- Scoping Review
- Open access
- Published: 14 November 2021

Effectiveness and safety of SARS-CoV-2 vaccine in real-world studies: a systematic review and meta-analysis
- Qiao Liu 1 na1 ,
- Chenyuan Qin 1 , 2 na1 ,
- Min Liu 1 &
- Jue Liu ORCID: orcid.org/0000-0002-1938-9365 1 , 2
Infectious Diseases of Poverty volume 10 , Article number: 132 ( 2021 ) Cite this article
57k Accesses
212 Citations
372 Altmetric
Metrics details
To date, coronavirus disease 2019 (COVID-19) becomes increasingly fierce due to the emergence of variants. Rapid herd immunity through vaccination is needed to block the mutation and prevent the emergence of variants that can completely escape the immune surveillance. We aimed to systematically evaluate the effectiveness and safety of COVID-19 vaccines in the real world and to establish a reliable evidence-based basis for the actual protective effect of the COVID-19 vaccines, especially in the ensuing waves of infections dominated by variants.
We searched PubMed, Embase and Web of Science from inception to July 22, 2021. Observational studies that examined the effectiveness and safety of SARS-CoV-2 vaccines among people vaccinated were included. Random-effects or fixed-effects models were used to estimate the pooled vaccine effectiveness (VE) and incidence rate of adverse events after vaccination, and their 95% confidence intervals ( CI ).
A total of 58 studies (32 studies for vaccine effectiveness and 26 studies for vaccine safety) were included. A single dose of vaccines was 41% (95% CI : 28–54%) effective at preventing SARS-CoV-2 infections, 52% (31–73%) for symptomatic COVID-19, 66% (50–81%) for hospitalization, 45% (42–49%) for Intensive Care Unit (ICU) admissions, and 53% (15–91%) for COVID-19-related death; and two doses were 85% (81–89%) effective at preventing SARS-CoV-2 infections, 97% (97–98%) for symptomatic COVID-19, 93% (89–96%) for hospitalization, 96% (93–98%) for ICU admissions, and 95% (92–98%) effective for COVID-19-related death, respectively. The pooled VE was 85% (80–91%) for the prevention of Alpha variant of SARS-CoV-2 infections, 75% (71–79%) for the Beta variant, 54% (35–74%) for the Gamma variant, and 74% (62–85%) for the Delta variant. The overall pooled incidence rate was 1.5% (1.4–1.6%) for adverse events, 0.4 (0.2–0.5) per 10 000 for severe adverse events, and 0.1 (0.1–0.2) per 10 000 for death after vaccination.
Conclusions
SARS-CoV-2 vaccines have reassuring safety and could effectively reduce the death, severe cases, symptomatic cases, and infections resulting from SARS-CoV-2 across the world. In the context of global pandemic and the continuous emergence of SARS-CoV-2 variants, accelerating vaccination and improving vaccination coverage is still the most important and urgent matter, and it is also the final means to end the pandemic.
Graphical Abstract
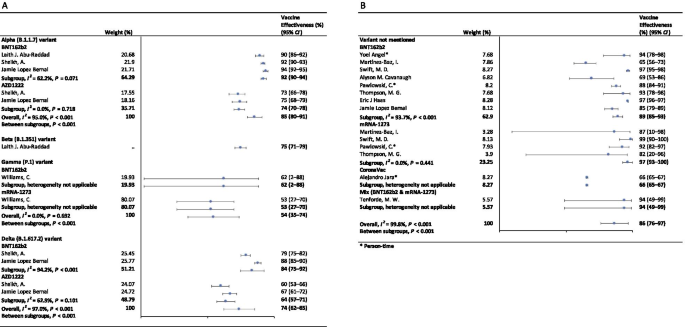
Since its outbreak, coronavirus disease 2019 (COVID-19) has spread rapidly, with a sharp rise in the accumulative number of infections worldwide. As of August 8, 2021, COVID-19 has already killed more than 4.2 million people and more than 203 million people were infected [ 1 ]. Given its alarming-spreading speed and the high cost of completely relying on non-pharmaceutical measures, we urgently need safe and effective vaccines to cover susceptible populations and restore people’s lives into the original [ 2 ].
According to global statistics, as of August 2, 2021, there are 326 candidate vaccines, 103 of which are in clinical trials, and 19 vaccines have been put into normal use, including 8 inactivated vaccines and 5 protein subunit vaccines, 2 RNA vaccines, as well as 4 non-replicating viral vector vaccines [ 3 ]. Our World in Data simultaneously reported that 27.3% of the world population has received at least one dose of a COVID-19 vaccine, and 13.8% is fully vaccinated [ 4 ].
To date, COVID-19 become increasingly fierce due to the emergence of variants [ 5 , 6 , 7 ]. Rapid herd immunity through vaccination is needed to block the mutation and prevent the emergence of variants that can completely escape the immune surveillance [ 6 , 8 ]. Several reviews systematically evaluated the effectiveness and/or safety of the three mainstream vaccines on the market (inactivated virus vaccines, RNA vaccines and viral vector vaccines) based on random clinical trials (RCT) yet [ 9 , 10 , 11 , 12 , 13 ].
In general, RNA vaccines are the most effective, followed by viral vector vaccines and inactivated virus vaccines [ 10 , 11 , 12 , 13 ]. The current safety of COVID-19 vaccines is acceptable for mass vaccination, but long-term monitoring of vaccine safety is needed, especially in older people with underlying conditions [ 9 , 10 , 11 , 12 , 13 ]. Inactivated vaccines had the lowest incidence of adverse events and the safety comparisons between mRNA vaccines and viral vectors were controversial [ 9 , 10 ].
RCTs usually conduct under a very demanding research circumstance, and tend to be highly consistent and limited in terms of population characteristics and experimental conditions. Actually, real-world studies differ significantly from RCTs in terms of study conditions and mass vaccination in real world requires taking into account factors, which are far more complex, such as widely heterogeneous populations, vaccine supply, willingness, medical accessibility, etc. Therefore, the real safety and effectiveness of vaccines turn out to be a major concern of international community. The results of a mass vaccination of CoronaVac in Chile demonstrated a protective effectiveness of 65.9% against the onset of COVID-19 after complete vaccination procedures [ 14 ], while the outcomes of phase 3 trials in Brazil and Turkey were 50.7% and 91.3%, reported on Sinovac’s website [ 14 ]. As for the Delta variant, the British claimed 88% protection after two doses of BNT162b2, compared with 67% for AZD1222 [ 15 ]. What is surprising is that the protection of BNT162b2 against infection in Israel is only 39% [ 16 ]. Several studies reported the effectiveness and safety of the COVID-19 vaccine in the real world recently, but the results remain controversial [ 17 , 18 , 19 , 20 ]. A comprehensive meta-analysis based upon the real-world studies is still in an urgent demand, especially for evaluating the effect of vaccines on variation strains. In the present study, we aimed to systematically evaluate the effectiveness and safety of the COVID-19 vaccine in the real world and to establish a reliable evidence-based basis for the actual protective effect of the COVID-19 vaccines, especially in the ensuing waves of infections dominated by variants.
Search strategy and selection criteria
Our methods were described in detail in our published protocol [PROSPERO (Prospective register of systematic reviews) registration, CRD42021267110]. We searched eligible studies published by 22 July 2021, from three databases including PubMed, Embase and Web of Science by the following search terms: (effectiveness OR safety) AND (COVID-19 OR coronavirus OR SARS-CoV-2) AND (vaccine OR vaccination). We used EndNoteX9.0 (Thomson ResearchSoft, Stanford, USA) to manage records, screen and exclude duplicates. This study was strictly performed according to the Preferred Reporting Items for Systematic Reviews and Meta-Analyses (PRISMA).
We included observational studies that examined the effectiveness and safety of severe acute respiratory syndrome coronavirus 2 (SARS-CoV-2) vaccines among people vaccinated with SARS-CoV-2 vaccines. The following studies were excluded: (1) irrelevant to the subject of the meta-analysis, such as studies that did not use SARS-CoV-2 vaccination as the exposure; (2) insufficient data to calculate the rate for the prevention of COVID-19, the prevention of hospitalization, the prevention of admission to the ICU, the prevention of COVID-19-related death, or adverse events after vaccination; (3) duplicate studies or overlapping participants; (4) RCT studies, reviews, editorials, conference papers, case reports or animal experiments; and (5) studies that did not clarify the identification of COVID-19.
Studies were identified by two investigators (LQ and QCY) independently following the criteria above, while discrepancies reconciled by a third investigator (LJ).
Data extraction and quality assessment
The primary outcome was the effectiveness of SARS-CoV-2 vaccines. The following data were extracted independently by two investigators (LQ and QCY) from the selected studies: (1) basic information of the studies, including first author, publication year and study design; (2) characteristics of the study population, including sample sizes, age groups, setting or locations; (3) kinds of the SARS-CoV-2 vaccines; (4) outcomes for the effectiveness of SARS-CoV-2 vaccines: the number of laboratory-confirmed COVID-19, hospitalization for COVID-19, admission to the ICU for COVID-19, and COVID-19-related death; and (5) outcomes for the safety of SARS-CoV-2 vaccines: the number of adverse events after vaccination.
We evaluated the risk of bias using the Newcastle–Ottawa quality assessment scale for cohort studies and case–control studies [ 21 ]. and assess the methodological quality using the checklist recommended by Agency for Healthcare Research and Quality (AHRQ) [ 22 ]. Cohort studies and case–control studies were classified as having low (≥ 7 stars), moderate (5–6 stars), and high risk of bias (≤ 4 stars) with an overall quality score of 9 stars. For cross-sectional studies, we assigned each item of the AHRQ checklist a score of 1 (answered “yes”) or 0 (answered “no” or “unclear”), and summarized scores across items to generate an overall quality score that ranged from 0 to 11. Low, moderate, and high risk of bias were identified as having a score of 8–11, 4–7 and 0–3, respectively.
Two investigators (LQ and QCY) independently assessed study quality, with disagreements resolved by a third investigator (LJ).
Data synthesis and statistical analysis
We performed a meta-analysis to pool data from included studies and assess the effectiveness and safety of SARS-CoV-2 vaccines by clinical outcomes (rates of the prevention of COVID-19, the prevention of hospitalization, the prevention of admission to the ICU, the prevention of COVID-19-related death, and adverse events after vaccination). Random-effects or fixed-effects models were used to pool the rates and adjusted estimates across studies separately, based on the heterogeneity between estimates ( I 2 ). Fixed-effects models were used if I 2 ≤ 50%, which represented low to moderate heterogeneity and random-effects models were used if I 2 > 50%, representing substantial heterogeneity.
We conducted subgroup analyses to investigate the possible sources of heterogeneity by using vaccine kinds, vaccination status, sample size, and study population as grouping variables. We used the Q test to conduct subgroup comparisons and variables were considered significant between subgroups if the subgroup difference P value was less than 0.05. Publication bias was assessed by funnel plot and Egger’s regression test. We analyzed data using Stata version 16.0 (StataCorp, Texas, USA).
A total of 4844 records were searched from the three databases. 2484 duplicates were excluded. After reading titles and abstracts, we excluded 2264 reviews, RCT studies, duplicates and other studies meeting our exclude criteria. Among the 96 studies under full-text review, 41 studies were excluded (Fig. 1 ). Ultimately, with three grey literatures included, this final meta-analysis comprised 58 eligible studies, including 32 studies [ 14 , 15 , 17 , 18 , 19 , 20 , 23 , 24 , 25 , 26 , 27 , 28 , 29 , 30 , 31 , 32 , 33 , 34 , 35 , 36 , 37 , 38 , 39 , 40 , 41 , 42 , 43 , 44 , 45 , 46 , 47 , 48 ] for vaccine effectiveness and 26 studies [ 49 , 50 , 51 , 52 , 53 , 54 , 55 , 56 , 57 , 58 , 59 , 60 , 61 , 62 , 63 , 64 , 65 , 66 , 67 , 68 , 69 , 70 , 71 , 72 , 73 , 74 ] for vaccine safety. Characteristics of included studies are showed in Additional file 1 : Table S1, Additional file 2 : Table S2. The risk of bias of all studies we included was moderate or low.
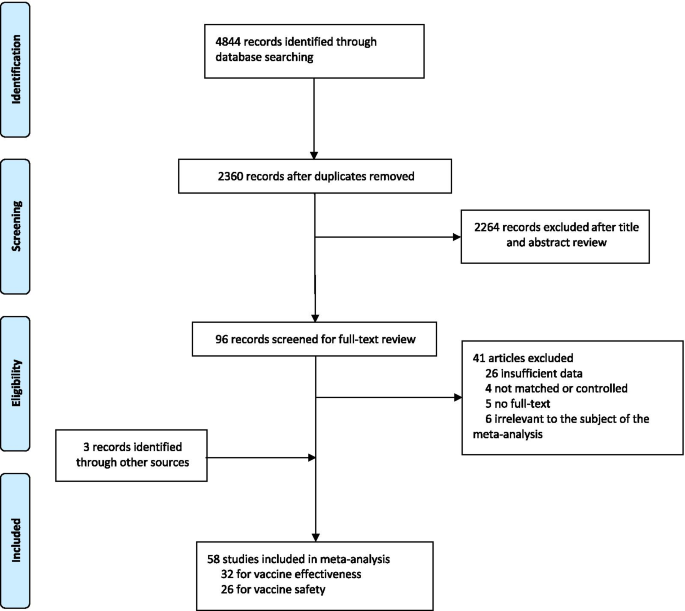
Flowchart of the study selection
Vaccine effectiveness for different clinical outcomes of COVID-19
We separately reported the vaccine effectiveness (VE) by the first and second dose of vaccines, and conducted subgroup analysis by the days after the first or second dose (< 7 days, ≥ 7 days, ≥ 14 days, and ≥ 21 days; studies with no specific days were classified as 1 dose, 2 dose or ≥ 1 dose).
For the first dose of SARS-CoV-2 vaccines, the pooled VE was 41% (95% CI : 28–54%) for the prevention of SARS-CoV-2 infection, 52% (95% CI : 31–73%) for the prevention of symptomatic COVID-19, 66% (95% CI : 50–81%) for the prevention of hospital admissions, 45% (95% CI : 42–49%) for the prevention of ICU admissions, and 53% (95% CI : 15–91%) for the prevention of COVID-19-related death (Table 1 ). The subgroup, ≥ 21 days after the first dose, was found to have the highest VE in each clinical outcome of COVID-19, regardless of ≥ 1 dose group (Table 1 ).
For the second dose of SARS-CoV-2 vaccines, the pooled VE was 85% (95% CI : 81–89%) for the prevention of SARS-CoV-2 infection, 97% (95% CI : 97–98%) for the prevention of symptomatic COVID-19, 93% (95% CI: 89–96%) for the prevention of hospital admissions, 96% (95% CI : 93–98%) for the prevention of ICU admissions, and 95% (95% CI : 92–98%) for the prevention of COVID-19-related death (Table 1 ). VE was 94% (95% CI : 78–98%) in ≥ 21 days after the second dose for the prevention of SARS-CoV-2 infection, higher than other subgroups, regardless of 2 dose group (Table 1 ). For the prevention of symptomatic COVID-19, VE was also relatively higher in 21 days after the second dose (99%, 95% CI : 94–100%). Subgroups showed no statistically significant differences in the prevention of hospital admissions, ICU admissions and COVID-19-related death (subgroup difference P values were 0.991, 0.414, and 0.851, respectively).
Vaccine effectiveness for different variants of SARS-CoV-2 in fully vaccinated people
In the fully vaccinated groups (over 14 days after the second dose), the pooled VE was 85% (95% CI: 80–91%) for the prevention of Alpha variant of SARS-CoV-2 infection, 54% (95% CI : 35–74%) for the Gamma variant, and 74% (95% CI : 62–85%) for the Delta variant. There was only one study [ 23 ] focused on the Beta variant, which showed the VE was 75% (95% CI : 71–79%) for the prevention of the Beta variant of SARS-CoV-2 infection. BNT162b2 vaccine had the highest VE in each variant group; 92% (95% CI : 90–94%) for the Alpha variant, 62% (95% CI : 2–88%) for the Gamma variant, and 84% (95% CI : 75–92%) for the Delta variant (Fig. 2 ).
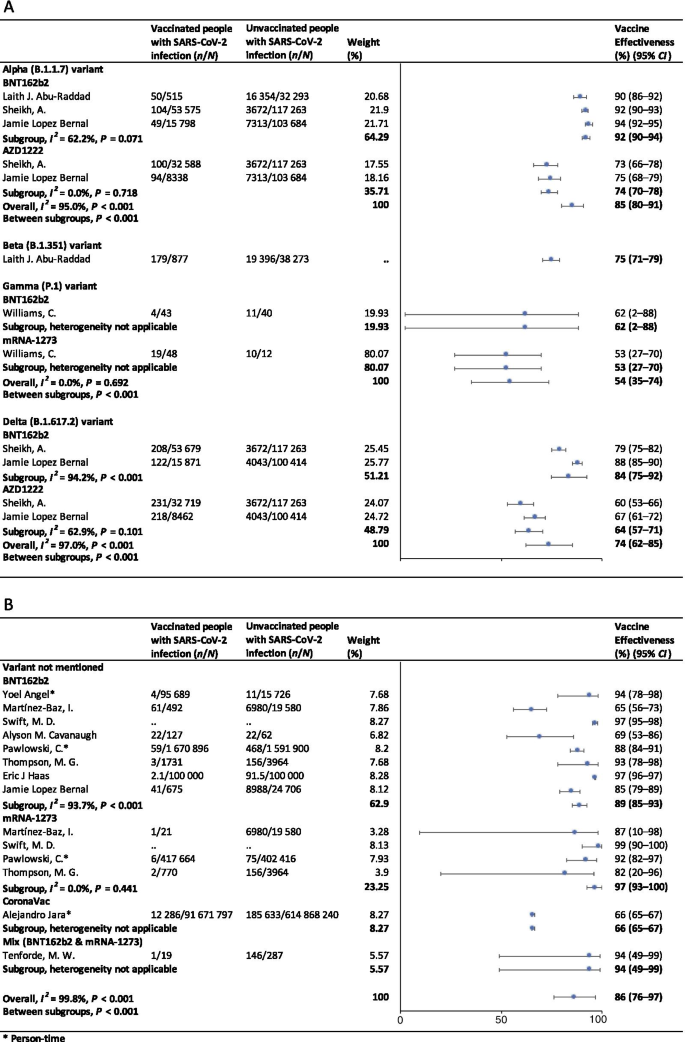
Forest plots for the vaccine effectiveness of SARS-CoV-2 vaccines in fully vaccinated populations. A Vaccine effectiveness against SARS-CoV-2 variants; B Vaccine effectiveness against SARS-CoV-2 with variants not mentioned. SARS-CoV-2 severe acute respiratory syndrome coronavirus 2, COVID-19 coronavirus disease 2019, CI confidence interval
For studies which had not mentioned the variant of SARS-CoV-2, the pooled VE was 86% (95% CI: 76–97%) for the prevention of SARS-CoV-2 infection in fully vaccinated people. mRNA-1273 vaccine had the highest pooled VE (97%, 95% CI: 93–100%, Fig. 2 ).
Safety of SARS-CoV-2 vaccines
As Table 2 showed, the incidence rate of adverse events varied widely among different studies. We conducted subgroup analysis by study population (general population, patients and healthcare workers), vaccine type (BNT162b2, mRNA-1273, CoronaVac, and et al.), and population size (< 1000, 1000–10 000, 10 000–100 000, and > 100 000). The overall pooled incidence rate was 1.5% (95% CI : 1.4–1.6%) for adverse events, 0.4 (95% CI : 0.2–0.5) per 10 000 for severe adverse events, and 0.1 (95% CI : 0.1–0.2) per 10 000 for death after vaccination. Incidence rate of adverse events was higher in healthcare workers (53.2%, 95% CI : 28.4–77.9%), AZD1222 vaccine group (79.6%, 95% CI : 60.8–98.3%), and < 1000 population size group (57.6%, 95% CI : 47.9–67.4%). Incidence rate of sever adverse events was higher in healthcare workers (127.2, 95% CI : 62.7–191.8, per 10 000), Gam-COVID-Vac vaccine group (175.7, 95% CI : 77.2–274.2, per 10 000), and 1000–10 000 population size group (336.6, 95% CI : 41.4–631.8, per 10 000). Incidence rate of death after vaccination was higher in patients (7.6, 95% CI : 0.0–32.2, per 10 000), BNT162b2 vaccine group (29.8, 95% CI : 0.0–71.2, per 10 000), and < 1000 population size group (29.8, 95% CI : 0.0–71.2, per 10 000). Subgroups of general population, vaccine type not mentioned, and > 100 000 population size had the lowest incidence rate of adverse events, severe adverse events, and death after vaccination.
Sensitivity analysis and publication bias
In the sensitivity analyses, VE for SARS-CoV-2 infections, symptomatic COVID-19 and COVID-19-related death got relatively lower when omitting over a single dose group of Maria et al.’s work [ 33 ]; when omitting ≥ 14 days after the first dose group and ≥ 14 days after the second dose group of Alejandro et al.’s work [ 14 ], VE for SARS-CoV-2 infections, hospitalization, ICU admission and COVID-19-related death got relatively higher; and VE for all clinical status of COVID-19 became lower when omitting ≥ 14 days after the second dose group of Eric et al.’s work [ 34 ]. Incidence rate of adverse events and severe adverse events got relatively higher when omitting China CDC’s data [ 74 ]. P values of Egger’s regression test for all the meta-analysis were more than 0.05, indicating that there might not be publication bias.
To our knowledge, this is a comprehensive systematic review and meta-analysis assessing the effectiveness and safety of SARS-CoV-2 vaccines based on real-world studies, reporting pooled VE for different variants of SARS-CoV-2 and incidence rate of adverse events. This meta-analysis comprised a total of 58 studies, including 32 studies for vaccine effectiveness and 26 studies for vaccine safety. We found that a single dose of SARS-CoV-2 vaccines was about 40–60% effective at preventing any clinical status of COVID-19 and that two doses were 85% or more effective. Although vaccines were not as effective against variants of SARS-CoV-2 as original virus, the vaccine effectiveness was still over 50% for fully vaccinated people. Normal adverse events were common, while the incidence of severe adverse events or even death was very low, providing reassurance to health care providers and to vaccine recipients and promote confidence in the safety of COVID-19 vaccines. Our findings strengthen and augment evidence from previous review [ 75 ], which confirmed the effectiveness of the BNT162b2 mRNA vaccine, and additionally reported the safety of SARS-CoV-2 vaccines, giving insight on the future of SARS-CoV-2 vaccine schedules.
Although most vaccines for the prevention of COVID-19 are two-dose vaccines, we found that the pooled VE of a single dose of SARS-CoV-2 vaccines was about 50%. Recent study showed that the T cell and antibody responses induced by a single dose of the BNT162b2 vaccine were comparable to those naturally infected with SARE-CoV-2 within weeks or months after infection [ 76 ]. Our findings could help to develop vaccination strategies under certain circumstances such as countries having a shortage of vaccines. In some countries, in order to administer the first dose to a larger population, the second dose was delayed for up to 12 weeks [ 77 ]. Some countries such as Canada had even decided to delay the second dose for 16 weeks [ 78 ]. However, due to a suboptimum immune response in those receiving only a single dose of a vaccine, such an approach had a chance to give rise to the emergence of variants of SARS-CoV-2 [ 79 ]. There remains a need for large clinical trials to assess the efficacy of a single-dose administration of two-dose vaccines and the risk of increasing the emergence of variants.
Two doses of SARS-CoV-2 vaccines were highly effective at preventing hospitalization, severe cases and deaths resulting from COVID-19, while the VE of different groups of days from the second vaccine dose showed no statistically significant differences. Our findings emphasized the importance of getting fully vaccinated, for the fact that most breakthrough infections were mild or asymptomatic. A recent study showed that the occurrence of breakthrough infections with SARS-CoV-2 in fully vaccinated populations was predictable with neutralizing antibody titers during the peri-infection period [ 80 ]. We also found getting fully vaccinated was at least 50% effective at preventing SARS-CoV-2 variants infections, despite reduced effectiveness compared with original virus; and BNT162b2 vaccine was found to have the highest VE in each variant group. Studies showed that the highly mutated variants were indicative of a form of rapid, multistage evolutionary jumps, which could preferentially occur in the milieu of partial immune control [ 81 , 82 ]. Therefore, immunocompromised patients should be prioritized for anti-COVID-19 immunization to mitigate persistent SARS-CoV-2 infections, during which multimutational SARS-CoV-2 variants could arise [ 83 ].
Recently, many countries, including Israel, the United States, China and the United Kingdom, have introduced a booster of COVID-19 vaccine, namely the third dose [ 84 , 85 , 86 , 87 ]. A study of Israel showed that among people vaccinated with BNT162b2 vaccine over 60 years, the risk of COVID-19 infection and severe illness in the non-booster group was 11.3 times (95% CI: 10.4–12.3) and 19.5 times (95% CI: 12.9–29.5) than the booster group, respectively [ 84 ]. Some studies have found that the third dose of Moderna, Pfizer-BioNTech, Oxford-AstraZeneca and Sinovac produced a spike in infection-blocking neutralizing antibodies when given a few months after the second dose [ 85 , 87 , 88 ]. In addition, the common adverse events associated with the third dose did not differ significantly from the symptoms of the first two doses, ranging from mild to moderate [ 85 ]. The overall incidence rate of local and systemic adverse events was 69% (57/97) and 20% (19/97) after receiving the third dose of BNT162b2 vaccine, respectively [ 88 ]. Results of a phase 3 clinical trial involving 306 people aged 18–55 years showed that adverse events after receiving a third dose of BNT162b2 vaccine (5–8 months after completion of two doses) were similar to those reported after receiving a second dose [ 85 ]. Based on V-safe, local reactions were more frequently after dose 3 (5323/6283; 84.7%) than dose 2 (5249/6283; 83.5%) among people who received 3 doses of Moderna. Systemic reactions were reported less frequently after dose 3 (4963/6283; 79.0%) than dose 2 (5105/6283; 81.3%) [ 86 ]. On August 4, WHO called for a halt to booster shots until at least the end of September to achieve an even distribution of the vaccine [ 89 ]. At this stage, the most important thing we should be thinking about is how to reach a global cover of people at risk with the first or second dose, rather than focusing on the third dose.
Based on real world studies, our results preliminarily showed that complete inoculation of COVID-19 vaccines was still effective against infection of variants, although the VE was generally diminished compared with the original virus. Particularly, the pooled VE was 54% (95% CI : 35–74%) for the Gamma variant, and 74% (95% CI : 62–85%) for the Delta variant. Since the wide spread of COVID-19, a number of variants have drawn extensive attention of international community, including Alpha variant (B.1.1.7), first identified in the United Kingdom; Beta variant (B.1.351) in South Africa; Gamma variant (P.1), initially appeared in Brazil; and the most infectious one to date, Delta variant (B.1.617.2) [ 90 ]. Israel recently reported a breakthrough infection of SARS-CoV-2, dominated by variant B.1.1.7 in a small number of fully vaccinated health care workers, raising concerns about the effectiveness of the original vaccine against those variants [ 80 ]. According to an observational cohort study in Qatar, VE of the BNT162b2 vaccine against the Alpha (B.1.1.7) and Beta (B.1.351) variants was 87% (95% CI : 81.8–90.7%) and 75.0% (95% CI : 70.5–7.9%), respectively [ 23 ]. Based on the National Immunization Management System of England, results from a recent real-world study of all the general population showed that the AZD1222 and BNT162b2 vaccines protected against symptomatic SARS-CoV-2 infection of Alpha variant with 74.5% (95% CI : 68.4–79.4%) and 93.7% (95% CI : 91.6–95.3%) [ 15 ]. In contrast, the VE against the Delta variant was 67.0% (95% CI : 61.3–71.8%) for two doses of AZD1222 vaccine and 88% (95% CI : 85.3–90.1%) for BNT162b2 vaccine [ 15 ].
In terms of adverse events after vaccination, the pooled incidence rate was very low, only 1.5% (95% CI : 1.4–1.6%). However, the prevalence of adverse events reported in large population (population size > 100 000) was much lower than that in small to medium population size. On the one hand, the vaccination population in the small to medium scale studies we included were mostly composed by health care workers, patients with specific diseases or the elderly. And these people are more concerned about their health and more sensitive to changes of themselves. But it remains to be proved whether patients or the elderly are more likely to have adverse events than the general. Mainstream vaccines currently on the market have maintained robust safety in specific populations such as cancer patients, organ transplant recipients, patients with rheumatic and musculoskeletal diseases, pregnant women and the elderly [ 54 , 91 , 92 , 93 , 94 ]. A prospective study by Tal Goshen-lag suggests that the safety of BNT162b2 vaccine in cancer patients is consistent with those previous reports [ 91 ]. In addition, the incidence rate of adverse events reported in the heart–lung transplant population is even lower than that in general population [ 95 ]. On the other hand, large scale studies at the national level are mostly based on national electronic health records or adverse event reporting systems, and it is likely that most mild or moderate symptoms are actually not reported.
Compared with the usual local adverse events (such as pain at the injection site, redness at the injection site, etc.) and normal systemic reactions (such as fatigue, myalgia, etc.), serious and life-threatening adverse events were rare due to our results. A meta-analysis based on RCTs only showed three cases of anaphylactic shock among 58 889 COVID-19 vaccine recipients and one in the placebo group [ 11 ]. The exact mechanisms underlying most of the adverse events are still unclear, accordingly we cannot establish a causal relation between severe adverse events and vaccination directly based on observational studies. In general, varying degrees of adverse events occur after different types of COVID-19 vaccination. Nevertheless, the benefits far outweigh the risks.
Our results showed the effectiveness and safety of different types of vaccines varied greatly. Regardless of SARS-CoV-2 variants, vaccine effectiveness varied from 66% (CoronaVac [ 14 ]) to 97% (mRNA-1273 [ 18 , 20 , 45 , 46 ]). The incidence rate of adverse events varied widely among different types of vaccines, which, however, could be explained by the sample size and population group of participants. BNT162b2, AZD1222, mRNA-1273 and CoronaVac were all found to have high vaccine efficacy and acceptable adverse-event profile in recent published studies [ 96 , 97 , 98 , 99 ]. A meta-analysis, focusing on the potential vaccine candidate which have reached to the phase 3 of clinical development, also found that although many of the vaccines caused more adverse events than the controls, most were mild, transient and manageable [ 100 ]. However, severe adverse events did occur, and there remains the need to implement a unified global surveillance system to monitor the adverse events of COVID-19 vaccines around the world [ 101 ]. A recent study employed a knowledge-based or rational strategy to perform a prioritization matrix of approved COVID-19 vaccines, and led to a scale with JANSSEN (Ad26.COV2.S) in the first place, and AZD1222, BNT162b2, and Sputnik V in second place, followed by BBIBP-CorV, CoronaVac and mRNA-1273 in third place [ 101 ]. Moreover, when deciding the priority of vaccines, the socioeconomic characteristics of each country should also be considered.
Our meta-analysis still has several limitations. First, we may include limited basic data on specific populations, as vaccination is slowly being promoted in populations under the age of 18 or over 60. Second, due to the limitation of the original real-world study, we did not conduct subgroup analysis based on more population characteristics, such as age. When analyzing the efficacy and safety of COVID-19 vaccine, we may have neglected the discussion on the heterogeneity from these sources. Third, most of the original studies only collected adverse events within 7 days after vaccination, which may limit the duration of follow-up for safety analysis.
Based on the real-world studies, SARS-CoV-2 vaccines have reassuring safety and could effectively reduce the death, severe cases, symptomatic cases, and infections resulting from SARS-CoV-2 across the world. In the context of global pandemic and the continuous emergence of SARS-CoV-2 variants, accelerating vaccination and improving vaccination coverage is still the most important and urgent matter, and it is also the final means to end the pandemic.
Availability of data and materials
All data generated or analyzed during this study are included in this published article and its additional information files.
Abbreviations
Coronavirus disease 2019
Severe Acute Respiratory Syndrome Coronavirus 2
Vaccine effectiveness
Confidence intervals
Intensive care unit
Random clinical trials
Preferred reporting items for systematic reviews and meta-analyses
COVID-19 Dashboard by the Center for Systems Science and Engineering (CSSE) at Johns Hopkins University (JHU). 2021. https://coronavirus.jhu.edu/map.html . Accessed 20 Aug 2021.
Barranco R, Rocca G, Molinelli A, Ventura F. Controversies and challenges of mass vaccination against SARS-CoV-2 in Italy: medico-legal perspectives and considerations. Healthcare (Basel). 2021. https://doi.org/10.3390/healthcare9091163 .
Article Google Scholar
COVID-19 vaccine tracker. 2021. https://vac-lshtm.shinyapps.io/ncov_vaccine_landscape/ . Accessed 20 Aug 2021.
Coronavirus (COVID-19) Vaccinations. 2021. https://ourworldindata.org/covid-vaccinations . Accessed 20 Aug 2021.
Kirby T. New variant of SARS-CoV-2 in UK causes surge of COVID-19. Lancet Respir Med. 2021;9(2):e20–1. https://doi.org/10.1016/s2213-2600(21)00005-9 .
Article CAS PubMed PubMed Central Google Scholar
Callaway E. Fast-spreading COVID variant can elude immune responses. Nature. 2021;589(7843):500–1. https://doi.org/10.1038/d41586-021-00121-z .
Article CAS PubMed Google Scholar
Reardon S. How the Delta variant achieves its ultrafast spread. Nature. 2021. https://doi.org/10.1038/d41586-021-01986-w .
Article PubMed Google Scholar
Li R, Liu J, Zhang H. The challenge of emerging SARS-CoV-2 mutants to vaccine development. J Genet Genomics. 2021;48(2):102–6. https://doi.org/10.1016/j.jgg.2021.03.001 .
Article PubMed PubMed Central Google Scholar
Chen M, Yuan Y, Zhou Y, Deng Z, Zhao J, Feng F, Zou H, Sun C. Safety of SARS-CoV-2 vaccines: a systematic review and meta-analysis of randomized controlled trials. Infect Dis Poverty. 2021;10(1):94. https://doi.org/10.1186/s40249-021-00878-5 .
Ling Y, Zhong J, Luo J. Safety and effectiveness of SARS-CoV-2 vaccines: a systematic review and meta-analysis. J Med Virol. 2021. https://doi.org/10.1002/jmv.27203 .
Pormohammad A, Zarei M, Ghorbani S, Mohammadi M, Razizadeh MH, Turner DL, Turner RJ. Efficacy and safety of COVID-19 vaccines: a systematic review and meta-analysis of randomized clinical trials. Vaccines (Basel). 2021. https://doi.org/10.3390/vaccines9050467 .
Sathian B, Asim M, Banerjee I, Roy B, Pizarro AB, Mancha MA, van Teijlingen ER, Kord-Varkaneh H, Mekkodathil AA, Subramanya SH, et al. Development and implementation of a potential coronavirus disease 2019 (COVID-19) vaccine: a systematic review and meta-analysis of vaccine clinical trials. Nepal J Epidemiol. 2021;11(1):959–82. https://doi.org/10.3126/nje.v11i1.36163 .
Yuan P, Ai P, Liu Y, Ai Z, Wang Y, Cao W, Xia X, Zheng JC. Safety, tolerability, and immunogenicity of COVID-19 vaccines: a systematic review and meta-analysis. medRxiv. 2020. https://doi.org/10.1101/2020.11.03.20224998 .
Jara A, Undurraga EA, González C, Paredes F, Fontecilla T, Jara G, Pizarro A, Acevedo J, Leo K, Leon F, et al. Effectiveness of an inactivated SARS-CoV-2 vaccine in Chile. N Engl J Med. 2021. https://doi.org/10.1056/NEJMoa2107715 .
Lopez Bernal J, Andrews N, Gower C, Gallagher E, Simmons R, Thelwall S, Stowe J, Tessier E, Groves N, Dabrera G, et al. Effectiveness of COVID-19 vaccines against the B.1.617.2 (Delta) variant. N Engl J Med. 2021. https://doi.org/10.1056/NEJMoa2108891 .
Israel says Pfizer Covid vaccine is just 39% effective as delta spreads, but still prevents severe illness. 2021. https://www.cnbc.com/2021/07/23/delta-variant-pfizer-covid-vaccine-39percent-effective-in-israel-prevents-severe-illness.html . Accessed 20 Aug 2021.
Zacay G, Shasha D, Bareket R, Kadim I, Hershkowitz Sikron F, Tsamir J, Mossinson D, Heymann AD. BNT162b2 vaccine effectiveness in preventing asymptomatic infection with SARS-CoV-2 virus: a nationwide historical cohort study. Open Forum Infect Dis. 2021;8(6): ofab262. https://doi.org/10.1093/ofid/ofab262 .
Martínez-Baz I, Miqueleiz A, Casado I, Navascués A, Trobajo-Sanmartín C, Burgui C, Guevara M, Ezpeleta C, Castilla J. Effectiveness of COVID-19 vaccines in preventing SARS-CoV-2 infection and hospitalisation, Navarre, Spain, January to April 2021. Eurosurveillance. 2021. https://doi.org/10.2807/1560-7917.Es.2021.26.21.2100438 .
Tenforde MW, Olson SM, Self WH, Talbot HK, Lindsell CJ, Steingrub JS, Shapiro NI, Ginde AA, Douin DJ, Prekker ME, et al. Effectiveness of Pfizer-BioNTech and moderna vaccines against COVID-19 among hospitalized adults aged ≥65 years—United States, January–March 2021. MMWR Morb Mortal Wkly Rep. 2021;70(18):674–9. https://doi.org/10.15585/mmwr.mm7018e1 .
Pawlowski C, Lenehan P, Puranik A, Agarwal V, Venkatakrishnan AJ, Niesen MJM, O’Horo JC, Virk A, Swift MD, Badley AD, et al. FDA-authorized mRNA COVID-19 vaccines are effective per real-world evidence synthesized across a multi-state health system. Med (N Y). 2021. https://doi.org/10.1016/j.medj.2021.06.007 .
Wells G, Shea B, O'Connell D, Peterson J, Welch V, Losos M, Tugwell P. The Newcastle-Ottawa Scale (NOS) for assessing the quality of nonrandomised studies in meta-analyses. http://www.ohri.ca/programs/clinical_epidemiology/oxford.asp . Accessed 20 Aug 2021.
Rostom A, Dubé C, Cranney A, et al. Celiac Disease. Rockville (MD): Agency for Healthcare Research and Quality (US); 2004 Sep. (Evidence Reports/Technology Assessments, No. 104.) Appendix D. Quality Assessment Forms. Available from: https://www.ncbi.nlm.nih.gov/books/NBK35156/ . Accessed 20 Aug 2021
Abu-Raddad LJ, Chemaitelly H, Butt AA. Effectiveness of the BNT162b2 COVID-19 vaccine against the B.1.1.7 and B.1.351 Variants. N Engl J Med. 2021;385(2):187–9. https://doi.org/10.1056/NEJMc2104974 .
Angel Y, Spitzer A, Henig O, Saiag E, Sprecher E, Padova H, Ben-Ami R. Association between vaccination with BNT162b2 and incidence of symptomatic and asymptomatic SARS-CoV-2 infections among health care workers. JAMA. 2021;325(24):2457–65. https://doi.org/10.1001/jama.2021.7152 .
Azamgarhi T, Hodgkinson M, Shah A, Skinner JA, Hauptmannova I, Briggs TWR, Warren S. BNT162b2 vaccine uptake and effectiveness in UK healthcare workers—a single centre cohort study. Nat Commun. 2021;12(1):3698. https://doi.org/10.1038/s41467-021-23927-x .
Bianchi FP, Germinario CA, Migliore G, Vimercati L, Martinelli A, Lobifaro A, Tafuri S, Stefanizzi P. BNT162b2 mRNA COVID-19 vaccine effectiveness in the prevention of SARS-CoV-2 infection: a preliminary report. J Infect Dis. 2021. https://doi.org/10.1093/infdis/jiab262 .
Britton A, Jacobs Slifka KM, Edens C, Nanduri SA, Bart SM, Shang N, Harizaj A, Armstrong J, Xu K, Ehrlich HY, et al. Effectiveness of the Pfizer-BioNTech COVID-19 vaccine among residents of two skilled nursing facilities experiencing COVID-19 outbreaks—Connecticut, December 2020–February 2021. MMWR Morb Mortal Wkly Rep. 2021;70(11):396–401. https://doi.org/10.15585/mmwr.mm7011e3 .
Cavanaugh AM, Fortier S, Lewis P, Arora V, Johnson M, George K, Tobias J, Lunn S, Miller T, Thoroughman D, et al. COVID-19 outbreak associated with a SARS-CoV-2 R1 lineage variant in a skilled nursing facility after vaccination program—Kentucky, March 2021. MMWR Morb Mortal Wkly Rep. 2021;70(17):639–43. https://doi.org/10.15585/mmwr.mm7017e2 .
Chemaitelly H, Yassine HM, Benslimane FM, Al Khatib HA, Tang P, Hasan MR, Malek JA, Coyle P, Ayoub HH, Al Kanaani Z, et al. mRNA-1273 COVID-19 vaccine effectiveness against the B.1.1.7 and B.1.351 variants and severe COVID-19 disease in Qatar. Nat Med. 2021. https://doi.org/10.1038/s41591-021-01446-y .
Chodick G, Tene L, Patalon T, Gazit S, Ben Tov A, Cohen D, Muhsen K. Assessment of effectiveness of 1 dose of BNT162b2 vaccine for SARS-CoV-2 infection 13 to 24 days after immunization. JAMA Netw Open. 2021;4(6): e2115985. https://doi.org/10.1001/jamanetworkopen.2021.15985 .
Chodick G, Tene L, Rotem RS, Patalon T, Gazit S, Ben-Tov A, Weil C, Goldshtein I, Twig G, Cohen D, et al. The effectiveness of the TWO-DOSE BNT162b2 vaccine: analysis of real-world data. Clin Infect Dis. 2021. https://doi.org/10.1093/cid/ciab438 .
Dagan N, Barda N, Kepten E, Miron O, Perchik S, Katz MA, Hernán MA, Lipsitch M, Reis B, Balicer RD. BNT162b2 mRNA COVID-19 vaccine in a nationwide mass vaccination setting. N Engl J Med. 2021;384(15):1412–23. https://doi.org/10.1056/NEJMoa2101765 .
Flacco ME, Soldato G, Acuti Martellucci C, Carota R, Di Luzio R, Caponetti A, Manzoli L. Interim estimates of COVID-19 vaccine effectiveness in a mass vaccination setting: data from an Italian Province. VacCInes (Basel). 2021. https://doi.org/10.3390/vaccines9060628 .
Haas EJ, Angulo FJ, McLaughlin JM, Anis E, Singer SR, Khan F, Brooks N, Smaja M, Mircus G, Pan K, et al. Impact and effectiveness of mRNA BNT162b2 vaccine against SARS-CoV-2 infections and COVID-19 cases, hospitalisations, and deaths following a nationwide vaccination campaign in Israel: an observational study using national surveillance data. Lancet. 2021;397(10287):1819–29. https://doi.org/10.1016/s0140-6736(21)00947-8 .
Hall VJ, Foulkes S, Saei A, Andrews N, Oguti B, Charlett A, Wellington E, Stowe J, Gillson N, Atti A, et al. COVID-19 vaccine coverage in health-care workers in England and effectiveness of BNT162b2 mRNA vaccine against infection (SIREN): a prospective, multicentre, cohort study. Lancet. 2021;397(10286):1725–35. https://doi.org/10.1016/s0140-6736(21)00790-x .
Hyams C, Marlow R, Maseko Z, King J, Ward L, Fox K, Heath R, Tuner A, Friedrich Z, Morrison L, et al. Effectiveness of BNT162b2 and ChAdOx1 nCoV-19 COVID-19 vaccination at preventing hospitalisations in people aged at least 80 years: a test-negative, case-control study. Lancet Infect Dis. 2021. https://doi.org/10.1016/s1473-3099(21)00330-3 .
Khan N, Mahmud N. Effectiveness of SARS-CoV-2 vaccination in a veterans affairs cohort of patients with inflammatory bowel disease with diverse exposure to immunosuppressive medications. Gastroenterology. 2021. https://doi.org/10.1053/j.gastro.2021.05.044 .
Knobel P, Serra C, Grau S, Ibañez R, Diaz P, Ferrández O, Villar R, Lopez AF, Pujolar N, Horcajada JP, et al. COVID-19 mRNA vaccine effectiveness in asymptomatic healthcare workers. Infect Control Hosp Epidemiol. 2021. https://doi.org/10.1017/ice.2021.287 .
Lopez Bernal J, Andrews N, Gower C, Robertson C, Stowe J, Tessier E, Simmons R, Cottrell S, Roberts R, O’Doherty M, et al. Effectiveness of the Pfizer-BioNTech and Oxford-AstraZeneca vaccines on covid-19 related symptoms, hospital admissions, and mortality in older adults in England: test negative case-control study. BMJ. 2021;373: n1088. https://doi.org/10.1136/bmj.n1088 .
Mazagatos C, Monge S, Olmedo C, Vega L, Gallego P, Martín-Merino E, Sierra MJ, Limia A, Larrauri A. Effectiveness of mRNA COVID-19 vaccines in preventing SARS-CoV-2 infections and COVID-19 hospitalisations and deaths in elderly long-term care facility residents, Spain, weeks 53, 2020 to 13 2021. Eurosurveillance. 2021. https://doi.org/10.2807/1560-7917.Es.2021.26.24.2100452 .
Pilishvili T, Fleming-Dutra KE, Farrar JL, Gierke R, Mohr NM, Talan DA, Krishnadasan A, Harland KK, Smithline HA, Hou PC, et al. Interim estimates of vaccine effectiveness of Pfizer-BioNTech and Moderna COVID-19 vaccines among health care personnel—33 US Sites, January–March 2021. MMWR Morb Mortal Wkly Rep. 2021;70(20):753–8. https://doi.org/10.15585/mmwr.mm7020e2 .
Sheikh A, McMenamin J, Taylor B, Robertson C. SARS-CoV-2 Delta VOC in Scotland: demographics, risk of hospital admission, and vaccine effectiveness. Lancet. 2021;397(10293):2461–2. https://doi.org/10.1016/s0140-6736(21)01358-1 .
Shrotri M, Krutikov M, Palmer T, Giddings R, Azmi B, Subbarao S, Fuller C, Irwin-Singer A, Davies D, Tut G, et al. Vaccine effectiveness of the first dose of ChAdOx1 nCoV-19 and BNT162b2 against SARS-CoV-2 infection in residents of long-term care facilities in England (VIVALDI): a prospective cohort study. Lancet Infect Dis. 2021. https://doi.org/10.1016/s1473-3099(21)00289-9 .
Skowronski DM, Setayeshgar S, Zou M, Prystajecky N, Tyson JR, Galanis E, Naus M, Patrick DM, Sbihi H, El Adam S, et al. Single-dose mRNA vaccine effectiveness against SARS-CoV-2, including Alpha and Gamma variants: a test-negative design in adults 70 years and older in British Columbia,Canada. Clin Infect Dis. 2021. https://doi.org/10.1093/cid/ciab616 .
Swift MD, Breeher LE, Tande AJ, Tommaso CP, Hainy CM, Chu H, Murad MH, Berbari EF, Virk A. Effectiveness of mRNA COVID-19 vaccines against SARS-CoV-2 infection in a cohort of healthcare personnel. Clin Infect Dis. 2021. https://doi.org/10.1093/cid/ciab361 .
Thompson MG, Burgess JL, Naleway AL, Tyner H, Yoon SK, Meece J, Olsho LEW, Caban-Martinez AJ, Fowlkes AL, Lutrick K, et al. Prevention and attenuation of COVID-19 with the BNT162b2 and mRNA-1273 Vaccines. N Engl J Med. 2021. https://doi.org/10.1056/NEJMoa2107058 .
Vasileiou E, Simpson CR, Shi T, Kerr S, Agrawal U, Akbari A, Bedston S, Beggs J, Bradley D, Chuter A, et al. Interim findings from first-dose mass COVID-19 vaccination roll-out and COVID-19 hospital admissions in Scotland: a national prospective cohort study. Lancet. 2021;397(10285):1646–57. https://doi.org/10.1016/s0140-6736(21)00677-2 .
Williams C, Al-Bargash D, Macalintal C, Stuart R, Seth A, Latham J, Gitterman L, Fedsin S, Godoy M, Kozak R, et al. COVID-19 outbreak associated with a SARS-CoV-2 P.1 lineage in a long-term care home after implementation of a vaccination program—Ontario, April–May 2021. Clin Infect Dis. 2021. https://doi.org/10.1093/cid/ciab617 .
Alhazmi A, Alamer E, Daws D, Hakami M, Darraj M, Abdelwahab S, Maghfuri A, Algaissi A. Evaluation of side effects associated with COVID-19 vaccines in Saudi Arabia. Vaccines (Basel). 2021. https://doi.org/10.3390/vaccines9060674 .
Andrzejczak-Grządko S, Czudy Z, Donderska M. Side effects after COVID-19 vaccinations among residents of Poland. Eur Rev Med Pharmacol Sci. 2021;25(12):4418–21. https://doi.org/10.26355/eurrev_202106_26153 .
Baldolli A, Michon J, Appia F, Galimard C, Verdon R, Parienti JJ. Tolerance of BNT162b2 mRNA COVI-19 vaccine in patients with a medical history of COVID-19 disease: a case control study. Vaccine. 2021;39(32):4410–3. https://doi.org/10.1016/j.vaccine.2021.06.054 .
Cherian S, Paul A, Ahmed S, Alias B, Manoj M, Santhosh AK, Varghese DR, Krishnan N, Shenoy P. Safety of the ChAdOx1 nCoV-19 and the BBV152 vaccines in 724 patients with rheumatic diseases: a post-vaccination cross-sectional survey. Rheumatol Int. 2021;41(8):1441–5. https://doi.org/10.1007/s00296-021-04917-0 .
Chevallier P, Coste-Burel M, Le Bourgeois A, Peterlin P, Garnier A, Béné MC, Imbert BM, Drumel T, Le Gouill S, Moreau P, et al. Safety and immunogenicity of a first dose of SARS-CoV-2 mRNA vaccine in allogeneic hematopoietic stem-cells recipients. EJHaem. 2021. https://doi.org/10.1002/jha2.242 .
Connolly CM, Ruddy JA, Boyarsky BJ, Avery RK, Werbel WA, Segev DL, Garonzik-Wang J, Paik JJ. Safety of the first dose of mRNA SARS-CoV-2 vaccines in patients with rheumatic and musculoskeletal diseases. Ann Rheum Dis. 2021. https://doi.org/10.1136/annrheumdis-2021-220231 .
Furer V, Eviatar T, Zisman D, Peleg H, Paran D, Levartovsky D, Zisapel M, Elalouf O, Kaufman I, Meidan R, et al. Immunogenicity and safety of the BNT162b2 mRNA COVID-19 vaccine in adult patients with autoimmune inflammatory rheumatic diseases and in the general population: a multicentre study. Ann Rheum Dis. 2021. https://doi.org/10.1136/annrheumdis-2021-220647 .
Gee J, Marquez P, Su J, Calvert GM, Liu R, Myers T, Nair N, Martin S, Clark T, Markowitz L, et al. First month of COVID-19 vaccine safety monitoring—United States, December 14, 2020–January 13, 2021. MMWR Morb Mortal Wkly Rep. 2021;70(8):283–8. https://doi.org/10.15585/mmwr.mm7008e3 .
Hashimoto T, Ozaki A, Bhandari D, Sawano T, Sah R, Tanimoto T. High anaphylaxis rates following vaccination with the Pfizer BNT162b2 mRNA vaccine against COVID-19 in Japanese health care workers; a secondary analysis of initial post-approval safety data. J Travel Med. 2021. https://doi.org/10.1093/jtm/taab090 .
Lv G, Yuan J, Xiong X, Li M. Mortality rate and characteristics of deaths following COVID-19 vaccination. Front Med (Lausanne). 2021;8: 670370. https://doi.org/10.3389/fmed.2021.670370 .
McMurry R, Lenehan P, Awasthi S, Silvert E, Puranik A, Pawlowski C, Venkatakrishnan AJ, Anand P, Agarwal V, O’Horo JC, et al. Real-time analysis of a mass vaccination effort confirms the safety of FDA-authorized mRNA COVID-19 vaccines. Med (N Y). 2021. https://doi.org/10.1016/j.medj.2021.06.006 .
Monin L, Laing AG, Muñoz-Ruiz M, McKenzie DR, Del Molino Del Barrio I, Alaguthurai T, Domingo-Vila C, Hayday TS, Graham C, Seow J, et al. Safety and immunogenicity of one versus two doses of the COVID-19 vaccine BNT162b2 for patients with cancer: interim analysis of a prospective observational study. Lancet Oncol. 2021;22(6):765–78. https://doi.org/10.1016/s1470-2045(21)00213-8 .
Pagotto V, Ferloni A, Mercedes Soriano M, Díaz M, Braguinsky Golde N, González MI, Asprea V, Staneloni MI, Zingoni P, Vidal G, et al. Active monitoring of early safety of Sputnik V vaccine in Buenos Aires, Argentina. MediCIna (B Aires). 2021;81(3):408–14.
Google Scholar
Peled Y, Ram E, Lavee J, Sternik L, Segev A, Wieder-Finesod A, Mandelboim M, Indenbaum V, Levy I, Raanani E, et al. BNT162b2 vaccination in heart transplant recipients: Clinical experience and antibody response. J Heart Lung Transplant. 2021. https://doi.org/10.1016/j.healun.2021.04.003 .
Quiroga B, Sánchez-Álvarez E, Goicoechea M, de Sequera P. COVID-19 vaccination among Spanish nephrologists: acceptance and side effects. J Healthc Qual Res. 2021. https://doi.org/10.1016/j.jhqr.2021.05.002 .
Ram R, Hagin D, Kikozashvilli N, Freund T, Amit O, Bar-On Y, Beyar-Katz O, Shefer G, Moshiashvili MM, Karni C, et al. Safety and immunogenicity of the BNT162b2 mRNA COVID-19 vaccine in patients after allogeneic HCT or CD19-based CART therapy—a single center prospective cohort study. Transplant Cell Ther. 2021. https://doi.org/10.1016/j.jtct.2021.06.024 .
Revon-Riviere G, Ninove L, Min V, Rome A, Coze C, Verschuur A, de Lamballerie X, André N. The BNT162b2 mRNA COVID-19 vaccine in adolescents and young adults with cancer: a monocentric experience. Eur J Cancer. 2021;154:30–4. https://doi.org/10.1016/j.ejca.2021.06.002 .
Riad A, Pokorná A, Mekhemar M, Conrad J, Klugarová J, Koščík M, Klugar M, Attia S. Safety of ChAdOx1 nCoV-19 vaccine: independent evidence from two EU states. Vaccines (Basel). 2021. https://doi.org/10.3390/vaccines9060673 .
Riad A, Sağıroğlu D, Üstün B, Pokorná A, Klugarová J, Attia S, Klugar M. Prevalence and risk factors of CoronaVac Side effects: an independent cross-sectional study among healthcare workers in Turkey. J Clin Med. 2021. https://doi.org/10.3390/jcm10122629 .
Rosman Y, Lavi N, Meir-Shafrir K, Lachover-Roth I, Cohen-Engler A, Mekori YA, Confino-Cohen R. Safety of BNT162b2 mRNA COVID-19 vaccine in patients with mast cell disorders. J Allergy Clin Immunol Pract. 2021. https://doi.org/10.1016/j.jaip.2021.06.032 .
Signorelli C, Odone A, Gianfredi V, Capraro M, Kacerik E, Chiecca G, Scardoni A, Minerva M, Mantecca R, Musarò P, et al. Application of the “immunization islands” model to improve quality, efficiency and safety of a COVID-19 mass vaccination site. Ann Ig. 2021;33(5):499–512. https://doi.org/10.7416/ai.2021.2456 .
Vallée A, Chan-Hew-Wai A, Bonan B, Lesprit P, Parquin F, Catherinot É, Choucair J, Billard D, Amiel-Taieb C, Camps È, et al. Oxford-AstraZeneca COVID-19 vaccine: need of a reasoned and effective vaccine campaign. Public Health. 2021;196:135–7. https://doi.org/10.1016/j.puhe.2021.05.030 .
Wang J, Hou Z, Liu J, Gu Y, Wu Y, Chen Z, Ji J, Diao S, Qiu Y, Zou S, et al. Safety and immunogenicity of COVID-19 vaccination in patients with non-alcoholic fatty liver disease (CHESS2101): a multicenter study. J Hepatol. 2021. https://doi.org/10.1016/j.jhep.2021.04.026 .
Zhang MX, Zhang TT, Shi GF, Cheng FM, Zheng YM, Tung TH, Chen HX. Safety of an inactivated SARS-CoV-2 vaccine among healthcare workers in China. Expert Rev Vaccines. 2021. https://doi.org/10.1080/14760584.2021.1925112 .
Shay DK, Gee J, Su JR, Myers TR, Marquez P, Liu R, Zhang B, Licata C, Clark TA, Shimabukuro TT. Safety monitoring of the Janssen (Johnson & Johnson) COVID-19 Vaccine—United States, March–April 2021. MMWR Morb Mortal Wkly Rep. 2021;70(18):680–4. https://doi.org/10.15585/mmwr.mm7018e2 .
Prevention CCfDCa. Information analysis of COVID-19 vaccine adverse reaction monitoring in China. 2021-5-28. http://www.chinacdc.cn/jkzt/ymyjz/ymyjjz_6758/202105/t20210528_230908.html . Accessed 20 Aug 2021.
Kow CS, Hasan SS. Real-world effectiveness of BNT162b2 mRNA vaccine: a meta-analysis of large observational studies. Inflammopharmacology. 2021;29(4):1075–90. https://doi.org/10.1007/s10787-021-00839-2 .
Angyal A, Longet S, Moore S, Payne RP, Harding A et al. T-Cell and Antibody Responses to First BNT162b2 Vaccine Dose in Previously SARS-CoV-2-Infected and Infection-Naive UK Healthcare Workers: A Multicentre, Prospective, Observational Cohort Study. Available at SSRN: https://ssrn.com/abstract=3820576 or https://doi.org/10.2139/ssrn.3820576 . Accessed 20 Aug 2021.
Pimenta D, Yates C, Pagel C, Gurdasani D. Delaying the second dose of covid-19 vaccines. BMJ. 2021;372: n710. https://doi.org/10.1136/bmj.n710 .
Tauh T, Mozel M, Meyler P, Lee SM. An updated look at the 16-week window between doses of vaccines in BC for COVID-19. BC Med J. 2021;63(3):102–3.
Kadire SR, Wachter RM, Lurie N. Delayed second dose versus standard regimen for COVID-19 vaccination. N Engl J Med. 2021;384(9): e28. https://doi.org/10.1056/NEJMclde2101987 .
Bergwerk M, Gonen T, Lustig Y, Amit S, Lipsitch M, Cohen C, Mandelboim M, Gal Levin E, Rubin C, Indenbaum V, et al. COVID-19 breakthrough infections in vaccinated health care workers. N Engl J Med. 2021. https://doi.org/10.1056/NEJMoa2109072 .
Truong TT, Ryutov A, Pandey U, Yee R, Goldberg L, Bhojwani D, Aguayo-Hiraldo P, Pinsky BA, Pekosz A, Shen L, et al. Persistent SARS-CoV-2 infection and increasing viral variants in children and young adults with impaired humoral immunity. medRxiv. 2021. https://doi.org/10.1101/2021.02.27.21252099 .
Choi B, Choudhary MC, Regan J, Sparks JA, Padera RF, Qiu X, Solomon IH, Kuo HH, Boucau J, Bowman K, et al. Persistence and evolution of SARS-CoV-2 in an Immunocompromised Host. N Engl J Med. 2020;383(23):2291–3. https://doi.org/10.1056/NEJMc2031364 .
Corey L, Beyrer C, Cohen MS, Michael NL, Bedford T, Rolland M. SARS-CoV-2 variants in patients with immunosuppression. N Engl J Med. 2021;385(6):562–6. https://doi.org/10.1056/NEJMsb2104756 .
Bar-On YM, Goldberg Y, Mandel M, Bodenheimer O, Freedman L, Kalkstein N, Mizrahi B, Alroy-Preis S, Ash N, Milo R, et al. Protection of BNT162b2 vaccine booster against Covid-19 in Israel. N Engl J Med. 2021;385(15):1393–400. https://doi.org/10.1056/NEJMoa2114255 .
Hause AM, Baggs J, Gee J, Marquez P, Myers TR, Shimabukuro TT, Shay DK. Safety monitoring of an additional dose of COVID-19 vaccine—United States, August 12–September 19, 2021. MMWR Morb Mortal Wkly Rep. 2021;70(39):1379–84. https://doi.org/10.15585/mmwr.mm7039e4 .
Furlow B. Immunocompromised patients in the USA and UK should receive third dose of COVID-19 vaccine. Lancet Rheumatol. 2021. https://doi.org/10.1016/s2665-9913(21)00313-1 .
Flaxman A, Marchevsky NG, Jenkin D, Aboagye J, Aley PK, Angus B, Belij-Rammerstorfer S, Bibi S, Bittaye M, Cappuccini F, et al. Reactogenicity and immunogenicity after a late second dose or a third dose of ChAdOx1 nCoV-19 in the UK: a substudy of two randomised controlled trials (COV001 and COV002). Lancet. 2021;398(10304):981–90. https://doi.org/10.1016/s0140-6736(21)01699-8 .
Peled Y, Ram E, Lavee J, Segev A, Matezki S, Wieder-Finesod A, Halperin R, Mandelboim M, Indenbaum V, Levy I, et al. Third dose of the BNT162b2 vaccine in heart transplant recipients: immunogenicity and clinical experience. J Heart Lung Transplant. 2021. https://doi.org/10.1016/j.healun.2021.08.010 .
WHO. WHO press conference on coronavirus disease (COVID-19)—4 August 2021. 2021. https://www.who.int/multi-media/details/who-press-conference-on-coronavirus-disease-(covid-19)---4-august-2021 . Accessed 20 Aug 2021.
Cascella M, Rajnik M, Aleem A, Dulebohn SC, Di Napoli R. Features, evaluation, and treatment of coronavirus (COVID-19). In: StatPearls. edn. Treasure Island (FL): StatPearls Publishing Copyright © 2021, StatPearls Publishing LLC.; 2021.
Goshen-Lago T, Waldhorn I, Holland R, Szwarcwort-Cohen M, Reiner-Benaim A, Shachor-Meyouhas Y, Hussein K, Fahoum L, Baruch M, Peer A, et al. Serologic status and toxic effects of the SARS-CoV-2 BNT162b2 vaccine in patients undergoing treatment for cancer. JAMA Oncol. 2021. https://doi.org/10.1001/jamaoncol.2021.2675 .
Ou MT, Boyarsky BJ, Motter JD, Greenberg RS, Teles AT, Ruddy JA, Krach MR, Jain VS, Werbel WA, Avery RK, et al. Safety and reactogenicity of 2 doses of SARS-CoV-2 vaccination in solid organ transplant recipients. Transplantation. 2021. https://doi.org/10.1097/tp.0000000000003780 .
Bookstein Peretz S, Regev N, Novick L, Nachshol M, Goffer E, Ben-David A, Asraf K, Doolman R, Sapir E, Regev Yochay G, et al. Short-term outcome of pregnant women vaccinated by BNT162b2 mRNA COVID-19 vaccine. Ultrasound Obstet Gynecol. 2021. https://doi.org/10.1002/uog.23729 .
Shimabukuro TT, Kim SY, Myers TR, Moro PL, Oduyebo T, Panagiotakopoulos L, Marquez PL, Olson CK, Liu R, Chang KT, et al. Preliminary findings of mRNA COVID-19 vaccine safety in pregnant persons. N Engl J Med. 2021;384(24):2273–82. https://doi.org/10.1056/NEJMoa2104983 .
Peled Y, Ram E, Lavee J, Sternik L, Segev A, Wieder-Finesod A, Mandelboim M, Indenbaum V, Levy I, Raanani E, et al. BNT162b2 vaccination in heart transplant recipients: clinical experience and antibody response. J Heart Lung Transplant. 2021;40(8):759–62. https://doi.org/10.1016/j.healun.2021.04.003 .
Thomas SJ, Moreira ED Jr, Kitchin N, Absalon J, Gurtman A, Lockhart S, Perez JL, Pérez Marc G, Polack FP, Zerbini C, et al. Safety and efficacy of the BNT162b2 mRNA COVID-19 vaccine through 6 months. N Engl J Med. 2021. https://doi.org/10.1056/NEJMoa2110345 .
Falsey AR, Sobieszczyk ME, Hirsch I, Sproule S, Robb ML, Corey L, Neuzil KM, Hahn W, Hunt J, Mulligan MJ, et al. Phase 3 safety and efficacy of AZD1222 (ChAdOx1 nCoV-19) COVID-19 vaccine. N Engl J Med. 2021. https://doi.org/10.1056/NEJMoa2105290 .
El Sahly HM, Baden LR, Essink B, Doblecki-Lewis S, Martin JM, Anderson EJ, Campbell TB, Clark J, Jackson LA, Fichtenbaum CJ, et al. Efficacy of the mRNA-1273 SARS-CoV-2 vaccine at completion of blinded phase. N Engl J Med. 2021. https://doi.org/10.1056/NEJMoa2113017 .
Tanriover MD, Doğanay HL, Akova M, Güner HR, Azap A, Akhan S, Köse Ş, Erdinç F, Akalın EH, Tabak ÖF, et al. Efficacy and safety of an inactivated whole-virion SARS-CoV-2 vaccine (CoronaVac): interim results of a double-blind, randomised, placebo-controlled, phase 3 trial in Turkey. Lancet. 2021;398(10296):213–22. https://doi.org/10.1016/s0140-6736(21)01429-x .
Kumar S, Saurabh MK, Maharshi V. Efficacy and safety of potential vaccine candidates against coronavirus disease 2019: a systematic review. J Adv Pharm Technol Res. 2021;12(3):215–21. https://doi.org/10.4103/japtr.JAPTR_229_20 .
Burgos-Salcedo J. A rational strategy to support approved COVID-19 vaccines prioritization. Hum Vaccin Immunother. 2021;17(10):3474–7. https://doi.org/10.1080/21645515.2021.1922060 .
Download references
Acknowledgements
This study was funded by the National Natural Science Foundation of China (72122001; 71934002) and the National Science and Technology Key Projects on Prevention and Treatment of Major infectious disease of China (2020ZX10001002). The funders had no role in study design, data collection and analysis, decision to publish, or preparation of the paper. No payment was received by any of the co-authors for the preparation of this article.
Author information
Qiao Liu and Chenyuan Qin are joint first authors
Authors and Affiliations
Department of Epidemiology and Biostatistics, School of Public Health, Peking University, Beijing, 100191, China
Qiao Liu, Chenyuan Qin, Min Liu & Jue Liu
Institute for Global Health and Development, Peking University, Beijing, 100871, China
Chenyuan Qin & Jue Liu
You can also search for this author in PubMed Google Scholar
Contributions
LQ and QCY contributed equally as first authors. LJ and LM contributed equally as correspondence authors. LJ and LM conceived and designed the study; LQ, QCY and LJ carried out the literature searches, extracted the data, and assessed the study quality; LQ and QCY performed the statistical analysis and wrote the manuscript; LJ, LM, LQ and QCY revised the manuscript. All authors read and approved the final manuscript.
Corresponding authors
Correspondence to Min Liu or Jue Liu .
Ethics declarations
Ethics approval and consent to participate.
Not applicable.
Consent for publication
Competing interests.
The authors have no conflicts of interest to declare that are relevant to the content of this article.
Supplementary Information
Additional file 1: table s1..
Characteristic of studies included for vaccine effectiveness.
Additional file 2: Table S2.
Characteristic of studies included for vaccine safety.
Rights and permissions
Open Access This article is licensed under a Creative Commons Attribution 4.0 International License, which permits use, sharing, adaptation, distribution and reproduction in any medium or format, as long as you give appropriate credit to the original author(s) and the source, provide a link to the Creative Commons licence, and indicate if changes were made. The images or other third party material in this article are included in the article's Creative Commons licence, unless indicated otherwise in a credit line to the material. If material is not included in the article's Creative Commons licence and your intended use is not permitted by statutory regulation or exceeds the permitted use, you will need to obtain permission directly from the copyright holder. To view a copy of this licence, visit http://creativecommons.org/licenses/by/4.0/ . The Creative Commons Public Domain Dedication waiver ( http://creativecommons.org/publicdomain/zero/1.0/ ) applies to the data made available in this article, unless otherwise stated in a credit line to the data.
Reprints and permissions
About this article
Cite this article.
Liu, Q., Qin, C., Liu, M. et al. Effectiveness and safety of SARS-CoV-2 vaccine in real-world studies: a systematic review and meta-analysis. Infect Dis Poverty 10 , 132 (2021). https://doi.org/10.1186/s40249-021-00915-3
Download citation
Received : 07 September 2021
Accepted : 01 November 2021
Published : 14 November 2021
DOI : https://doi.org/10.1186/s40249-021-00915-3
Share this article
Anyone you share the following link with will be able to read this content:
Sorry, a shareable link is not currently available for this article.
Provided by the Springer Nature SharedIt content-sharing initiative
- Effectiveness
- Meta-analysis
Infectious Diseases of Poverty
ISSN: 2049-9957
- Submission enquiries: Access here and click Contact Us
- General enquiries: [email protected]
Thank you for visiting nature.com. You are using a browser version with limited support for CSS. To obtain the best experience, we recommend you use a more up to date browser (or turn off compatibility mode in Internet Explorer). In the meantime, to ensure continued support, we are displaying the site without styles and JavaScript.
- View all journals
- My Account Login
- Explore content
- About the journal
- Publish with us
- Sign up for alerts
- Open access
- Published: 26 February 2024
Persistence in risk and effect of COVID-19 vaccination on long-term health consequences after SARS-CoV-2 infection
- Ivan Chun Hang Lam ORCID: orcid.org/0000-0002-5891-3940 1 na1 ,
- Ran Zhang 2 na1 ,
- Kenneth Keng Cheung Man ORCID: orcid.org/0000-0001-8645-1942 1 , 3 , 4 , 5 ,
- Carlos King Ho Wong ORCID: orcid.org/0000-0002-6895-6071 1 , 2 , 3 , 6 ,
- Celine Sze Ling Chui 3 , 7 , 8 , 9 ,
- Francisco Tsz Tsun Lai ORCID: orcid.org/0000-0002-9121-1959 1 , 2 , 3 , 9 ,
- Xue Li ORCID: orcid.org/0000-0003-4836-7808 1 , 3 , 9 , 10 ,
- Esther Wai Yin Chan ORCID: orcid.org/0000-0002-7602-9470 1 , 3 , 11 , 12 ,
- Chak Sing Lau ORCID: orcid.org/0000-0001-6698-8355 13 ,
- Ian Chi Kei Wong ORCID: orcid.org/0000-0001-8242-0014 1 , 3 , 9 , 14 na2 &
- Eric Yuk Fai Wan ORCID: orcid.org/0000-0002-6275-1147 1 , 2 , 3 , 9 na2
Nature Communications volume 15 , Article number: 1716 ( 2024 ) Cite this article
29k Accesses
2 Citations
403 Altmetric
Metrics details
- Disease prevention
- Epidemiology
- Infectious diseases
The persisting risk of long-term health consequences of SARS-CoV-2 infection and the protection against such risk conferred by COVID-19 vaccination remains unclear. Here we conducted a retrospective territory-wide cohort study on 1,175,277 patients with SARS-CoV-2 infection stratified by their vaccination status and non-infected controls to evaluate the risk of clinical sequelae, cardiovascular and all-cause mortality using a territory-wide public healthcare database with population-based vaccination records in Hong Kong. A progressive reduction in risk of all-cause mortality was observed over one year between patients with SARS-CoV-2 infection and controls. Patients with complete vaccination or have received booster dose incurred a lower risk of health consequences including major cardiovascular diseases, and all-cause mortality than unvaccinated or patients with incomplete vaccination 30-90 days after infection. Completely vaccinated and patients with booster dose of vaccines did not incur significant higher risk of health consequences from 271 and 91 days of infection onwards, respectively, whilst un-vaccinated and incompletely vaccinated patients continued to incur a greater risk of clinical sequelae for up to a year following SARS-CoV-2 infection. This study provided real-world evidence supporting the effectiveness of COVID-19 vaccines in reducing the risk of long-term health consequences of SARS-CoV-2 infection and its persistence following infection.
Similar content being viewed by others
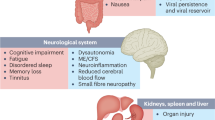
Long COVID: major findings, mechanisms and recommendations
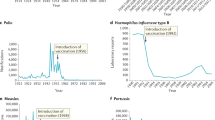
A guide to vaccinology: from basic principles to new developments
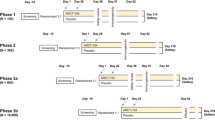
Safety, immunogenicity and efficacy of the self-amplifying mRNA ARCT-154 COVID-19 vaccine: pooled phase 1, 2, 3a and 3b randomized, controlled trials
Introduction.
Since the outbreak of the Coronavirus disease 2019 (COVID-19) pandemic caused by the SARS-CoV-2 virus in late 2019, substantial research has been undertaken to uncover the health consequences associated with SARS-CoV-2 infection. The current body of evidence has reported that patients with SARS-CoV-2 infection may incur a greater risk of acute and post-acute health consequences involving multiple organ systems and associated mortality following SARS-CoV-2 infection 1 , 2 , 3 .
A universal definition of post-COVID-19 condition remains to be determined owing to the discrepancy in definition published by different healthcare regulatory bodies 4 . Nevertheless, the current literature referred to clinical presentations develop within 30 days of initial infection as acute clinical sequelae, while complications that developed or persisted beyond the acute phase of SARS-CoV-2 infection were referred to as post-acute clinical sequelae 5 . Despite the current evidence suggesting that most patients may recover from SARS-CoV-2 infection within two to four weeks of symptoms appearance, the increased risk of incident cardiovascular, neurological, psychiatric diseases, diabetes and all-cause mortality was shown to persist for up to two years 6 , 7 , 8 , 9 , 10 . Patients with a severe SARS-CoV-2 infection or critically ill patients are at particular risk of developing long-term adverse outcome beyond their acute infection. Nevertheless, evidence emerged from the earlier stage of the pandemic were limited by the selection of under-represented samples as the study population 11 . Moreover, findings reported from the current body of literature were based largely on the assumption of a constant risk increase of clinical sequelae over a prolonged duration which may not be able to account for the change in risk over time, thus hindering the representativeness on the burden of the long-term health consequences of SARS-CoV-2 infection. Such speculation was evident from the gradual improvement in pulmonary function in most patients who recovered from severe SARS-CoV-2 infection over a three-monthly observation period for 12 months 12 . Meanwhile, a recent study in Israel have reported a considerably reduced risk of PASC towards the later period of SARS-CoV-2 infection over a course of one year amongst patients with mild infection 13 .
Shortly after the outbreak of the pandemic, the global initiative to develop vaccines against SARS-CoV-2 infection followed by the international vaccination campaign has been shown to successfully reduce the risk of primary infection, disease severity and hospitalization associated with SARS-CoV-2 infection 14 . A two dose vaccination regimen was initially recommended for the vast majority of brand of COVID-19 vaccines including the BioNtech and CoronaVac offered in Hong Kong based on the efficacy profile in preventing primary infection established from earlier clinical trials 15 , 16 . Nevertheless, a third and even fourth booster dose of the vaccine was later introduced in certain countries to restore immunity within the population in the face of the Omicron variant of SARS-CoV-2 17 , 18 . Despite the cumulative evidence of the covid-19 vaccines’ ability to reduce disease burden during acute infection, its effect in preventing the adverse outcome in the post-acute phase of SARS-CoV-2 infection remained largely unknown owing to the inconsistent findings from the existing studies 19 , 20 . While a protective effect of COVID-19 vaccination on incident health outcomes has been reported in several population and community-based studies, the extent of risk reduction, especially from the booster dose of vaccines remains to be evaluated 21 , 22 .
This population-based study aims to evaluate the progressive risk of health consequences associated with SARS-CoV-2 infection over 1 year and compare the risk and persistence of such risk differences between patients of different COVID-19 vaccination statuses.
A total of 1,175,277 patients with SARS-CoV-2 infection were identified in this study, of those, 124,443, 101,379, 457,896, and 491,559 patients were unvaccinated, had one, two and three or more doses of COVID-19 vaccination record prior to infection, respectively (Fig. 1 ). The median time between the latest dose of vaccination and SARS-CoV-2 infection for patients who have received one, two and three or more doses of COVID-19 vaccination was 18 (interquartile range 12–42), 175 (119–208) and 101 (37–170) days, respectively. The median follow-up period for controls, patients with SARS-CoV-2 who were unvaccinated, have received one, two and three or more doses of COVID-19 vaccination were 318 (173–329), 320 (253–331), 324 (312–329), 325 (312–330), and 171 (135–316) days, respectively. The baseline characteristics before and after weighting were summarized in Tables 1 and 2 , respectively. The SMDs of all baseline characteristics after weighting were less than 0.1, indicating a good balance between groups of patients with different vaccination statuses. The number of patients at risk at each observation period between days 0–30, 31–90, 91–180, 181–270, 271–365 were 2,815,023, 2,801,396, 2,764,243, 2,119,507, and 1,876,858, respectively.
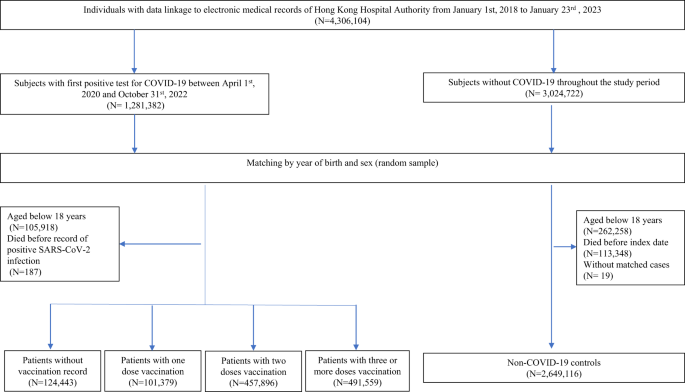
Flowchart for identifying study population.
Overall, a graded reduction in the risk of clinical sequelae among infected patients was observed over time and with the increased number of doses of COVID-19 vaccines received prior to infection. Compared to non-infected controls, patients with SARS-CoV-2 infection were observed to incur a greater risk of clinical sequelae including major cardiovascular diseases [HR dose=0: 4.64 (4.00, 5.38); dose=1: 3.13 (2.60, 3.76); dose=2: 2.53 (2.21, 2.89); dose ≥3: 1.99 (1.72, 2.29)], and all-cause mortality [HR dose=0: 18.89 (18.07, 19.74); dose=1: 8.96 (8.46, 9.48); dose=2: 3.95 (3.71, 4.20); dose ≥3: 1.74 (1.50, 2.02)] across patients with different COVID-19 vaccination status during the acute phase of infection. A graded decrease in risk was observed in patients with a greater number of doses of COVID-19 vaccination with unvaccinated and incompletely vaccinated patients incurring a greater risk of most clinical sequelae than those completely vaccinated and those who received booster doses of vaccines. Most notably, there was an approximately five-fold reduction in risk of all-cause mortality between unvaccinated patients (18.89; 18.07,19.74) and patients with complete vaccination (3.95; 3.71, 4.20) during the acute phase of infection. Such risk was further reduced amongst patients who received booster dose of vaccines (1.74; 1.50, 2.02).
The risk of all-cause mortality reduced progressively over one year between patients with SARS-CoV-2 infection and non-COVID-19 controls [0–30 d: 18.89 (18.07, 19.74); 31–90 d: 3.79 (3.56, 4.03); 91–180 d: 2.11 (1.97, 2.26); 181-270d: 1.97 (1.83, 2.13); 271–365 d: 2.12 (1.92, 2.33)]. A sharp decline in the risks of the outcomes were observed during the post-acute phase between 31 and 90 days of infection compared to the acute phase especially in patients who received complete and booster dose of vaccination. Nevertheless, the risk of several clinical sequelae including heart failure (dose=2: 1.43; 1.13, 1.81; dose ≥3: 1.35; 1.05, 1.75) and all-cause mortality (dose=2: 1.11; 1.02, 1.21) remained significantly greater in patients with SARS-CoV-2 infection. The risk of clinical sequelae further reduced in the subsequent observation period with no significant greater risk of clinical sequelae observed among completely vaccinated and patients with booster dose of vaccines from 271 and 91 days onwards, respectively. Meanwhile, the increased risk of certain clinical sequelae including all-cause mortality (2.12; 1.92, 2.33) continued to persist for up to a year amongst unvaccinated patients. (Tables 3 – 7 and Fig. 2 ) The cumulative incidence plots for individual observation windows were shown in Supplementary Fig. 1 . Sensitivity analyses reported largely consistent findings for the aforementioned outcomes (Supplementary Tables 2 – 11 ). A moderate increase in risk of lung cancer and lymphoma was observed during the first 30 days of infection. (Supplementary Table 12 ).
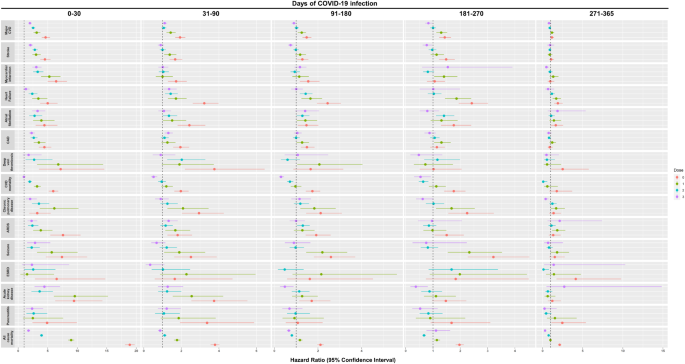
Note: Major CVD major cardiovascular disease, Composite outcome of stroke, heart failure and coronary artery disease, ARDS acute respiratory distress syndrome, CAD coronary artery disease, ESRD end-stage renal disease. Hazard ratio (HR) and 95% confidence interval (95% CI) were estimated by Cox regression, HR > 1 (or <1) indicates patients with COVID-19 had higher (lower) risk of sequelae compared to the non-COVID-19 control cohort. Dose 0, 1, 2, 3 refers to study population who have received 0 dose, 1 dose, 2 doses, and ≥3 doses of BioNtech or CoronaVac COVID-19 vaccines, respectively.
Patients aged above 65 or with a CCI of four or above are at a greater risk of clinical sequelae during the acute and post-acute phase of SARS-CoV-2 infection especially amongst unvaccinated or incompletely vaccinated patients. Male and female incurred broadly comparable risk of acute and post-acute sequelae with respect to their vaccination status. (Supplementary Tables 13 – 18 ).
This study examined the risk of long-term health consequences of SARS-CoV-2 infection involving multiple organ systems between patients with a history of SARS-CoV-2 infection and non-infected controls over the course of 365 days. The findings of this study showed a reduction in the risk of the majority of clinical sequelae over the course of the observation window suggesting the gradual subside of the risk of long-term health consequences over a year. COVID-19 vaccination, especially the uptake of the booster dose, was found to be effective in reducing the risk of health consequences. Patients who received three or more doses of vaccines did not incur any significant risk increased in clinical sequelae from 91 days onwards from their initial infection. On the other hand, unvaccinated patients were at a greater risk of several clinical sequelae including all-cause mortality up to one year following infection. Such findings provided evidence to support the potential protection from vaccination in reducing the risk of long-term health consequences following SARS-CoV-2 infection.
Previous studies that characterized and evaluated the risk of post-acute sequelae have reported a considerable increase in risk of clinical sequelae involving multiple organ systems 1 , 2 , 7 , 8 , 10 . In particular, patients with a severe SARS-CoV-2 infection during the acute phase were reported to incur further risk of post-acute clinical sequelae following infection 23 . Nevertheless, evidence that emerged in the early stage of the pandemic has been generated based on data with limited representation of the general population such as discharged hospitalized and older patients with SARS-CoV-2 infection. Since these factors were indicative of patients with more severe disease outcomes compared to the general population, the findings from these studies have shown great discrepancies in the prevalence and risk of clinical sequelae following infection 11 , 24 . More recent evidence from several large-scale, nationwide population-based studies have indicated a gradual improvements in their recovery status characterized by the reduction in prevalence of self-reported symptoms and proportion of infected individuals reporting non-recovery from health outcomes associated with SARS-CoV-2 infection 25 , 26 . A reduction in risk of post-infection clinical sequelae was also reported 6 months following their initial infection amongst individuals with a mild SARS-CoV-2 infection 13 . With consideration of the comparable disease severity induced by the dominant XBB variant to the other sublineages of the omicron strain of SARS-CoV-2, the current body of evidence may indicate the gradual subside and lesser clinical burden on the long-term health consequences caused by such milder strain of SARS-CoV-2 virus than that reported in the earlier stage of the pandemic 27 . Nonetheless, high-risk patients including older people, lack of vaccination, immunosuppression, and individuals with certain underlying comorbidities may still be more vulnerable to poor clinical prognosis from the adverse clinical outcomes following SARS-CoV-2 infection. This emphasizes the need for closer monitoring for these patient groups and provide timely treatment if necessary.
As the pandemic evolved, the global COVID-19 vaccination campaigns have shown to be effective in preventing serious illness and death associated with SARS-CoV-2 infection. Recent research on the booster dose of COVID-19 vaccination has reported substantial extra protection against initial, severe SARS-CoV-2 infection and the rate of mortality by over 80% with a greater risk reduction estimated amongst high-risk population with multi-morbidity, in addition to that conferred by the first and second doses 28 , 29 , 30 , 31 . The bivalent omicron-containing booster vaccine was also shown to confer broader immunity against the Omicron variant of SARS-CoV-2 and reduce hospitalizations or deaths due to SARS-CoV-2 infection 32 , 33 . Previous studies investigating the impact of COVID-19 vaccines on the risk of developing clinical sequelae associated with SARS-CoV-2 infection have reported inconsistent findings due to the difference in the methodological approach employed to evaluate two key aspects: the effect of vaccination on the risk of incident clinical sequelae following SARS-CoV-2 infection and the prevalence of post-infection sequelae outcomes among COVID-19 survivors 19 , 20 . Further to the current evidence supporting the protection against both acute and post-acute health consequences of SARS-CoV-2 infection conferred by COVID-19 vaccination, the findings of this study demonstrated a graded reduction in the incidence and the persistence in risk according to the number of vaccines doses received prior to infection. The protective effect was more pronounced among the older people and patients with a greater degree of morbidity. Nevertheless, further studies are warranted to evaluate the effect of COVID-19 vaccination in reducing the prevalence of clinical sequelae and understand the potential protective effect of COVID-19 vaccination in patients who have developed onset of clinical sequelae associated with SARS-CoV-2 infection.
Despite the recapitulation of evidence demonstrating the association of COVID-19 vaccination with a reduced risk of clinical sequelae and adverse clinical outcomes during the acute phase of SARS-CoV-2 infection, its protective effect against clinical sequelae in the post-acute phase of infection remained largely unknown. One putative mechanism for the protection effects against the long-term health consequences from vaccination observed could be attributed to the protection against severe SARS-CoV-2 infection during the acute infection may subsequently reduce the risk of long-term health outcomes. The most common COVID-19 vaccines development approach was based on the spike protein of the virus as an antigen accessible by antibodies and immunological cells in the body. The BioNtech COVID-19 vaccines contains mRNA molecules carrying the information for the SARS-CoV-2 spike protein whilst the CoronaVac consisted of whole attenuated SARS-CoV-2 virus capable of provoking an immune response upon administration. The spike proteins attracts antibodies and provoke a high response of sub-types CD8+ killer and CD4 helper T cells, signaling the production of cytokines and proliferations of T memory cells to mediate a lasting immunity, preventing severe health consequences from SARS-CoV-2 infection and the associated irreversible damage to vital organ systems in our body, constituting to a reduced risk of subsequent clinical sequelae beyond the acute phase of SARS-CoV-2 infection 34 .
The findings of this study demonstrated a gradual reduction in the risk of long-term health consequences associated with SARS-CoV-2 over one year, indicating a lesser disease burden compared to that reported in earlier studies as well as the effect of COVID-19 vaccination in reducing the risk of clinical sequelae beyond the acute phase of SARS-CoV-2 infection. The comprehensive records of vaccination records provided by the Department of Health ensures the accuracy of information on the vaccination status of individuals reported in this study. As the pandemic progresses, our findings provided real-world evidence supporting the effectiveness of the COVID-19 vaccines in the prevention of long-term health consequences following SARS-CoV-2 infection. Nevertheless, our study is subject to several limitations. First, detection bias might be inherent in this study due to the potential under-reporting of existing underlying conditions prior to a diagnosis of SARS-CoV-2 infection. In addition, the increased healthcare contacts from receiving further examination amongst patients diagnosed with SARS-CoV-2 infection could result in the increased diagnosis of condition which could have persisted prior to infection. For instance, patients presenting with sequelae may have developed certain diseases prior to their diagnosis of SARS-CoV-2 infection; yet they did not receive a diagnosis for those conditions until a confirmed diagnosis of SARS-CoV-2 infection, resulting in the over-attribution of disease diagnosis as post-infection sequelae. Nevertheless, the history of chronic diseases in the HKHA has been recorded with high completeness as demonstrated in previous study, thus ensuring the accuracy and reliability of data to distinguish existing comorbidities and sequelae of SARS-CoV-2 infection 35 . Given the sufficiently long observation period, any existing comorbidities of subjects that were not captured is considered unlikely. Such error would also have a minimal effect on sequelae observed during the post-acute phase of infection. Furthermore, sequelae reported in this study including stroke, MI and seizure were largely of great disease severity which would typically result in distinct symptoms upon the onset of disease. Thus, the incidence of such sequelae would not be affected by the increased surveillance on patients following SARS-CoV-2 infection. Second, potential selection bias may also arise from the increased SARS-CoV-2 testing amongst individuals with prevalent comorbidities. Third, the emergence of novel variant of SARS-CoV-2 is strongly correlated with better vaccination coverage and booster dose of vaccination. Given the lower severity and risk of health consequences associated with Delta and Omicron variant emerged later in the pandemic, this could lead to potential confounding bias in our findings. Nevertheless, sensitivity analysis which adjusted for the likely variant of SARS-CoV-2 amongst the infected patients have reported a largely consistent result as the main analysis suggesting that such potential confounding bias should not impact the study’s overall conclusions. Fourth, the estimation of period-specific HR is subjected to possible built-in selection bias from the censoring of patients upon the incident of clinical sequelae and the systematic difference in distribution of unknown ubiquitous factors between survivors from separate cohorts exist generally especially toward the later stage of follow-up. This could contribute to the reduction in the magnitude of HR estimated in the later observation windows. Further study is warranted to evaluate the effect of the built-in selection bias described 36 , 37 . Lastly, residual confounding bias can remain even after weighing subjects according to their propensity scores. Several important unmeasured confounders, namely obesity, smoking, socioeconomic status, educational level and strains of SARS-CoV-2 virus found in individual patients, could not be accounted for in this study owing to data availability, which may have introduced bias to our results.
This study examined the progressive risk of acute and post-acute sequelae following SARS-CoV-2 infection at 3 monthly intervals up to a year amongst patients with different vaccination status. The risk of clinical sequelae was observed to reduce gradually over the observation period. Complete vaccination and the uptake of booster dose of COVID-19 vaccines were found to further reduce the risk and persistence in risk of long-term health consequences of SARS-CoV-2 infection. The findings of this study indicated a lesser disease burden caused by health consequences of SARS-CoV-2 infection compared to that reported in earlier study and provided real-world evidence supporting the effectiveness of COVID-19 vaccines in reducing the risk of long-term health consequences following infection.

Data source
In this retrospective cohort study, routine electronic medical records were retrieved from the Hong Kong Hospital Authority (HKHA). The Hospital Authority is a statutory body that manages all public hospitals and their ambulatory clinics in Hong Kong. The service is available to all HK residents (> 7.2 million) covering ~80% of all routine hospital admissions 38 . Electronic medical records from the HKHA database consisted of disease diagnoses recorded in planned or unplanned doctor consultations from in- and outpatient hospitals and emergency visits, thus allowing timely capture of all medical records of all users of the public health services in HK. Records were obtained from the Hong Kong Deaths Registry to identify mortality in this study. Information on vaccination status was provided by the Department of Health, The Government of Hong Kong Special Administrative Region whilst records of confirmed cases of SARS-CoV-2 infection were obtained from the Centre for Health Protection of the Government, the Hong Kong Special Administrative Region and HKHA. Anonymized unique patient identifiers were used to integrate these databases. These population-based databases have been used in previous studies on the long-term sequelae of COVID-19 infection, COVID-19 vaccines safety surveillance and effectiveness 3 , 6 , 38 , 39 , 40 , 41 , 42 .
Study design and population
Individuals with data linkage to electronic medical records of Hong Kong Hospital Authority from January 1, 2018 to January 23, 2023 were eligible for this study. A cohort study was conducted to evaluate the risk of health consequences between patients with and without SARS-CoV-2 infection aged 18 years or above. Patients with an incident SARS-CoV-2 infection (confirmed by rapid antigen test [RAT] or polymerase chain reaction [PCR] test in throat swab, nasopharyngeal aspirate, or deep throat sputum specimens) between April 1, 2020 and October 31, 2022 were matched to non-infected controls without a positive SARS-CoV-2 test record throughout the study period with the exact birth-year and sex. All individuals without a record of positive test record of the same birth-year and sex were selected as matched controls. Patients with SARS-CoV-2 infection were further stratified into (1) unvaccinated (0 dose), (2) incompletely vaccinated (1 dose), (3) completely (2 doses), and (4) vaccinated with booster doses ( ≥ 3 doses) according to the number of BioNtech or CoronaVac vaccines received prior to first SARS-CoV-2 infection. The index date of patients with SARS-CoV-2 infection was defined as the date of first diagnosis date of SARS-CoV-2 infection. The identical index date was assigned to randomly selected corresponding matched controls as the pseudo-index date.
All subjects were followed up from the index date until the date of death, the occurrence of outcome, SARS-CoV-2 re-infection or the end of the separate observation periods at 30, 90, 180, 270, and 365 days after the index date or the end of the study period January 31, 2023, whichever occurred earlier.
Anonymized longitudinal clinical healthcare data since 2016 and the earliest date of data availability were obtained for all subjects from HKHA. Relevant data included baseline demographic (sex, age and Charlson Comorbidity Index); pre-existing morbidities captured by clinical diagnosis codes (cardiovascular, cerebrovascular, respiratory, chronic kidney, liver diseases, rheumatoid arthritis and malignancy; Supplementary Table 1 ), history of long-term medication (renin–angiotensin-system agents, beta-blockers, calcium channel blockers, diuretics, nitrates, lipid-lowering agents, insulins, antidiabetic drugs, oral anticoagulants, antiplatelets and immunosuppressants) and COVID-19 vaccination status before index date.
This study was reported according to the Reporting of studies Conducted using Observational Routinely-collected Data (RECORD), extended from the Strengthening the Reporting of Observational Studies in Epidemiology (STROBE) guideline.
Outcomes of clinical diagnosis
The outcomes of this study were selected based on previous evidence on the risk of clinical sequelae associated with SARS-CoV-2 infection which includes incidences of major cardiovascular diseases (a composite outcome of stroke, heart failure and coronary heart disease), stroke, myocardial infarction (MI), heart failure, atrial fibrillation, coronary artery disease, deep vein thrombosis (DVT), chronic pulmonary disease, acute respiratory distress syndrome, seizure, end-stage renal disease, acute kidney injury, pancreatitis, cardiovascular and all-cause mortality 1 , 8 , 9 , 10 , 43 . Outcomes were identified based on the International Classification of Diseases, Ninth Revision, Clinical Modification (ICD-9-CM; Supplementary Table 1 ).
Statistical analyses
Inverse Probability Treatment Weighting (IPTW) 44 based on age, sex, Charlson Comorbidity index (CCI), history of separate class of medication (renin–angiotensin system agents, beta-blockers, calcium channel blockers, diuretics, nitrates, lipid-lowering agents, insulins, antidiabetic drugs, oral anticoagulants, antiplatelets and immunosuppressants), the number of hospital admission and doctor consultation within one year of index date was applied to account for potential confounding factors. Standardized mean difference (SMD) between cases and controls was estimated, SMD ≤ 0.1 was regarded as sufficient balance between case and control groups 45 . Subjects with a history of outcome of interest were excluded from the analysis of the specific conditions whilst continued to be considered at risk for other disease outcomes. The incidence rate (per 1000 person-years), hazard ratio (HR) and 95% confidence interval (CI) of each outcome were estimated between COVID and non-COVID-19 cohorts separately for each of the observation period using Cox proportional hazard regressions. Sensitivity analysis was performed by only including individuals with a positive PCR SARS-CoV-2 screening test results, cases of SARS-CoV-2 infection from the Omicron wave in Hong Kong 46 , unvaccinated patients with COVID-19 and matched control with the same vaccination status, adjusting for the likely variant of SARS-CoV-2 responsible for the infection, excluding patients who received their last dose of vaccine more than 6 months before SARS-CoV-2 infection owing to the waning of immunity following vaccination 47 , 48 , and controlling for the false discovery rate at 0.05 through Benjamin-Hochberg procedure 49 . Lung cancer, brain cancer, and lymphoma which were considered to have a prolonged latent period for their development were included as negative control outcomes to detect possible testing bias. Subgroup analyses were predefined taking account of the risk factors of post-COVID-19 condition 50 . Patients were stratified by (1) age (≤65, >65), (2) sex, (3) Charlson Comorbidity index (CCI; <4, ≥4).
All statistical analyses were performed using R version 4.1.2 (R Foundation for Statistical Computing, Vienna, Austria). All significance tests were two‐tailed. A P value less than 0.05 or 95% CI excluding 1.0 were taken to indicate statistical significance. At least two investigators (ICHL, RZ, and EYFW) conducted each of the statistical analyses independently for quality assurance.
Data access
EYFW and ICKW had full access to all the data in the study and took responsibility for the integrity of the data and the accuracy of the data analysis.
Ethical approval
Ethical approval for this study was granted by the Institutional Review Board of the University of HK/HA HK West Cluster (UW20-556 and UW21-149) and Department of Health, HK (L/M21/2021 and L/M175/2022) with an exemption for informed consent from participants as patients’ confidentiality was maintained in this retrospective cohort study.
Reporting summary
Further information on research design is available in the Nature Portfolio Reporting Summary linked to this article.
Data availability
The data contains confidential information and hence cannot be shared with the public due to third-party use restrictions. Local academic institutions, government departments, or non-governmental organizations may apply for the access to data through the Hospital Authority’s data-sharing portal ( https://www3.ha.org.hk/data ).
Code availability
The code used in this study is available on Zenodo ( https://doi.org/10.5281/zenodo.10132693 ).
Daugherty, S. E. et al. Risk of clinical sequelae after the acute phase of SARS-CoV-2 infection: retrospective cohort study. BMJ 373 , n1098 (2021).
Article PubMed Google Scholar
Al-Aly, Z., Xie, Y. & Bowe, B. High-dimensional characterization of post-acute sequelae of COVID-19. Nature 594 , 259–264 (2021).
Article ADS PubMed CAS Google Scholar
Lam, I. C. H. et al. Long-term post-acute sequelae of COVID-19 infection: a retrospective, multi-database cohort study in Hong Kong and the UK. eClinicalMedicine 60 , 102000 (2023).
Article PubMed PubMed Central Google Scholar
Chaichana, U. et al. Definition of post-COVID-19 condition among published research studies. JAMA Netw. Open 6 , e235856–e235856 (2023).
Thaweethai, T. et al. Development of a definition of postacute sequelae of SARS-CoV-2 infection. JAMA 329 , 1934–1946 (2023).
Article PubMed PubMed Central CAS Google Scholar
Wan, E. Y. F. et al. Association of COVID-19 with short- and long-term risk of cardiovascular disease and mortality: a prospective cohort in UK Biobank. Cardiovasc. Res. 119 , 1718–1727 (2023).
Taquet, M. et al. Neurological and psychiatric risk trajectories after SARS-CoV-2 infection: an analysis of 2-year retrospective cohort studies including 1 284 437 patients. Lancet Psychiatry 9 , 815–827 (2022).
Xie, Y. & Al-Aly, Z. Risks and burdens of incident diabetes in long COVID: a cohort study. Lancet Diabetes Endocrinol. 10 , 311–321 (2022).
Xie, J. et al. Clinical and genetic risk factors for acute incident venous thromboembolism in ambulatory patients with COVID-19. JAMA Intern. Med. 182 , 1063–1070 (2022).
Xu, E., Xie, Y. & Al-Aly, Z. Long-term neurologic outcomes of COVID-19. Nat. Med. 28 , 2406–2415 (2022).
Wu, Q., Ailshire, J. A. & Crimmins, E. M. Long COVID and symptom trajectory in a representative sample of Americans in the first year of the pandemic. Sci. Rep. 12 , 11647 (2022).
Article ADS PubMed PubMed Central CAS Google Scholar
Wu, X. et al. 3-month, 6-month, 9-month, and 12-month respiratory outcomes in patients following COVID-19-related hospitalisation: a prospective study. Lancet Respir. Med. 9 , 747–754 (2021).
Mizrahi, B. et al. Long covid outcomes at one year after mild SARS-CoV-2 infection: nationwide cohort study. BMJ 380 , e072529 (2023).
Dagan, N. et al. BNT162b2 mRNA Covid-19 vaccine in a nationwide mass vaccination setting. New Engl. J. Med. 384 , 1412–1423 (2021).
Article PubMed CAS Google Scholar
Polack, F. P. et al. Safety and efficacy of the BNT162b2 mRNA Covid-19 vaccine. New Engl. J. Med. 383 , 2603–2615 (2020).
Tanriover, M. D. et al. Efficacy and safety of an inactivated whole-virion SARS-CoV-2 vaccine (CoronaVac): interim results of a double-blind, randomised, placebo-controlled, phase 3 trial in Turkey. Lancet 398 , 213–222 (2021).
Menni, C. et al. COVID-19 vaccine waning and effectiveness and side-effects of boosters: a prospective community study from the ZOE COVID Study. Lancet Infect. Dis. 22 , 1002–1010 (2022).
Andrews, N. et al. Covid-19 vaccine effectiveness against the Omicron (B.1.1.529) variant. New Engl. J. Med. 386 , 1532–1546 (2022).
Byambasuren, O., Stehlik, P., Clark, J., Alcorn, K. & Glasziou, P. Effect of covid-19 vaccination on long covid: systematic review. BMJ Med. 2 , e000385 (2023).
Notarte, K. I. et al. Impact of COVID-19 vaccination on the risk of developing long-COVID and on existing long-COVID symptoms: a systematic review. eClinicalMedicine 53 , 101624 (2022).
Al-Aly, Z., Bowe, B. & Xie, Y. Long COVID after breakthrough SARS-CoV-2 infection. Nat. Med. 28 , 1461–1467 (2022).
Ayoubkhani, D. et al. Risk of long COVID in people infected with severe acute respiratory syndrome coronavirus 2 after 2 doses of a coronavirus disease 2019 vaccine: community-based, matched cohort study. Open Forum Infect. Dis. 9 , ofac464 (2022).
Xie, Y., Bowe, B. & Al-Aly, Z. Burdens of post-acute sequelae of COVID-19 by severity of acute infection, demographics and health status. Nat. Commun. 12 , 6571 (2021).
Ballering, A. V., van Zon, S. K. R., olde Hartman, T. C. & Rosmalen, J. G. M. Persistence of somatic symptoms after COVID-19 in the Netherlands: an observational cohort study. Lancet 400 , 452–461 (2022).
Ballouz, T. et al. Recovery and symptom trajectories up to two years after SARS-CoV-2 infection: population based, longitudinal cohort study. BMJ 381 , e074425 (2023).
Hastie, C. E. et al. Natural history of long-COVID in a nationwide, population cohort study. Nat. Commun. 14 , 3504 (2023).
Mahase, E. Covid-19: what do we know about XBB.1.5 and should we be worried? BMJ 380 , p153 (2023).
Article Google Scholar
Arbel, R. et al. BNT162b2 vaccine booster and mortality due to Covid-19. New Engl. J. Med 385 , 2413–2420 (2021).
Bar-On, Y. M. et al. Protection of BNT162b2 vaccine booster against Covid-19 in Israel. New Engl. J. Med 385 , 1393–1400 (2021).
Magen, O. et al. Fourth dose of BNT162b2 mRNA Covid-19 vaccine in a nationwide setting. New Engl. J. Med 386 , 1603–1614 (2022).
Barda, N. et al. Effectiveness of a third dose of the BNT162b2 mRNA COVID-19 vaccine for preventing severe outcomes in Israel: an observational study. Lancet 398 , 2093–2100 (2021).
Arbel, R. et al. Effectiveness of a bivalent mRNA vaccine booster dose to prevent severe COVID-19 outcomes: a retrospective cohort study. Lancet Infect. Dis. 23 , 914–921 (2023).
Moreira, E. D. et al. Safety and efficacy of a third dose of BNT162b2 Covid-19 vaccine. New Engl. J. Med. 386 , 1910–1921 (2022).
Mascellino, M. T., Di Timoteo, F., De Angelis, M. & Oliva, A. Overview of the main anti-SARS-CoV-2 vaccines: mechanism of action, efficacy and safety. Infect. Drug Resist. 14 , 3459–3476 (2021).
Wong, M. C. S. et al. Health services research in the public healthcare system in Hong Kong: an analysis of over 1 million antihypertensive prescriptions between 2004–2007 as an example of the potential and pitfalls of using routinely collected electronic patient data. BMC Health Serv. Res. 8 , 138 (2008).
Hernán, M. A. The hazards of hazard ratios. Epidemiology 21 , 13–15 (2010).
Bartlett, J. W. et al. The hazards of period specific and weighted hazard ratios. Stat. Biopharm. Res. 12 , 518–519 (2020).
Lai, F. T. T. et al. Carditis after COVID-19 vaccination with a messenger RNA vaccine and an inactivated virus vaccine. Ann. Intern. Med. 175 , 362–370 (2022).
Wan, E. Y. F. et al. Safety of an inactivated, whole-virion COVID-19 vaccine (CoronaVac) in people aged 60 years or older in Hong Kong: a modified self-controlled case series. Lancet Healthy Longev. 3 , e491–e500 (2022).
Yan, V. K. C. et al. Effectiveness of BNT162b2 and CoronaVac vaccinations against mortality and severe complications after SARS-CoV-2 Omicron BA.2 infection: a case-control study. Emerg. Microbes Infect. 11 , 2304–2314 (2022).
Yan, X. et al. Follow-up study of pulmonary function among COVID-19 survivors 1 year after recovery. J. Infect. 83 , 381–412 (2021).
Wan, E. Y. F. et al. Bell’s palsy following vaccination with mRNA (BNT162b2) and inactivated (CoronaVac) SARS-CoV-2 vaccines: a case series and nested case-control study. Lancet Infect. Dis. 22 , 64–72 (2022).
Cohen, K. et al. Risk of persistent and new clinical sequelae among adults aged 65 years and older during the post-acute phase of SARS-CoV-2 infection: retrospective cohort study. BMJ 376 , e068414 (2022).
Ruth, C. et al. Long-Term Outcomes Of Manitoba’s Insight Mentoring Program: A Comparative Statistical Analysis (Manitoba Centre for Health Policy, Winnipeg, MB, 2015).
Austin, P. C. Using the standardized difference to compare the prevalence of a binary variable between two groups in observational research. Commun. Stat. Simul. Comput. 38 , 1228–1234 (2009).
Article MathSciNet Google Scholar
Xie, R. et al. Resurgence of Omicron BA.2 in SARS-CoV-2 infection-naive Hong Kong. Nat. Commun. 14 , 2422 (2023).
Feikin, D. R. et al. Duration of effectiveness of vaccines against SARS-CoV-2 infection and COVID-19 disease: results of a systematic review and meta-regression. Lancet 399 , 924–944 (2022).
Ponticelli, D. et al. Dynamics of antibody response to BNT162b2 mRNA COVID-19 vaccine after 6 months. J. Travel Med. 28 , taab173 (2021).
Benjamini, Y. & Hochberg, Y. Controlling the false discovery rate: a practical and powerful approach to multiple testing. J. R. Stat. Soc. Ser. B (Methodol.) 57 , 289–300 (1995).
MathSciNet Google Scholar
Subramanian, A. et al. Symptoms and risk factors for long COVID in non-hospitalized adults. Nat. Med. 28 , 1706–1714 (2022).
Download references
Acknowledgements
The authors thank the Hospital Authority for the generous provision of data for this study. This work was supported by HMRF Research on COVID-19, The Hong Kong Special Administrative Region (HKSAR) Government (Principal Investigator: EWYC; Ref No. COVID1903011); Collaborative Research Fund, University Grants Committee, the HKSAR Government (Principal Investigator: ICKW; Ref. No. C7154-20GF); and Research Grant from the Health Bureau, the HKSAR Government (Principal Investigator: I.C.K.W.; Ref. No. COVID19F01). I.C.K.W. and F.T.T.L. are partially supported by the Laboratory of Data Discovery for Health (D 2 4H) funded by the AIR@InnoHK administered by the Innovation and Technology Commission. The funders did not have any role in design and conduct of the study; collection, management, analysis, and interpretation of the data; preparation, review, or approval of the manuscript; and decision to submit the manuscript for publication.
Author information
These authors contributed equally: Ivan Chun Hang Lam, Ran Zhang.
These authors jointly supervised this work: Ian Chi Kei Wong, Eric Yuk Fai Wan.
Authors and Affiliations
Centre for Safe Medication Practice and Research, Department of Pharmacology and Pharmacy, Li Ka Shing Faculty of Medicine, The University of Hong Kong, Hong Kong SAR, China
Ivan Chun Hang Lam, Kenneth Keng Cheung Man, Carlos King Ho Wong, Francisco Tsz Tsun Lai, Xue Li, Esther Wai Yin Chan, Ian Chi Kei Wong & Eric Yuk Fai Wan
Department of Family Medicine and Primary Care, School of Clinical Medicine, Li Ka Shing Faculty of Medicine, The University of Hong Kong, Hong Kong SAR, China
Ran Zhang, Carlos King Ho Wong, Francisco Tsz Tsun Lai & Eric Yuk Fai Wan
Laboratory of Data Discovery for Health (D24H), Hong Kong Science and Technology Park, Sha Tin, Hong Kong SAR, China
Kenneth Keng Cheung Man, Carlos King Ho Wong, Celine Sze Ling Chui, Francisco Tsz Tsun Lai, Xue Li, Esther Wai Yin Chan, Ian Chi Kei Wong & Eric Yuk Fai Wan
Research Department of Practice and Policy, School of Pharmacy, University College London, London, UK
Kenneth Keng Cheung Man
Centre for Medicines Optimisation Research and Education, University College London Hospitals NHS Foundation Trust, London, UK
Department of Infectious Disease Epidemiology & Dynamics, Faculty of Epidemiology and Population Health, London School of Hygiene and Tropical Medicine, London, UK
Carlos King Ho Wong
School of Nursing, Li Ka Shing Faculty of Medicine, The University of Hong Kong SAR, Hong Kong, China
Celine Sze Ling Chui
School of Public Health, Li Ka Shing Faculty of Medicine, The University of Hong Kong, Hong Kong SAR, China
Advanced Data Analytics for Medical Science (ADAMS) Limited, Hong Kong, China
Celine Sze Ling Chui, Francisco Tsz Tsun Lai, Xue Li, Ian Chi Kei Wong & Eric Yuk Fai Wan
Department of Medicine, School of Clinical Medicine, Li Ka Shing Faculty of Medicine, The University of Hong Kong, Hong Kong SAR, China
Department of Medicine, The University of Hong Kong-Shenzhen Hospital, Shenzhen, China
Esther Wai Yin Chan
The University of Hong Kong Shenzhen Institute of Research and Innovation, Hong Kong SAR, China
Division of Rheumatology and Clinical Immunology, Department of Medicine, School of Clinical Medicine, Li Ka Shing Faculty of Medicine, The University of Hong Kong, Hong Kong SAR, China
Chak Sing Lau
Aston Pharmacy School, Aston University, Birmingham, UK
Ian Chi Kei Wong
You can also search for this author in PubMed Google Scholar
Contributions
I.C.H.L., E.Y.F.W., and I.C.K.W. had the original idea for the study, contributed to the development of the study, extracted data from the source database, constructed the study design and the statistical model, reviewed the literature, and act as guarantors for the study. I.C.H.L., R.Z., and E.Y.F.W. accessed and verified the data, and performed statistical analysis. I.C.H.L., R.Z., E.Y.F.W., and I.C.K.W. wrote the first draft of the manuscript. I.C.K.W. is the principal investigator and provided oversight for all aspects of this project. K.K.C.M., C.K.H.W., C.S.L.C., F.T.T.L., X.L., E.W.Y.C., C.S.L., E.Y.F.W., and I.C.K.W. provided critical input to the analyses, study design, and discussion. All authors contributed to the interpretation of the analysis, critically reviewed and revised the manuscript, and approved the final manuscript to be submitted.
Corresponding authors
Correspondence to Ian Chi Kei Wong or Eric Yuk Fai Wan .
Ethics declarations
Competing interests.
K.K.C.M. reported grants from the Hong Kong Research Grant Council, the CW Maplethorpe Fellowship, UK National Institute for Health and Care Research, European Commission Framework Horizon 2020, Innovation and Technology Commission of the Government of the Hong Kong Special Administrative Region, and personal fees from IQVIA Ltd outside the submitted work. CKHW. reports the receipt of General Research Fund, Research Grant Council, Government of Hong Kong SAR; EuroQol Research Foundation; AstraZeneca and Boehringer Ingelheim, all outside the submitted work. C.S.L.C. has received grants from the Health Bureau of the Hong Kong Government, Hong Kong Research Grant Council, Hong Kong Innovation and Technology Commission, Pfizer, IQVIA, and Amgen; and personal fees from PrimeVigilance; outside the submitted work. F.T.T.L. has been supported by the RGC Postdoctoral Fellowship under the Hong Kong Research Grants Council and has received research grants from the Health Bureau of the Government of the Hong Kong Special Administrative Region, outside the submitted work. X.L. has received research grants from Hong Kong Health and Medical Research Fund (HMRF, HMRF Fellowship Scheme, HKSAR), Research Grants Council Early Career Scheme (RGC/ECS, HKSAR), Janssen and Pfizer; internal funding from the University of Hong Kong; consultancy fees from Merck Sharp & Dohme and Pfizer, unrelated to this work. E.W.C. reports grants from Research Grants Council (RGC, Hong Kong), Research Fund Secretariat of the Food and Health Bureau, National Natural Science Fund of China, Wellcome Trust, Bayer, Bristol-Myers Squibb, Pfizer, Janssen, Amgen, Takeda, and Narcotics Division of the Security Bureau of the Hong Kong Special Administrative Region; honorarium from Hospital Authority; outside the submitted work. ICKW reports grants from Amgen, Bristol-Myers Squibb, Pfizer, Janssen, Bayer, GSK and Novartis, the Hong Kong RGC, and the Hong Kong Health and Medical Research Fund in Hong Kong, National Institute for Health Research in England, European Commission, National Health and Medical Research Council in Australia, consulting fees from IQVIA and World Health Organization, payment for expert testimony for Appeal Court of Hong Kong and is a non-executive director of Jacobson Medical in Hong Kong and Therakind in England, outside of the submitted work; no other relationships or activities that could appear to have influenced the submitted work. EYFW has received research grants from the Health Bureau of the Government of the Hong Kong Special Administrative Region, and the Hong Kong Research Grants Council, outside the submitted work. The remaining authors declare no competing interests.
Peer review
Peer review information.
Nature Communications thanks the anonymous reviewer(s) for their contribution to the peer review of this work. A peer review file is available.
Additional information
Publisher’s note Springer Nature remains neutral with regard to jurisdictional claims in published maps and institutional affiliations.
Supplementary information
Supplementary information, peer review file, reporting summary, rights and permissions.
Open Access This article is licensed under a Creative Commons Attribution 4.0 International License, which permits use, sharing, adaptation, distribution and reproduction in any medium or format, as long as you give appropriate credit to the original author(s) and the source, provide a link to the Creative Commons licence, and indicate if changes were made. The images or other third party material in this article are included in the article’s Creative Commons licence, unless indicated otherwise in a credit line to the material. If material is not included in the article’s Creative Commons licence and your intended use is not permitted by statutory regulation or exceeds the permitted use, you will need to obtain permission directly from the copyright holder. To view a copy of this licence, visit http://creativecommons.org/licenses/by/4.0/ .
Reprints and permissions
About this article
Cite this article.
Lam, I.C.H., Zhang, R., Man, K.K.C. et al. Persistence in risk and effect of COVID-19 vaccination on long-term health consequences after SARS-CoV-2 infection. Nat Commun 15 , 1716 (2024). https://doi.org/10.1038/s41467-024-45953-1
Download citation
Received : 24 August 2023
Accepted : 08 February 2024
Published : 26 February 2024
DOI : https://doi.org/10.1038/s41467-024-45953-1
Share this article
Anyone you share the following link with will be able to read this content:
Sorry, a shareable link is not currently available for this article.
Provided by the Springer Nature SharedIt content-sharing initiative
By submitting a comment you agree to abide by our Terms and Community Guidelines . If you find something abusive or that does not comply with our terms or guidelines please flag it as inappropriate.
Quick links
- Explore articles by subject
- Guide to authors
- Editorial policies
Sign up for the Nature Briefing newsletter — what matters in science, free to your inbox daily.


An official website of the United States government
The .gov means it’s official. Federal government websites often end in .gov or .mil. Before sharing sensitive information, make sure you’re on a federal government site.
The site is secure. The https:// ensures that you are connecting to the official website and that any information you provide is encrypted and transmitted securely.
- Publications
- Account settings
Preview improvements coming to the PMC website in October 2024. Learn More or Try it out now .
- Advanced Search
- Journal List
- Wiley - PMC COVID-19 Collection

Effectiveness of COVID‐19 vaccines: findings from real world studies
David a henry.
1 Institute for Evidence-Based Healthcare, Bond University, Gold Coast QLD
2 Gold Coast University Hospital and Health Service, Gold Coast QLD
Mark A Jones
3 University of Queensland, Brisbane QLD
Paulina Stehlik
Paul p glasziou.
Community‐based studies in five countries show consistent strong benefits from early rollouts of COVID‐19 vaccines
By the beginning of June 2021, almost 11% of the world’s population had received at least one dose of a coronavirus disease 2019 (COVID‐19) vaccine. 1 This represents an extraordinary scientific and logistic achievement — in 18 months, researchers, manufacturers and governments collaborated to produce and distribute vaccines that appear effective and acceptably safe in preventing COVID‐19 and its complications. 2 , 3
The initial randomised trials confirmed immunological responses and generated unbiased evidence of vaccine efficacy. They were conducted in selected populations with limited numbers of participants in high risk groups, such as older people and those with serious underlying medical conditions. 2 , 3 They provided sparse information on the impact of vaccination on transmission of severe acute respiratory syndrome coronavirus 2 (SARS‐CoV‐2), were too small to quantify rare but serious harms, and did not take account of the logistic obstacles encountered during the community‐wide rollout of new vaccines. While large cluster randomised trials could address some of these concerns, 4 large observational studies have used large linked routinely collected population datasets in five countries to address important knowledge gaps. 5 , 6 , 7 , 8 , 9
This article reviews findings from the initial real world studies and stresses that researchers in Australia currently do not have timely access to the linked Commonwealth and state datasets needed to perform such analyses.
Real world studies
In five countries (Israel, England, Scotland, Sweden and the United States) researchers have analysed routinely collected data to report the early outcomes of community‐wide vaccination programs with three of the first vaccines to reach market: the BNT162b2 mRNA (Pfizer–BioNTech), mRNA‐1273 (Moderna) and ChAdOx1 adenoviral vector (Oxford–AstraZeneca) vaccines. 5 , 6 , 7 , 8 , 9
At the time of writing, two of the articles (from the US and Sweden ) have not yet been peer reviewed, so details reported here may change after revisions to these reports. 8 , 9 There is a rapidly growing literature on the community impact of COVID‐19 and it has provided very consistent evidence of substantial vaccine effectiveness with the original (Wuhan) viral strain and the Alpha variant. An important focus of future work will be the effectiveness of existing vaccines against emerging viral variants.
The vaccination programs against COVID‐19 commenced in December 2020 in the study countries, so follow‐up is limited. Most of the investigators used rigorous designs and statistical methods to analyse linked routinely collected person‐level data from large community‐wide databases that tracked outcomes in vaccinated and unvaccinated individuals ( Box ). Importantly, allocation to vaccines was not by randomisation, and vaccinated and unvaccinated populations differed in respect of factors that were associated with both the probability of vaccination and with the severe outcomes of COVID‐19. Information that featured in most studies included demographic details, a vaccine register, results of laboratory polymerase chain reaction (PCR) testing, records of hospitalisation and death, and some geographic measures of social deprivation. In addition, the Israeli, US and Scottish studies included linkage to clinical records from which to quantify comorbidities. 5 , 6 , 8 The Israeli study included information on previous adherence to influenza vaccination schedules. 5
Characteristics of five real world community‐based studies of effectiveness of SARS‐CoV‐2 vaccines
BNT162b2 =Pfizer–BioNTech mRNA vaccine; ChAdOx1 = Oxford–AstraZeneca adenoviral vector vaccine; mRNA‐1273 = Moderna mRNA vaccine; NHS = National Health Service; PCR = polymerase chain reaction.
Study designs and adjustments for confounding
The studies used different approaches to adjust for confounding ( Box ). The most advanced design was used to analyse the linked data from members of the Clalit Health Services integrated health care organisation in Israel, which covers around 4.7 million people. 5 The investigators extracted data on matched cohorts of vaccinees and non‐vaccinated controls and analysed study endpoints using rules that emulated the steps taken in a randomised trial. 10 These steps minimised selection or measurement biases and controlled for potential confounders through precise 1:1 matching of vaccinated and non‐vaccinated subjects across seven domains. The investigators took the additional step of calibrating their statistical model against the results of the pivotal phase 3 randomised trial, which found no benefit during the first 2 weeks after vaccination. 2 In contrast, this observational study found lower rates of infection in the first 2 weeks after vaccination, which remained after matching for age and sex — illustrating the potential for confounding. Only after full matching on seven factors was this source of bias eliminated. 5
In England, investigators linked data from a national vaccine register to laboratory PCR swab results, emergency department admissions, demographic and ethnicity data, care home status, and deaths in participants aged 70 years and over ( Box ). 7 The first part was a test‐negative case–control design, which compared vaccination status in those who received a positive PCR swab result with contemporaneous controls who returned a negative result. That both cases and controls had been tested for SARS‐CoV‐2 should have controlled for clinical and behavioural factors that influence the probability of having a test. The second part of the study followed participants aged 80 years and over with a positive PCR test result and analysed them according to vaccination status. The investigators calculated adjusted hazard ratios for death up to and beyond 14 days from the first vaccine dose.
A study in Scotland used an unmatched cohort design comparing hospital admission for COVID‐19 in people who received either the Pfizer–BioNTech or Oxford–AstraZeneca vaccines with an unvaccinated control group. 6 The Oxford–AstraZeneca vaccine was given later to an older population. The study adjusted for age and sex, frequency of prior PCR tests and clinical risk groups extracted from linked health records. The statistical model generated unexpectedly strong protective effects of the vaccines on hospitalisation rates in the first 2 weeks after vaccination, indicating possible bias due to a healthy vaccinee effect.
In the US, researchers working within the Mayo Clinic health system used postcode and propensity scores (based on age, sex, race, ethnicity and records of PCR testing) to match a cohort of individuals who received the Pfizer–BioNTech or Moderna mRNA vaccine with unvaccinated controls, to measure impact on infections and hospitalisations. 8
A simple unmatched cohort design using linkage of routinely collected administrative data measured infection rates in a cohort who received the Pfizer–BioNTech vaccine in a single county in Sweden. 9 The unvaccinated population acted as controls ( Box ). Confounding adjustments in this study were limited to age and sex.
The Box summarises the results of these studies. All included at least one mRNA vaccine and the reductions in infections and hospitalisations were consistent and large. Two studies reported on mortality and the reductions were substantial, although based on small numbers of deaths in Israel. 5 , 7 The studies did not directly compare vaccines, but the Oxford–AstraZeneca vaccine appeared to perform as well as the mRNA vaccines in reducing hospitalisations.
Other approaches to estimating vaccine effectiveness
In the UK, over 600 000 volunteers using a COVID‐19 symptom mobile phone app recorded adverse events after vaccination with either the Pfizer–BioNTech or Oxford–AstraZeneca vaccine. 11 Based on post‐vaccination self‐reports of infections and after adjustment for age, sex, obesity and comorbidities, they estimated effectiveness rates of 60–70% beyond 21 days after administration of either vaccine.
Three studies measured the effectiveness of COVID‐19 vaccines in care home, health care and other frontline workers in the UK, Israel and the US. 12 , 13 , 14 These projects enrolled smaller numbers of participants than the community‐based studies but used similar designs and adjustment techniques. Importantly, workers in these settings undergo routine PCR testing for SARS‐CoV‐2, which enabled detection of asymptomatic infections. These studies also found large protective effects and a potential to reduce viral transmission. The latter possibility has been investigated directly in a study conducted in Scotland that showed that 14 days or more after health care workers received a second dose of vaccine, their household members had a 54% lower rate of COVID‐19 than individuals who shared households with non‐vaccinated health care workers. 15
Conclusions
We can draw important conclusions from these non‐randomised studies of vaccine effectiveness. Most importantly, the currently available COVID‐19 vaccines appear to be very effective in preventing severe complications and deaths from COVID‐19 in adults of all ages. Recent real world studies confirm that substantial protection extends to the Delta variant of SARS‐CoV‐2, although this requires two vaccine doses. 16 , 17 Follow‐up periods in all studies are relatively short, and these reports do not provide information on rare but serious adverse events, such as cerebral venous thrombosis. The use of sophisticated trial emulation methods in the Israeli study 5 replicated some key features of the pivotal randomised trial of the Pfizer–BioNTech vaccine, 2 particularly by controlling for an early healthy cohort effect that confounded the incompletely adjusted endpoint analyses. This design should prove useful in enabling direct head‐to‐head comparisons of effectiveness and safety of vaccines, the duration of their protective effects, the degree to which vaccines prevent transmission of viral variants, and the impact of vaccines on so‐called long COVID.
These studies exemplify the value of advanced analyses of large multiply linked routinely collected community datasets. This resource is not yet readily available to researchers in Australia due to continued lack of agreement on the governance of linked state and Commonwealth datasets. 18 While Australia’s current low rates of community transmission of SARS‐CoV‐2 reduce the feasibility of observational studies of vaccine effectiveness, the available data can provide important information on potential harms of vaccines. With continuing questions about the comparative safety of vaccines, the emergence of viral variants, the long term effects of COVID‐19 and the likelihood of future epidemics, it is essential that Australia urgently removes barriers to allowing prequalified researchers to safely access the linked de‐identified population datasets that are needed to expeditiously conduct the types of studies reviewed here.
Competing interests
No relevant disclosures.
Not commissioned; externally peer reviewed.
The unedited version of this article was published as a preprint on mja.com.au on 20 May 2021.

COMMENTS
The BNT162b2 COVID-19 vaccine developed by BioNTech and Pfizer is a lipid nanoparticle-formulated, nucleoside-modified RNA vaccine that encodes a prefusion membrane-anchored SARS-CoV-2 full-length spike protein. 9 It was the first vaccine approved by the US Food and Drug Association (FDA) and now it has been approved in many other countries. 10 ...
A two-dose regimen of BNT162b2 (30 μg per dose, given 21 days apart) was found to be safe and 95% effective against Covid-19. The vaccine met both primary efficacy end points, with more than a 99 ...
The 3 3 3 factorial design requires × × judgments of 27 cases. The effectiveness factor varied level of vaccine effec-tiveness for preventing COVID-19: 50%, 70%, or 90% protection. The three levels were chosen to reflect the effectiveness of common vaccines, with particular emphasis on influenza vaccines.
Safety and adverse effects of current COVID-19 vaccines. As shown in Table I, current vaccines have demonstrated considerable efficacy in diminishing mild, moderate and severe cases with a low risk of adverse events 21.For some of these vaccines [such as Convidicea (AD5-nCoV), Janssen (Ad26.COV2.S), Sinopharm (BBIBP-CorV), Covaxin (BBV152) and Sinovac (CoronaVac)], there is the information ...
The Coronavirus Efficacy (COVE) phase 3 trial was launched in late July 2020 to assess the safety and efficacy of the mRNA-1273 vaccine in preventing SARS-CoV-2 infection. An independent data and ...
The protective effects of vaccination and prior infection against severe Covid-19 are reviewed, with proposed directions for future research, including mucosal immunity and intermittent vaccine boo...
1. A phase 1/2 randomized, placebo-controlled, multicentre study to evaluate the safety and immunogenicity of COVID-19 vaccine in healthy adults. RBD SARS-CoV-2 HBsAg VLP vaccine, administered at two dose amounts 5 mcg and 25 mcg, by intramuscular injection by investigators during an in-clinic visit. RBD-HBsAg VLPs.
DRAFT Prepared by the SAGE Working Group on COVID-19 Vaccines 22 December 2020 3 . I Epidemiology . Coronavirus disease 2019 (COVID-19) is caused by SARS-CoV-2, a newly emergent coronavirus, that was first recognized in Wuhan, China, in December 2019. Globally, as of 19 December 2020, there
Our analyses indicate that vaccine effectiveness generally decreases over time against SARS-CoV-2 infections, hospitalisations, and mortality. The baseline vaccine effectiveness levels for the omicron variant were notably lower than for other variants. Therefore, other preventive measures (eg, face-mask wearing and physical distancing) might be necessary to manage the pandemic in the long term.
2020 has been a difficult year for all, but has seen 58 vaccines against severe acute respiratory syndrome coronavirus 2 (SARS-CoV-2) be developed and in clinical trials,1 with some vaccines reportedly having more than 90% efficacy against COVID-19 in clinical trials. This remarkable achievement is much-needed good news as COVID-19 cases are currently at their highest daily levels globally.2 ...
The development of effective COVID-19 vaccines, treatments and public health interventions alone has provided humanity with some hope for the future. This updated report once again brings a spotlight to the immense and tireless global research effort to control COVID-19. The global coordination and support for the world's leading scientists and
There is no question that the current vaccines are effective and safe. The risk of severe reaction to a COVID-19 jab, say researchers, is outweighed by the protection it offers against the deadly ...
To date, coronavirus disease 2019 (COVID-19) becomes increasingly fierce due to the emergence of variants. Rapid herd immunity through vaccination is needed to block the mutation and prevent the emergence of variants that can completely escape the immune surveillance. We aimed to systematically evaluate the effectiveness and safety of COVID-19 vaccines in the real world and to establish a ...
Despite questions remain about the impact of virus variants and the duration of the immune response, messenger RNA (mRNA)-based and adenoviral vectored vaccines have demonstrated an overall efficacy from 70 to 95% in both phase III trials and real life. In addition, all these vaccines also reduce the severe forms of the disease and might ...
Our results provide support for high effectiveness of BNT162b2 against hospital admissions up until around 6 months after being fully vaccinated, even in the face of widespread dissemination of the delta variant. Reduction in vaccine effectiveness against SARS-CoV-2 infections over time is probably primarily due to waning immunity with time rather than the delta variant escaping vaccine ...
These population-based databases have been used in previous studies on the long-term sequelae of COVID-19 infection, COVID-19 vaccines safety surveillance and effectiveness 3,6,38,39,40,41,42 ...
The effectiveness of vaccines against SARS-CoV-2 has been confirmed in real-world studies, 10,11 but high-quality real-world safety data on the messenger RNA (mRNA)-based Covid-19 vaccines ...
1. Introduction. Since declaration of the COVID-19 pandemic by the World Health Organization (WHO) on March 11, 2020 [1] more than 13.5 billion doses of COVID-19 vaccines have been administered worldwide [2].As of November 2023, at least 70.5 % of the world's population had received at least one dose of a COVID-19 vaccine [2].This unparalleled scenario underscores the pressing need for ...
2. Vaccination strategies. Many efforts have been directed towards the development of the vaccines against COVID-19, to avert the pandemic and most of the developing vaccine candidates have been using the S-protein of SARS-CoV-2 (Dhama et al., 2020).As of July 2, 2020, the worldwide SARS-CoV-2 vaccine landscape includes 158 vaccine candidates, out of which 135 are in the preclinical or the ...
the COVID-19 vaccine in the health workers (HWs), with a focus on hospital-based HWs. This document is intended to be used as a guidance document to support countries and institutions that are interested in conducting research on COVID-19 vaccine effectiveness in health workers. It outlines
The effectiveness against infection of COVID-19 vaccines waned considerably 5-8 months after primary vaccination, although it remained high, particularly among people younger than 55 years. Vaccine boosters were effective in restoring protection against infection and had a good safety profile in the community.
and manufacturing of COVID-19 vaccines: Background paper for the World Bank/CEPI financing COVID-19 vaccine development consultation on February 20, 2020. The Center for Policy Impact in Global Health. Duke ... Research led by the Center for Policy Impact in Global Health at Duke University has illustrated a valley of
Community‐based studies in five countries show consistent strong benefits from early rollouts of COVID‐19 vaccines. By the beginning of June 2021, almost 11% of the world's population had received at least one dose of a coronavirus disease 2019 (COVID‐19) vaccine. 1 This represents an extraordinary scientific and logistic achievement — in 18 months, researchers, manufacturers and ...
PDF | On May 10, 2024, Devika S Kumar and others published Innovations and Challenges in the Development of COVID-19 Vaccines for a Safer Tomorrow | Find, read and cite all the research you need ...
The effectiveness of the mRNA vaccines in preventing COVID-19 disease progression in 2021 set new expectations about the role of prevention interventions for the disease. Efficacy observed in the trials was more than 90%.1,2 The efficacy of other vaccines evaluated in large randomised trials, such as the Oxford-AstraZeneca (70%) and Sputnik V (91%) vaccines, have been criticised for elements ...
Semantic Scholar extracted view of "Hypothesis for COVID-19 Vaccine Detoxification" by Yang Pachanki. ... Semantic Scholar's Logo. Search 218,539,814 papers from all fields of science. Search. Sign In Create Free Account. DOI: 10.31031/ajhs.2023.02 ... AI-powered research tool for scientific literature, based at the Allen Institute for AI. ...