilling the thesis requirement for the Master's Degree in Genetic Counseling.
GENETIC DIVERSITY AMONG ELITE MAIZE(Zea mays L.) INBRED LINES USING SIMPLE SEQUENCE REPEAT MARKERS ... This thesis has been submitted in partial fulfillment for the requirement of an advanced MSc degree at Jimma University and is deposited at the University Library to be made ...
Analysis of genetic relationships in crops is a prerequisite for crop breeding programs, as it serves to provide information about genetic variation (10). lack of genetic diversity can potentially lower the resistance of cropping systems to unknown or evolving pests, pathogens, or adverse environmental conditions.
By my signature below, I declare and confirm that this thesis is my work and all sources of materials used for this thesis has been acknowledged. I have followed all proper and technical principles of scholarships in the research, data collection, data analysis and accomplishment of this thesis.
Gene: ↑ A section of DNA that contains the instructions for a trait. Genetic Diversity: ↑ The overall diversity in the DNA between the individuals of a species. Mutation: ↑ A change in an organism's DNA. This can be a change of a single letter or a much bigger change of hundreds of letters at once.
GENETIC DIVERSITY OF SPOTTED TURTLE (CLEMMYS GUTTATA) POPULATIONS IN A FRAGMENTED LANDSCAPE. Download (913.66 kB) + Collect. thesis. posted on 2019-10-16, 10:18 authored by Tyler J Scoville. Turtle species are facing losses to genetic variation caused by habitat fragmentation and large-scale landscape changes caused by humans.
We hereby certify that we have read and evaluated this thesis entitled "Genetic Diversity Among Bread Wheat (Triticum aestivum L.) Genotypes for Drought Tolerance in Eastern Bale, Ethiopia" prepared under our guidance by Kasahun Tadese. We recommend that it be submitted as fulfilling the thesis requirement.
crucial for the survival of a breed. A decline in genetic diversity can result in a higher disease susceptibility and a reduction of production traits. A closer examination of the term "genetic diversity" is conducted in Chapter 2.2 of this thesis and further specifications regarding equine genetic diversity are included as well.
2.6. Genetic Diversity in Sorghum 11 2.7. Genetic Variability Components 13 2.7.1. Genotypic and phenotypic variability 13 2.7.2. Phenotypic and genotypic coefficients of variability 14 2.8. Heritability and Genetic Advance 14 2.9. Correlation Coefficient 15 2.10. Path Coefficient Analysis 17 2.11. Genetic Distance and Clustering 18
coordinates accounted for 29% of the genetic variation. Generally, the present studies showed the existence of low genetic diversity among the released varieties which calls for timely action to widen the genetic diversity of Ethiopian released tef varieties. Moreover, both marker systems
The thesis has been submitted for examination with our approval as University supervisors Signed Date 4 / 10 / 11 Professor Patrick R. Rubaihayo Department of Agricultural Production, College of Agricultural and Environmental Sciences, Makerere ... Genetic Diversity in White- and Orange-fleshed Sweetpotato Farmer Varieties from
Gudeta Dida Hordofa have read and evaluated his thesis entitled "Genetic Diversity of Ethiopian Coffee (Coffea arabica L.) Collection Using SSR Marker" and examined the candidate. This is therefore to certify that the thesis has been accepted in partial fulfillment of the requirements for the degree of Master of Science in Plant Biotechnology.
f all cereal crops and feeds more than half of the world's population. Assessment of genetic diversity is of utmost important in rice breedin. from the perspective of selection, conservation and proper utilization. The present study was undertaken with an objective to asses. the genetic diversity among 28 rice cultivars by use of 22 SSR ma.
Citation: Temesgen Begna, Hayilu Gichile and Werkissa Yali (2022) Genetic Diversity and Its Impact in Enhancement of Crop Plants, Global Journal of Agricultural Research , Vol.10, No.2, pp.13-25 Plant breeding is a science that focuses on the development of new plant varieties in a systematic and ongoing method. It takes advantage of genetic variation among … Genetic Diversity and Its Impact ...
Genetic discrimination is an evolving phenomenon that impacts fundamental human rights such as dignity, justice and equity. Although, in the past, various definitions to better conceptualize ...
It lacks global genetic diversity as it is based on a small sample of U.S. donors, it fails to include many common sequencing variations, and it does not accurately reflect haplotype configurations from different populations. ... TThis thesis sought to develop and validate pipelines that integrate these advanced technologies to study regions ...
The few animals that founded the island had very little genetic diversity, and Dr. Dalén and his colleagues found that the level stayed low for the next 6,000 years.