- Utility Menu


Michael J. Sandel
Anne t. and robert m. bass professor of government, the case against perfection: ethics in the age of genetic engineering.

Breakthroughs in genetics present us with a promise and a predicament. The promise is that we will soon be able to treat and prevent a host of debilitating diseases. The predicament is that our newfound genetic knowledge may enable us to manipulate our nature—to enhance our genetic traits and those of our children. Although most people find at least some forms of genetic engineering disquieting, it is not easy to articulate why. What is wrong with re-engineering our nature?
The Case against Perfection explores these and other moral quandaries connected with the quest to perfect ourselves and our children. Michael Sandel argues that the pursuit of perfection is flawed for reasons that go beyond safety and fairness. The drive to enhance human nature through genetic technologies is objectionable because it represents a bid for mastery and dominion that fails to appreciate the gifted character of human powers and achievements. Carrying us beyond familiar terms of political discourse, this book contends that the genetic revolution will change the way philosophers discuss ethics and will force spiritual questions back onto the political agenda.
In order to grapple with the ethics of enhancement, we need to confront questions largely lost from view in the modern world. Since these questions verge on theology, modern philosophers and political theorists tend to shrink from them. But our new powers of biotechnology make these questions unavoidable. Addressing them is the task of this book, by one of America’s preeminent moral and political thinkers.
“In the future, genetic manipulation of embryos is expected to have the potential to go beyond the treatment of diseases to improvements: children who are taller, more athletic, and have higher IQs… In The Case against Perfection , Michael Sandel argues that the unease many people feel about such manipulations have a basis in reason… This beautifully crafted little book…quickly and clearly lays out the key issues at stake.” —Gregory M. Lamb, The Christian Science Monitor
“Sandel worries that more genetic choice will undermine our appreciation of the gifted character of human life—our sense that the way we are is not solely the product of our own doing…. Many of us feel uneasy about such a future, without being quite able to say why. Michael Sandel’s graceful and intelligent new book, The Case against Perfection , is an extended effort to diagnose that unease.” —Carl Elliott, The New England Journal of Medicine
“ The Case against Perfection by Michael Sandel is a brief, concise, and dazzling argument by one of America’s foremost moral and political thinkers that brings you up to speed on the core ethical issues informing current debates about genetic engineering and stem cell research.” —Gabriel Gbadamosi, BBC Radio
“Given the vast gulf between progressive and conservative thinking, the time is ripe for a philosopher to take on the issues of biotechnology. And in The Case against Perfection Harvard’s Michael Sandel does just that, attempting to develop a new position on biotechnology, one that, like Sandel himself, is not easily identified as either left or right. A former member of the President’s Council on Bioethics, Sandel is uniquely well suited for this task, and to challenge the left to get its bearings on the brave new biology… Sandel poses an important challenge to contemporary progressives who have failed to grasp the importance of the emerging biopolitics.” —Jonathan Moreno, Democracy
“Just what exactly is wrong with an athlete tweaking his genes to perform better, if all the other athletes are doing it? And why shouldn't parents with the means to do so shape the genes of their future children? Many of us find these ideas disturbing, but it's difficult to articulate why. In The Case Against Perfection , political philosopher Michael Sandel, presents a moral explanation for this unease…. He makes the compelling case that genetic engineering to gain advantage for ourselves and our children is deeply disempowering, because it turns us away from the communal good, toward self-centered striving.” —Anne Harding, The Lancet
A “marvelous little book about the moral issues raised by genetic engineering and other forms of biotechnology…. The care with which Sandel examines arguments for and against various forms of biotechnology makes this an excellent primer on how to formulate and assess moral arguments…. The greatest strength of this book is Sandel’s understanding of how the Promethean aspiration to mastery erodes a sense of what he calls the ‘giftedness of life,’ and how the eclipse of this sense diminishes our humanity.” – Paul Lauritzen, Commonweal
“Sandel’s arguments ultimately speak to our gut-level qualms about enhancement; and his aim in fact is to give these qualms a coherent moral basis… His book in the end is more a lyrical plea for reverence and humility than a lawyer’s watertight ‘case against.’ …The ethicist Michael Sandel wants us…to think about where, in a hyper-competitive world, re-engineering our natures will ultimately lead.” —Michele Pridmore-Brown, The Times Literary Supplement
“Michael Sandel‘s dive into the sea of genetic engineering provides a great tasty gulp of contemporary ethical controversy. Quickly read, The Case Against Perfection is nonetheless dense with challenging quandaries, loaded with moral puzzles and filled with facts. An inveterate highlighter, I underlined half the book.” —John F. Kavanaugh, America
“Anyone who thinks our culture is too competitive and consumer-driven should find that Sandel’s diagnosis resonates. He provides not only a warning about the shape of the future, but equally an indictment of—or at least a call to examine—our individual moral lives and our contemporary social values. Those who support the practice of genetic enhancement argue that the technology is not substantially different from other forms of ‘enhancement’ we use to improve our lives and the lives of our children. Sandel agrees, but he does not base his argument on any particular distinction about the means of enhancement; rather he is deeply concerned about the underlying impetus of mastery and dominion.” —Debra Greenfield, Bioethics Forum
“For many years I have been ambivalent about reproductive innovations, from surrogate gestation to preimplantation screening for gender selection. After reading Sandel’s exceedingly elegant little book, The Case Against Perfection: Ethics in the Age of Genetic Engineering , I could finally put satisfactory names to core values implicit in my hesitation: acceptance and solidarity. I encountered Sandel’s book as a participant in the intellectual discourse about parenting. But the book’s greatest value to me was its validation of the commitments of solidarity expressed in my volunteer work on behalf of poor mothers and of acceptance implicit in my determination to mother a child with catastrophic mental illness.” —Anita L. Allen, The Chronicle of Higher Education
“In this short and provocative treatise, Sandel, who is professor of government at Harvard and a member of the President’s Council on Bioethics, takes on the question of why certain kinds of newly available genetic technologies make us uneasy…[his] book reminds us that the proper starting point for bioethics is not, ‘what should we do?’ but rather, ‘what kind of society do we want?’ And ‘what kind of people are we?’” —Faith McLellan, The Scientist
“In a highly readable, wise and little book titled The Case against Perfection: Ethics in the Age of Genetic Engineering , Michael Sandel argues that parents’ quest to create the ideal child reflects a drive for mastery and domination over life.” —Douglas Todd, The Vancouver Sun
“An illuminating ethical analysis of stem-cell research concludes this stellar work of public philosophy.” —Ray Olson, Booklist
“Sandel explores a paramount question of our era: how to extend the power and promise of biomedical science to overcome debility without compromising our humanity. His arguments are acute and penetrating, melding sound logic with compassion. We emerge from this book feeling edified and inspired.” —Jerome Groopman, Harvard Medical School, author of How Doctors Think
“We live in a world, says Michael Sandel, where ‘science moves faster than moral understanding.’ But thanks to Sandel, moral understanding is catching up. Cloning, stem cell research, performance-enhancing drugs, pills that make you stronger or taller: if some scientific development bothers you, but you can’t explain why, Michael Sandel will help you to figure out why you’re troubled. And then he’ll tell you whether you should be.” —Michael Kinsley
“Michael Sandel has engaged in a bioethical debate that has produced similar front lines in Germany and in the USA…. [He] is after a philosophically illuminating explanation of the injunction not to convert all that is technically do-able into marketable technologies…. [His] eloquently presented…opinion on the question of the desirability and permissibility of eugenic changes in the human organism rests on a well thought-out neo-Aristotelian position. This argumentative background gives the book a philosophical interest quite independent of the for-and-against of particular political decisions…. His analysis draws on the idea that eugenic practices undermine a ‘sense of giftedness’ that is indispensable for a civilized common life.” –Jürgen Habermas, preface to the German edition
Recent Publications
- Michael Sandel: ‘The energy of Brexiteers and Trump is born of the failure of elites'
- Market Reasoning as Moral Reasoning: Why Economists Should Re-engage with Political Philosophy
- The Moral Economy of Speculation: Gambling, Finance, and the Common Good
- What Isn’t for Sale?
- What Money Can't Buy: The Moral Limits of Markets
- Obama and Civic Idealism
Thank you for visiting nature.com. You are using a browser version with limited support for CSS. To obtain the best experience, we recommend you use a more up to date browser (or turn off compatibility mode in Internet Explorer). In the meantime, to ensure continued support, we are displaying the site without styles and JavaScript.
- View all journals
Genetic engineering articles from across Nature Portfolio
Genetic engineering is the act of modifying the genetic makeup of an organism. Modifications can be generated by methods such as gene targeting, nuclear transplantation, transfection of synthetic chromosomes or viral insertion. Selective breeding is not considered a form of genetic engineering.
Latest Research and Reviews
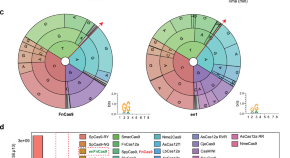
PAM-flexible Engineered FnCas9 variants for robust and ultra-precise genome editing and diagnostics
Francisella novicida Cas9 (FnCas9) has low cellular editing ability which limits its therapeutic utilities. Here, the authors rationally engineer the protein to develop enhanced FnCas9 (enFnCas9) variants with high on-target editing efficiency, high precision, broadened target range, and flexible base editing outcomes.
- Sundaram Acharya
- Asgar Hussain Ansari
- Debojyoti Chakraborty
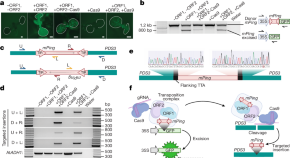
Transposase-assisted target-site integration for efficient plant genome engineering
Fusion of rice Pong transposase to the Cas9 or Cas12a programmable nucleases provides sequence-specific targeted insertion of enhancer elements, an open reading frame and gene expression cassette into the genome of the model plant Arabidopsis and crop soybean .
- Kaushik Panda
- R. Keith Slotkin
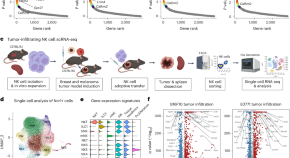
In vivo AAV–SB-CRISPR screens of tumor-infiltrating primary NK cells identify genetic checkpoints of CAR-NK therapy
The antitumor efficacy of chimeric antigen receptor natural killer cells is enhanced by genetic engineering.
- Paul A. Renauer
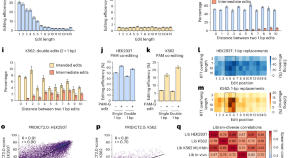
Machine learning prediction of prime editing efficiency across diverse chromatin contexts
A machine learning model for prime editing efficiency prediction takes into account chromatin context.
- Nicolas Mathis
- Ahmed Allam
- Gerald Schwank
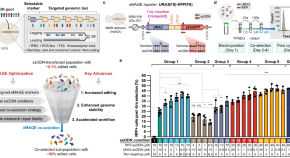
Enhanced eMAGE applied to identify genetic factors of nuclear hormone receptor dysfunction via combinatorial gene editing
Technologies that generate precise combinatorial genome modifications are well suited to dissect the polygenic basis of complex phenotypes and engineer synthetic genomes. Here the authors systematically optimize eMAGE for enhanced editing efficiency and editing distance and apply these advances to identify genetic factors of nuclear hormone dysfunction.
- Peter N. Ciaccia
- Zhuobin Liang
- Farren J. Isaacs
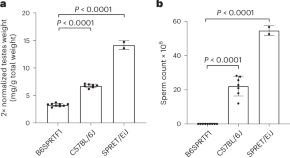
Naturally sterile Mus spretus hybrids are suitable for the generation of pseudopregnant embryo transfer recipients
Preece et al. show that B6SPRTF1 hybrid males between C57BL/6J and Mus spretus are suitable for the generation of pseudopregnant female mice for embryo transfer. By providing an alternative to vasectomized males, the method shows clear 3R benefits.
- Chris Preece
- Daniel Biggs
- Benjamin Davies
News and Comment
Rna editing with crispr.
- Petra Gross
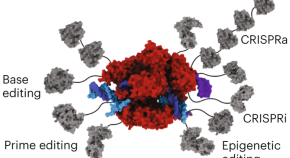
The genetic engineering Swiss army knife
In molecular biology, few molecules have had as profound an impact as Cas9. Madeleine King, Kayla Perry, Mitchell McAndrew and Audrone Lapinaite discuss how this multifunctional molecular tool of genetic engineering is revolutionizing multiple fields.
- Madeleine B. King
- Kayla N. Perry
- Audrone Lapinaite
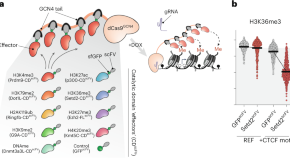
Chromatin modifications integrate cis genomic context to instruct transcriptional outputs
By developing a modular system for precision epigenome editing, we were able to delineate the causal and quantitative role of chromatin modifications in transcription regulation. The precise effect of chromatin modifications is influenced by multiple contextual factors, including the underlying DNA sequence, transcription factor occupancy and genomic positioning.
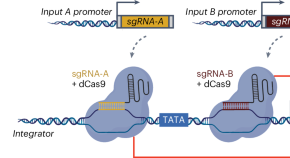
Advancing programmable gene expression in plants using CRISPRi-based Boolean gates
To advance the toolset for controlling plant gene expression, we developed a CRISPR interference-based platform for the construction of synthetic Boolean logic gates that is functional in multiple plant species. These genetic circuits are programmable and reversible in nature, which will enable spatiotemporal control of plant responses to dynamic cues.
A MEGA RNA-editing tool
In this Tools of the Trade article, Victor Tieu describes the development of MEGA, a platform that exploits the RNA-targeting capability of CRISPR–Cas13d and demonstrates its use to improve the anti-tumour activity of CAR T cells.
- Victor Tieu
Quest: my postdoc home
For a postdoctoral fellowship, it’s advisable to be selective about lab choice and to be clear about expectations.
- Vivien Marx
Quick links
- Explore articles by subject
- Guide to authors
- Editorial policies

- DSpace@MIT Home
- Synthetic Biology
- Communications
Cellular computation and communications using engineered genetic regulatory networks
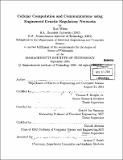
Other Contributors
Terms of use, description, date issued, collections.
- Doctoral Theses
Show Statistical Information
- Search Menu
- Sign in through your institution
- Volume 12, Issue 1, 2024 (In Progress)
- Volume 11, Issue 1, 2023
- Advance articles
- Editor's Choice
- Virtual Issues
- Clinical Briefs
- ISEMPH Prizes
- Author Guidelines
- Submission Site
- Open Access
- Calls for Papers
- Why submit?
- About Evolution, Medicine, and Public Health
- About the International Society for Evolution, Medicine and Public Health
- Editorial Board
- Advertising and Corporate Services
- Journals Career Network
- Self-Archiving Policy
- For Reviewers
- Journals on Oxford Academic
- Books on Oxford Academic
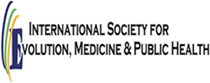
Article Contents
Introduction, human enhancement, genetic engineering, conclusions.
- < Previous
Human enhancement: Genetic engineering and evolution
- Article contents
- Figures & tables
- Supplementary Data
Mara Almeida, Rui Diogo, Human enhancement: Genetic engineering and evolution, Evolution, Medicine, and Public Health , Volume 2019, Issue 1, 2019, Pages 183–189, https://doi.org/10.1093/emph/eoz026
- Permissions Icon Permissions
Genetic engineering opens new possibilities for biomedical enhancement requiring ethical, societal and practical considerations to evaluate its implications for human biology, human evolution and our natural environment. In this Commentary, we consider human enhancement, and in particular, we explore genetic enhancement in an evolutionary context. In summarizing key open questions, we highlight the importance of acknowledging multiple effects (pleiotropy) and complex epigenetic interactions among genotype, phenotype and ecology, and the need to consider the unit of impact not only to the human body but also to human populations and their natural environment (systems biology). We also propose that a practicable distinction between ‘therapy’ and ‘enhancement’ may need to be drawn and effectively implemented in future regulations. Overall, we suggest that it is essential for ethical, philosophical and policy discussions on human enhancement to consider the empirical evidence provided by evolutionary biology, developmental biology and other disciplines.
Lay Summary: This Commentary explores genetic enhancement in an evolutionary context. We highlight the multiple effects associated with germline heritable genetic intervention, the need to consider the unit of impact to human populations and their natural environment, and propose that a practicable distinction between ‘therapy’ and ‘enhancement’ is needed.
There are countless examples where technology has contributed to ameliorate the lives of people by improving their inherent or acquired capabilities. For example, over time, there have been biomedical interventions attempting to restore functions that are deficient, such as vision, hearing or mobility. If we consider human vision, substantial advances started from the time spectacles were developed (possibly in the 13th century), continuing in the last few years, with researchers implanting artificial retinas to give blind patients partial sight [ 1–3 ]. Recently, scientists have also successfully linked the brain of a paralysed man to a computer chip, which helped restore partial movement of limbs previously non-responsive [ 4 , 5 ]. In addition, synthetic blood substitutes have been created, which could be used in human patients in the future [ 6–8 ].
The progress being made by technology in a restorative and therapeutic context could in theory be applied in other contexts to treat non-pathological conditions. Many of the technologies and pharmaceutical products developed in a medical context to treat patients are already being used by humans to ‘enhance’ some aspect of their bodies, for example drugs to boost brain power, nutritional supplements, brain stimulating technologies to control mood or growth hormones for children of short stature. Assistive technology for disabled people, reproductive medicine and pharmacology, beside their therapeutic and restorative use, have a greater potential for human ‘enhancement’ than currently thought. There are also dual outcomes as some therapies can have effects that amount to an enhancement as for example, the artificial legs used by the South African sprinter Oscar Pistorius providing him with a competitive advantage.
This commentary will provide general ethical considerations on human enhancement, and within the several forms of so-called human biomedical enhancement, it will focus on genetic engineering, particularly on germline (heritable) genetic interventions and on the insights evolutionary biology can provide in rationalizing its likely impact. These insights are a subject often limited in discussions on genetic engineering and human enhancement in general, and its links to ethical, philosophical and policy discussions, in particular [ 9 ]. The rapid advances in genetic technology make this debate very topical. Moreover, genes are thought to play a very substantial role in biological evolution and development of the human species, thus making this a topic requiring due consideration. With this commentary, we explore how concepts based in evolutionary biology could contribute to better assess the implications of human germline modifications, assuming they were widely employed. We conclude our brief analysis by summarizing key issues requiring resolution and potential approaches to progress them. Overall, the aim is to contribute to the debate on human genetic enhancement by looking not only at the future, as it is so often done, but also at our evolutionary past.
The noun ‘enhancement’ comes from the verb ‘enhance’, meaning ‘to increase or improve’. The verb enhance can be traced back to the vulgar Latin inaltiare and late Latin inaltare (‘raise, exalt’), from ‘ altare ’ (‘make high’) and altus (‘high’), literally ‘grown tall’. For centuries human enhancement has populated our imagination outlined by stories ranging from the myths of supernormal strengths and eternal life to the superpowers illustrated by the 20th century comic books superheroes. The desire of overcoming normal human capacities and the transformation to an almost ‘perfect’ form has been part of the history of civilization, extending from arts and religion to philosophy. The goal of improving the human condition and health has always been a driver for innovation and biomedical developments.
In the broadest sense, the process of human enhancement can be considered as an improvement of the ‘limitations’ of a ‘natural version’ of the human species with respect to a specific reference in time, and to different environments, which can vary depending on factors such as, for example, climate change. The limitations of the human condition can be physical and/or mental/cognitive (e.g. vision, strength or memory). This poses relevant questions of what a real or perceived human limitation is in the environment and times in which we are living and how it can be shifted over time considering social norms and cultural values of modern societies. Besides, the impact that overcoming these limitations will have on us humans, and the environment, should also be considered. For example, if we boost the immune system of specific people, this may contribute to the development/evolution of more resistant viruses and bacteria or/and lead to new viruses and bacteria to emerge. In environmental terms, enhancing the longevity of humans could contribute to a massive increase in global population, creating additional pressures on ecosystems already under human pressure.
Two decades ago, the practices of human enhancement have been described as ‘biomedical interventions that are used to improve human form or functioning beyond what is necessary to restore or sustain health’ [ 10 ]. The range of these practices has now increased with technological development, and they are ‘any kind of genetic, biomedical, or pharmaceutical intervention aimed at improving human dispositions, capacities, or well-being, even if there is no pathology to be treated’ [ 11 ]. Practices of human enhancement could be visualized as upgrading a ‘system’, where interventions take place for a better performance of the original system. This is far from being a hypothetical situation. The rapid progress within the fields of nanotechnology, biotechnology, information technology and cognitive science has brought back discussions about the evolutionary trajectory of the human species by the promise of new applications which could provide abilities beyond current ones [ 12 , 13 ]. If such a possibility was consciously embraced and actively pursued, technology could be expected to have a revolutionary interference with human life, not just helping humans in achieving general health and capabilities commensurate with our current ones but helping to overcome human limitations far beyond of what is currently possible for human beings. The emergence of new technologies has provided a broader range of potential human interventions and the possibility of transitioning from external changes to our bodies (e.g. external prosthesis) to internal ones, especially when considering genetic manipulation, whose changes can be permanent and transmissible.
The advocates of a far-reaching human enhancement have been referred to as ‘transhumanists’. In their vision, so far, humans have largely worked to control and shape their exterior environments (niche construction) but with new technologies (e.g. biotechnology, information technology and nanotechnology) they will soon be able to control and fundamentally change their own bodies. Supporters of these technologies agree with the possibility of a more radical interference in human life by using technology to overcome human limitations [ 14–16 ], that could allow us to live longer, healthier and even happier lives [ 17 ]. On the other side, and against this position, are the so-called ‘bioconservatives’, arguing for the conservation and protection of some kind of ‘human essence’, with the argument that it exists something intrinsically valuable in human life that should be preserved [ 18 , 19 ].
There is an ongoing debate between transhumanists [ 20–22 ] and bioconservatives [ 18 , 19 , 23 ] on the ethical issues regarding the use of technologies in humans. The focus of this commentary is not centred on this debate, particularly because the discussion of these extreme, divergent positions is already very prominent in the public debate. In fact, it is interesting to notice that the ‘moderate’ discourses around this topic are much less known. In a more moderate view, perhaps one of the crucial questions to consider, independently of the moral views on human enhancement, is whether human enhancement (especially if considering germline heritable genetic interventions) is a necessary development, and represents an appropriate use of time, funding and resources compared to other pressing societal issues. It is crucial to build space for these more moderate, and perhaps less polarized voices, allowing the consideration of other positions and visions beyond those being more strongly projected so far.
Ethical and societal discussions on what constitutes human enhancement will be fundamental to support the development of policy frameworks and regulations on new technological developments. When considering the ethical implications of human enhancement that technology will be available to offer now and in the future, it could be useful to group the different kinds of human enhancements in the phenotypic and genetic categories: (i) strictly phenotypic intervention (e.g. ranging from infrared vision spectacles to exoskeletons and bionic limbs); (ii) somatic, non-heritable genetic intervention (e.g. editing of muscle cells for stronger muscles) and (iii) germline, heritable genetic intervention (e.g. editing of the C–C chemokine receptor type 5 (CCR5) gene in the Chinese baby twins, discussed later on). These categories of enhancement raise different considerations and concerns and currently present different levels of acceptance by our society. The degree of ethical, societal and environmental impacts is likely to be more limited for phenotypic interventions (i) but higher for genetic interventions (ii and iii), especially for the ones which are transmissible to future generations (iii).
The rapid advances in technology seen in the last decades, have raised the possibility of ‘radical enhancement’, defined by Nicholas Agar, ‘as the improvement of human attributes and abilities to levels that greatly exceed what is currently possible for human beings’ [ 24 ]. Genetic engineering offers the possibility of such an enhancement by providing humans a profound control over their own biology. Among other technologies, genetic engineering comprises genome editing (also called gene editing), a group of technologies with the ability to directly modify an organism’s DNA through a targeted intervention in the genome (e.g. insertion, deletion or replacement of specific genetic material) [ 25 ]. Genome editing is considered to achieve much greater precision than pre-existing forms of genetic engineering. It has been argued to be a revolutionary tool due to its efficiency, reducing cost and time. This technology is considered to have many applications for human health, in both preventing and tackling disease. Much of the ethical debate associated with this technology concerns the possible application of genome editing in the human germline, i.e. the genome that can be transmitted to following generations, be it from gametes, a fertilized egg or from first embryo divisions [ 26–28 ]. There has been concern as well as enthusiasm on the potential of the technology to modify human germline genome to provide us with traits considered positive or useful (e.g. muscle strength, memory and intelligence) in the current and future environments.
Genetic engineering: therapy or enhancement and predictability of outcomes
To explore some of the possible implications of heritable interventions we will take as an example the editing (more specifically ‘deletion’ using CRISPR genome editing technology) of several base pairs of the CCR5 gene. Such intervention was practised in 2018 in two non-identical twin girls born in China. Loss of function mutations of the CCR5 had been previously shown to provide resistance to HIV. Therefore, the gene deletion would be expected to protect the twin baby girls from risk of transmission of HIV which could have occurred from their father (HIV-positive). However, the father had the infection kept under control and the titre of HIV virus was undetectable, which means that risk of transmission of HIV infection to the babies was negligible [ 29 ].
From an ethical ground, based on current acceptable practices, this case has been widely criticized by the scientific community beside being considered by many a case of human enhancement intervention rather than therapy [ 29 , 30 ]. One of the questions this example helps illustrate is that the ethical boundary between a therapy that ‘corrects’ a disorder by restoring performance to a ‘normal’ scope, and an intervention that ‘enhances’ human ability outside the accepted ‘normal’ scope, is not always easy to draw. For the sake of argument, it could be assumed that therapy involves attempts to restore a certain condition of health, normality or sanity of the ‘natural’ condition of a specific individual. If we take this approach, the question is how health, normality and sanity, as well as natural per se, are defined, as the meaning of these concepts shift over time to accommodate social norms and cultural values of modern societies. It could be said that the difficulty of developing a conceptual distinction between therapy and enhancement has always been present. However, the potential significance of such distinction is only now, with the acceleration and impact of technological developments, becoming more evident.
Beyond ethical questions, a major problem of this intervention is that we do not (yet?) know exactly the totality of the effects that the artificial mutation of the CCR5 may have, at both the genetic and phenotypic levels. This is because we now know that, contrary to the idea of ‘one gene-one trait’ accepted some decades ago, a gene—or its absence—can affect numerous traits, many of them being apparently unrelated (a phenomenon also known as pleiotropy). That is, due to constrained developmental interactions, mechanisms and genetic networks, a change in a single gene can result in a cascade of multiple effects [ 31 ]. In the case of CCR5, we currently know that the mutation offers protection against HIV infection, and also seems to increase the risk of severe or fatal reactions to some infectious diseases, such as the influenza virus [ 32 ]. It has also been observed that among people with multiple sclerosis, the ones with CCR5 mutation are twice as likely to die early than are people without the mutation [ 33 ]. Some studies have also shown that defective CCR5 can have a positive effect in cognition to enhance learning and memory in mice [ 34 ]. However, it’s not clear if this effect would be translated into humans. The example serves to illustrate that, even if human enhancement with gene editing methods was considered ethically sound, assessing the totality of its implications on solid grounds may be difficult to achieve.
Genetic engineering and human evolution: large-scale impacts
Beyond providing the opportunity of enhancing human capabilities in specific individuals, intervening in the germline is likely to have an impact on the evolutionary processes of the human species raising questions on the scale and type of impacts. In fact, the use of large-scale genetic engineering might exponentially increase the force of ‘niche construction’ in human evolution, and therefore raise ethical and practical questions never faced by our species before. It has been argued that natural selection is a mechanism of lesser importance in the case of current human evolution, as compared to other organisms, because of advances in medicine and healthcare [ 35 ]. According to such a view, among many others advances, natural selection has been conditioned by our ‘niche-construction’ ability to improve healthcare and access to clean water and food, thus changing the landscape of pressures that humans have been facing for survival. An underlying assumption or position of the current debate is that, within our human species, the force of natural selection became minimized and that we are somehow at the ‘end-point’ of our evolution [ 36 ]. If this premise holds true, one could argue that evolution is no longer a force in human history and hence that any human enhancement would not be substituting itself to human evolution as a key driver for future changes.
However, it is useful to remember that, as defined by Darwin in his book ‘On the Origin of the Species’, natural selection is a process in which organisms that happen to be ‘better’ adapted to a certain environment tend to have higher survival and/or reproductive rates than other organisms [ 37 ]. When comparing human evolution to human genetic enhancement, an acceptable position could be to consider ethically sound those interventions that could be replicated naturally by evolution, as in the case of the CCR5 gene. Even if this approach was taken, however, it is important to bear in mind that human evolution acts on human traits sometimes increasing and sometimes decreasing our biological fitness, in a constant evolutionary trade-off and in a contingent and/or neutral—in the sense of not ‘progressive’—process. In other worlds, differently from genetic human enhancement, natural selection does not ‘ aim ’ at improving human traits [ 38 ]. Human evolution and the so-called genetic human enhancement would seem therefore to involve different underlying processes, raising several questions regarding the implications and risks of the latter.
But using genetic engineering to treat humans has been proposed far beyond the therapeutic case or to introduce genetic modifications known to already occur in nature. In particular, when looking into the views expressed on the balance between human evolution and genetic engineering, some argue that it may be appropriate to use genetic interventions to go beyond what natural selection has contributed to our species when it comes to eradicate vulnerabilities [ 17 ]. Furthermore, when considering the environmental, ecological and social issues of contemporary times, some suggest that genetic technologies could be crucial tools to contribute to human survival and well-being [ 20–22 ]. The possible need to ‘engineer’ human traits to ensure our survival could include the ability to allow our species to adapt rapidly to the rate of environmental change caused by human activity, for which Darwinian evolution may be too slow [ 39 ]. Or, for instance, to support long-distance space travel by engineering resistance to radiation and osteoporosis, along with other conditions which would be highly advantageous in space [ 40 ].
When considering the ethical and societal merits of these propositions, it is useful to consider how proto-forms of enhancement has been approached by past human societies. In particular, it can be argued that humans have already employed—as part of our domestication/‘selective breeding’ of other animals—techniques of indirect manipulation of genomes on a relatively large scale over many millennia, albeit not on humans. The large-scale selective breeding of plants and animals over prehistoric and historic periods could be claimed to have already shaped some of our natural environment. Selective breeding has been used to obtain specific characteristics considered useful at a given time in plants and animals. Therefore, their evolutionary processes have been altered with the aim to produce lineages with advantageous traits, which contributed to the evolution of different domesticated species. However, differently from genetic engineering, domestication possesses inherent limitations in its ability to produce major transformations in the created lineages, in contrast with the many open possibilities provided by genetic engineering.
When considering the impact of genetic engineering on human evolution, one of questions to be considered concerns the effects, if any, that genetic technology could have on the genetic pool of the human population and any implication on its resilience to unforeseen circumstances. This underlines a relevant question associated with the difference between ‘health’ and biological fitness. For example, a certain group of animals can be more ‘healthy’—as domesticated dogs—but be less biologically ‘fit’ according to Darwin’s definition. Specifically, if such group of animals are less genetically diverse than their ancestors, they could be less ‘adaptable’ to environmental changes. Assuming that, the human germline modification is undertaken at a global scale, this could be expected to have an effect, on the distribution of genetically heritable traits on the human population over time. Considering that gene and trait distributions have been changing under the processes of evolution for billions of years, the impact on evolution will need to be assessed by analysing which genetic alterations have been eventually associated with specific changes within the recent evolutionary history of humans. On this front, a key study has analysed the implications of genetic engineering on the evolutionary biology of human populations, including the possibility of reducing human genetic diversity, for instance creating a ‘biological monoculture’ [ 41 ]. The study argued that genetic engineering will have an insignificant impact on human diversity, while it would likely safeguard the capacity of human populations to deal with disease and new environmental challenges and therefore, ensure the health and longevity of our species [ 41 ]. If the findings of this study were considered consistent with other knowledge and encompassing, the impact of human genetic enhancements on the human genetic pool and associated impacts could be considered secondary aspects. However, data available from studies on domestication strongly suggests that domestication of both animals and plans might lead to not only decreased genetic diversity per se, but even affect patterns of variation in gene expression throughout the genome and generally decreased gene expression diversity across species [ 42–44 ]. Given that, according to recent studies within the field of biological anthropology recent human evolution has been in fact a process of ‘self-domestication’ [ 45 ], one could argue that studies on domestication could contribute to understanding the impacts of genetic engineering.
Beyond such considerations, it is useful to reflect on the fact that human genetic enhancement could occur on different geographical scales, regardless of the specific environment and geological periods in which humans are living and much more rapidly than in the case of evolution, in which changes are very slow. If this was to occur routinely and on a large scale, the implications of the resulting radical and abrupt changes may be difficult to predict and its impacts difficult to manage. This is currently highlighted by results of epigenetics studies, and also of the microbiome and of the effects of pollutants in the environment and their cumulative effect on the development of human and non-human organisms alike. Increasingly new evidence indicates a greater interdependence between humans and their environments (including other microorganisms), indicating that modifying the environment can have direct and unpredictable consequences on humans as well. This highlight the need of a ‘systems level’ approach. An approach in which the ‘bounded body’ of the individual human as a basic unit of biological or social action would need to be questioned in favour of a more encompassing and holistic unit. In fact, within biology, there is a new field, Systems Biology, which stresses the need to understand the role that pleiotropy, and thus networks at multiple levels—e.g. genetic, cellular, among individuals and among different taxa—play within biological systems and their evolution [ 46 ]. Currently, much still needs to be understood about gene function, its role in human biological systems and the interaction between genes and external factors such as environment, diet and so on. In the future if we do choose to genetically enhance human traits to levels unlikely to be achieved by human evolution, it would be crucial to consider if and how our understanding of human evolution enable us to better understand the implications of genetic interventions.
New forms of human enhancement are increasingly coming to play due to technological development. If phenotypic and somatic interventions for human enhancement pose already significant ethical and societal challenges, germline heritable genetic intervention, require much broader and complex considerations at the level of the individual, society and human species as a whole. Germline interventions associated with modern technologies are capable of much more rapid, large-scale impacts and seem capable of radically altering the balance of humans with the environment. We know now that beside the role genes play on biological evolution and development, genetic interventions can induce multiple effects (pleiotropy) and complex epigenetics interactions among genotype, phenotype and ecology of a certain environment. As a result of the rapidity and scale with which such impact could be realized, it is essential for ethical and societal debates, as well as underlying scientific studies, to consider the unit of impact not only to the human body but also to human populations and their natural environment (systems biology). An important practicable distinction between ‘therapy’ and ‘enhancement’ may need to be drawn and effectively implemented in future regulations, although a distinct line between the two may be difficult to draw.
In the future if we do choose to genetically enhance human traits to levels unlikely to be achieved by human evolution, it would be crucial to consider if and how our understanding of humans and other organisms, including domesticated ones, enable us to better understand the implications of genetic interventions. In particular, effective regulation of genetic engineering may need to be based on a deep knowledge of the exact links between phenotype and genotype, as well the interaction of the human species with the environment and vice versa .
For a broader and consistent debate, it will be essential for technological, philosophical, ethical and policy discussions on human enhancement to consider the empirical evidence provided by evolutionary biology, developmental biology and other disciplines.
This work was supported by Fundação para a Ciência e a Tecnologia (FCT) of Portugal [CFCUL/FIL/00678/2019 to M.A.].
Conflict of interest : None declared.
Pham P , Roux S , Matonti F et al. Post-implantation impedance spectroscopy of subretinal micro-electrode arrays, OCT imaging and numerical simulation: towards a more precise neuroprosthesis monitoring tool . J Neural Eng 2013 ; 10 : 046002 .
Google Scholar
Maghami MH , Sodagar AM , Lashay A et al. Visual prostheses: the enabling technology to give sight to the blind . J Ophthal Vis Res 2014 ; 9 : 494 – 505 .
Weitz AC , Nanduri D , Behrend MR et al. Improving the spatial resolution of epiretinal implants by increasing stimulus pulse duration . Sci Transl Med 2015 ; 7 : 318ra203.
Bouton CE , Shaikhouni A , Annetta NV et al. Restoring cortical control of functional movement in a human with quadriplegia . Nature 2016 ; 533 : 247 – 50 .
Geddes L. First paralysed person to be ‘reanimated’ offers neuroscience insights. Technique moves man’s arm by decoding his thoughts and electrically stimulating his own muscles . Nat News 2016 ; 533 .
Squires JE. Artificial blood . Science 2002 ; 295 : 1002 – 5 .
Lowe KC. Blood substitutes: from chemistry to clinic . J Mater Chem 2006 ; 16 : 4189 – 96 .
Moradi S , Jahanian-Najafabadi A , Roudkenar MH. Artificial blood substitutes: first steps on the long route to clinical utility . Clin Med Insights Blood Disord 2016 ; 9 : 33 – 41 .
Powell R , Kahane G , Savulescu J. Evolution, genetic engineering, and human enhancement . Philos Technol 2012 ; 25 : 439 – 58 .
Parens E (ed.). Enhancing Human Traits: Ethical and Social Implications . Washington, DC : Georgetown University Press , 1998 .
Google Preview
Giubilini A , Sanyal S. Challenging human enhancement. In: Clarke S , Savulescu J , Coady T et al. (eds). The Ethics of Human Enhancement: Understanding the Debate . Oxford : Oxford University Press , 2016 .
Elliott C. Better Than Well: American Medicine Meets the American Dream . New York, NY : WWW Norton & Company, Inc ., 2003 .
Kramer P. Listening to Prozac . London : Fourth Estate , 1994 .
Moravec H. Mind Children: The Future of Robot and Human Intelligence . Cambridge : Harvard University Press , 1990 .
Bostrom N. Human genetic enhancements: a transhumanist perspective . J Value Inq 2003 ; 37 : 493 – 506 .
Kurzweil R. The Singularity is Near: When Humans Transcend Biology . New York, NY : Viking , 2005 .
Harris J. Enhancing Evolution: The Ethical Case for Making Better People . Princeton, NJ : Princeton University Press , 2010 .
Fukuyama F. Our Posthuman Future: Consequences of the Biotechnology Revolution . New York, NY : Picador , 2002 .
Sandel M. The Case Against Perfection: Ethics in the Age of Genetic Engineering . Cambridge : The Belknap Press of Harvard University Press , 2007 .
Savulescu J , Persson I. The perils of cognitive enhancement and the urgent imperative to enhance the moral character of humanity . J Appl Philos 2008 ; 25 : 162 – 77 .
Buchanan A. Beyond Humanity . Oxford : Oxford University Press , 2011 .
Persson I , Savulescu J. Moral enhancement, freedom, and the god machine . Monist 2012 ; 95 : 399 – 421 .
Leon K. Ageless bodies, happy souls: biotechnology and the pursuit of perfection . New Atlantis 2003 ; 1 : 9 – 28 .
Agar N. Humanity’s End: Why We Should Reject Radical Enhancement . Cambridge : MIT Press , 2010 .
Gaj T , Gersbach CA , Barbas CF III ,. ZFN, TALEN, and CRISPR/Cas based methods for genome engineering . Trends Biotechnol 2013 ; 3 : 397 – 405 .
Baltimore D , Berg P , Botchan M et al. Biotechnology. A prudent path forward for genomic engineering and germline gene modification . Science 2015 ; 348 : 36 – 8 .
Otieno MO. CRISPR/Cas9 human genome editing: challenges, ethical concerns and implications . J Clin Res Bioeth 2015 ; 6 : 253 .
Ishii T. Germline genome-editing research and its socio-ethical implications . Trends Mol Med 2015 ; 21 : 473 – 81 .
Bionews.org.uk. First Genome-edited Babies: A Very Different Perception of Ethics , 2018 . https://www.bionews.org.uk/page_140060 (27 August 2019, date last accessed).
Cyranoski D. CRISPR-baby scientist fails to satisfy his critics . Nat News 2018 ; 564 : 13 – 4 .
Galis F , Metz JA. Evolutionary novelties: the making and breaking of pleiotropic constraints . Integr Comp Biol 2007 ; 47 : 409 – 19 .
Falcon A , Cuevas MT , Rodriguez-Frandsen A et al. CCR5 deficiency predisposes to fatal outcome in influenza virus infection . J Gen Virol 2015 ; 96 : 2074 – 8 .
Gade-Andavolu R , Comings DE , MacMurray J et al. Association of CCR5 Δ32 deletion with early death in multiple sclerosis . Genet Med 2004 ; 6 : 126 – 31 .
Zhou M , Greenhill S , Huang S et al. CCR5 is a suppressor for cortical plasticity and hippocampal learning and memory . eLife 2016 ; 5 : e20985 .
Tibayrenc M , Ayala FJ (eds). On Human Nature: Biology, Psychology, Ethics, Politics, and Religion . London : Academic Press , 2017 .
Baldi P. The Shattered Self: The End of Natural Evolution . Cambridge : MIT Press , 2001 .
Darwin C. On the Origin of Species by Means of Natural Selection, or, the Preservation of Favoured Races in the Struggle for Life . London : J. Murray , 1859 .
Gould SJ. The Structure of Evolutionary Theory . Belknap, NY : Harvard University Press , 2002 .
Rees M. Our Final Century: Will the Humans Race Survive the Twenty-first Century? Eastbourne : Gardners Books , 2003 .
Nuffield Council on Bioethics. Genome Editing: An Ethical Review . London : Nuffield Council on Bioethics , 2016 .
Powell R. The evolutionary biological implications of human genetic engineering . J Med Philos 2012 ; 37 : 204 – 26 .
Liu W , Chen L , Zhang S et al. Decrease of gene expression diversity during domestication of animals and plants . BMC Evol Biol 2019 ; 19 : 1 – 11 .
Fages A , Hanghøj K , Khan N et al. Tracking five millennia of horse management with extensive ancient genome time series . Cell 2019 ; 177 : 1419 – 35 .
Zhang J , Wang X , Yao J et al. Effect of domestication on the genetic diversity and structure of Saccharina japonica populations in China . Sci Rep 2017 ; 7 : 42158 .
Theofanopoulou C , Gastaldon S , O’Rourke T et al. Self-domestication in Homo sapiens : insights from comparative genomics . PLoS One 2018 ; 13 : e0196700 .
Capra F , Luisi PL. The Systems View of Life . Cambridge : Cambridge University Press , 2014
- genetic engineering
Month: | Total Views: |
---|---|
September 2019 | 51 |
October 2019 | 571 |
November 2019 | 512 |
December 2019 | 474 |
January 2020 | 492 |
February 2020 | 712 |
March 2020 | 680 |
April 2020 | 615 |
May 2020 | 609 |
June 2020 | 599 |
July 2020 | 605 |
August 2020 | 632 |
September 2020 | 842 |
October 2020 | 1,322 |
November 2020 | 1,583 |
December 2020 | 1,688 |
January 2021 | 1,536 |
February 2021 | 2,019 |
March 2021 | 3,077 |
April 2021 | 2,893 |
May 2021 | 2,223 |
June 2021 | 1,434 |
July 2021 | 906 |
August 2021 | 911 |
September 2021 | 1,393 |
October 2021 | 2,158 |
November 2021 | 2,377 |
December 2021 | 1,736 |
January 2022 | 1,315 |
February 2022 | 1,783 |
March 2022 | 2,522 |
April 2022 | 1,946 |
May 2022 | 1,552 |
June 2022 | 789 |
July 2022 | 675 |
August 2022 | 486 |
September 2022 | 919 |
October 2022 | 1,462 |
November 2022 | 1,427 |
December 2022 | 1,216 |
January 2023 | 1,372 |
February 2023 | 1,740 |
March 2023 | 2,320 |
April 2023 | 2,195 |
May 2023 | 1,646 |
June 2023 | 1,066 |
July 2023 | 940 |
August 2023 | 1,070 |
September 2023 | 1,283 |
October 2023 | 1,953 |
November 2023 | 1,886 |
December 2023 | 1,684 |
January 2024 | 1,871 |
February 2024 | 2,312 |
March 2024 | 2,729 |
April 2024 | 2,752 |
May 2024 | 2,034 |
June 2024 | 1,081 |
Email alerts
Citing articles via, affiliations.
- Online ISSN 2050-6201
- Copyright © 2024 International Society for Evolution, Medicine, and Public Health
- About Oxford Academic
- Publish journals with us
- University press partners
- What we publish
- New features
- Open access
- Institutional account management
- Rights and permissions
- Get help with access
- Accessibility
- Advertising
- Media enquiries
- Oxford University Press
- Oxford Languages
- University of Oxford
Oxford University Press is a department of the University of Oxford. It furthers the University's objective of excellence in research, scholarship, and education by publishing worldwide
- Copyright © 2024 Oxford University Press
- Cookie settings
- Cookie policy
- Privacy policy
- Legal notice
This Feature Is Available To Subscribers Only
Sign In or Create an Account
This PDF is available to Subscribers Only
For full access to this pdf, sign in to an existing account, or purchase an annual subscription.
Featured Topics
Featured series.
A series of random questions answered by Harvard experts.
Explore the Gazette
Read the latest.

Putting human past on the MAPS
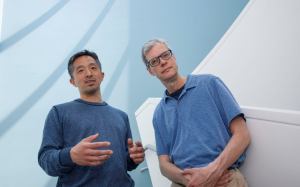
Does AI help humans make better decisions?
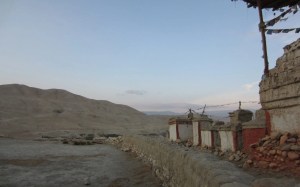
Tracking entwined histories of malaria, humans
Perspectives on gene editing.
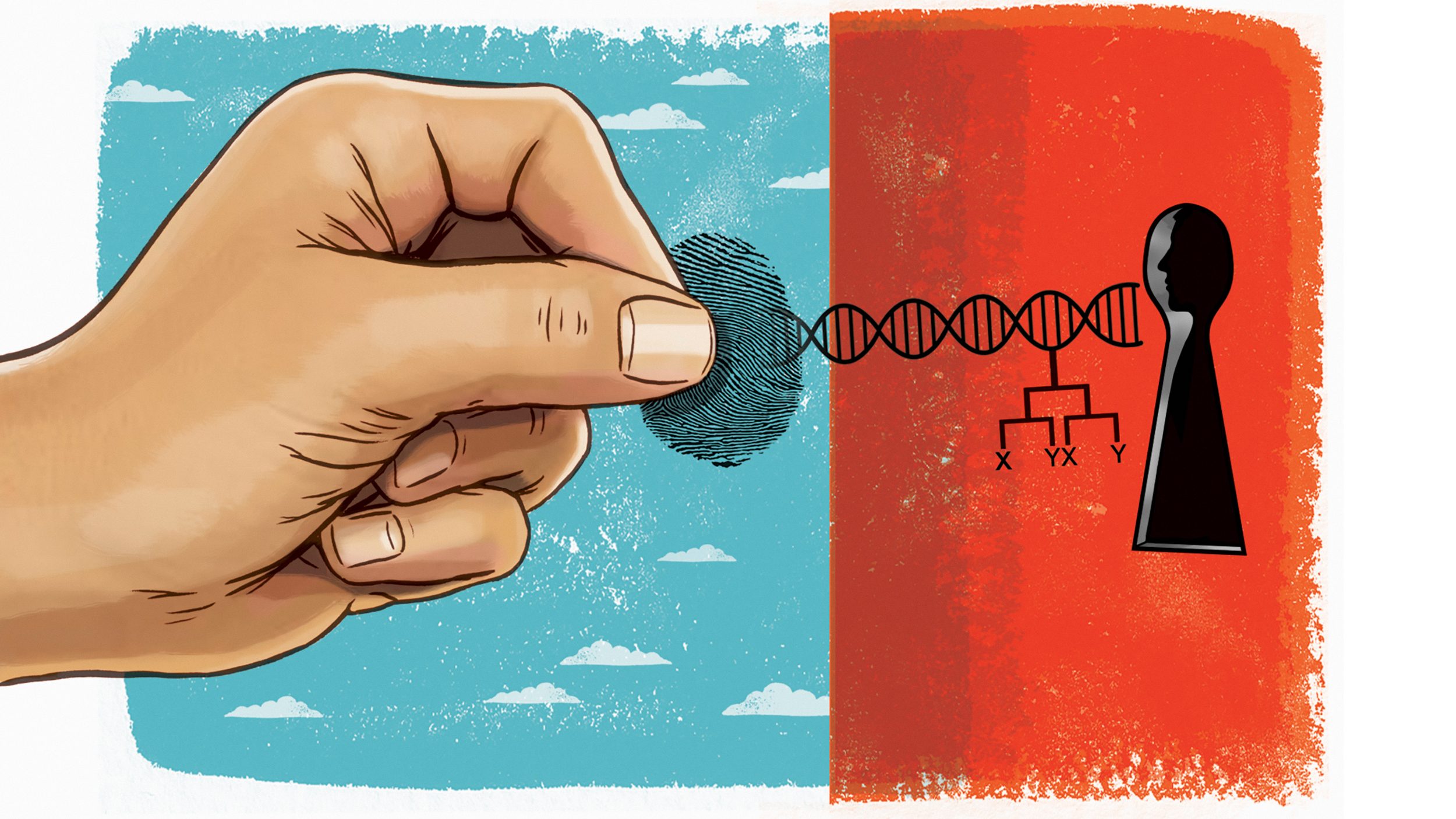
Illustration by Dan Mitchell
Mary Todd Bergman
Harvard Correspondent
Harvard researchers, others share their views on key issues in the field
Medicine is at a turning point, on the cusp of major change as disruptive technologies such as gene, RNA, and cell therapies enable scientists to approach diseases in new ways. The swiftness of this change is being driven by innovations such as CRISPR gene editing , which makes it possible to correct errors in DNA with relative ease.
Progress in this field has been so rapid that the dialogue around potential ethical, societal, and safety issues is scrambling to catch up.
This disconnect was brought into stark relief at the Second International Summit on Human Genome Editing , held in Hong Kong in November, when exciting updates about emerging therapies were eclipsed by a disturbing announcement. He Jiankui, a Chinese researcher, claimed that he had edited the genes of two human embryos, and that they had been brought to term.
There was immediate outcry from scientists across the world, and He was subjected to intense social pressure, including the removal of his affiliations, for having allegedly disregarded ethical norms and his patients’ safety.
Yet as I. Glenn Cohen, faculty director of the Petrie-Flom Center for Health Law Policy, Biotechnology, and Bioethics at Harvard Law School, has said, gene editing comes in many varieties, with many consequences. Any deep ethical discussion needs to take into account those distinctions.
Human genome editing: somatic vs. germline
The germline editing He claimed to have carried out is quite different from the somatic gene therapies that are currently changing the frontiers of medicine. While somatic gene editing affects only the patient being treated (and only some of his or her cells), germline editing affects all cells in an organism, including eggs and sperm, and so is passed on to future generations. The possible consequences of that are difficult to predict.
Somatic gene therapies involve modifying a patient’s DNA to treat or cure a disease caused by a genetic mutation. In one clinical trial, for example, scientists take blood stem cells from a patient, use CRISPR techniques to correct the genetic mutation causing them to produce defective blood cells, then infuse the “corrected” cells back into the patient, where they produce healthy hemoglobin. The treatment changes the patient’s blood cells, but not his or her sperm or eggs.
Germline human genome editing, on the other hand, alters the genome of a human embryo at its earliest stages. This may affect every cell, which means it has an impact not only on the person who may result, but possibly on his or her descendants. There are, therefore, substantial restrictions on its use.
Germline editing in a dish can help researchers figure out what the health benefits could be, and how to reduce risks. Those include targeting the wrong gene; off-target impacts, in which editing a gene might fix one problem but cause another; and mosaicism, in which only some copies of the gene are altered. For these and other reasons, the scientific community approaches germline editing with caution, and the U.S. and many other countries have substantial policy and regulatory restrictions on using germline human genome editing in people.
But many scientific leaders are asking: When the benefits are believed to outweigh the risks, and dangers can be avoided, should science consider moving forward with germline genome editing to improve human health? If the answer is yes, how can researchers do so responsibly?
CRISPR pioneer Feng Zhang of the Broad Institute of Harvard and MIT responded immediately to He’s November announcement by calling for a moratorium on implanting edited embryos in humans. Later, at a public event on “Altering the Human Genome” at the Belfer Center at Harvard Kennedy School (HKS), he explained why he felt it was important to wait:
“The moratorium is a pause. Society needs to figure out if we all want to do this, if this is good for society, and that takes time. If we do, we need to have guidelines first so that the people who do this work can proceed in a responsible way, with the right oversight and quality controls.”
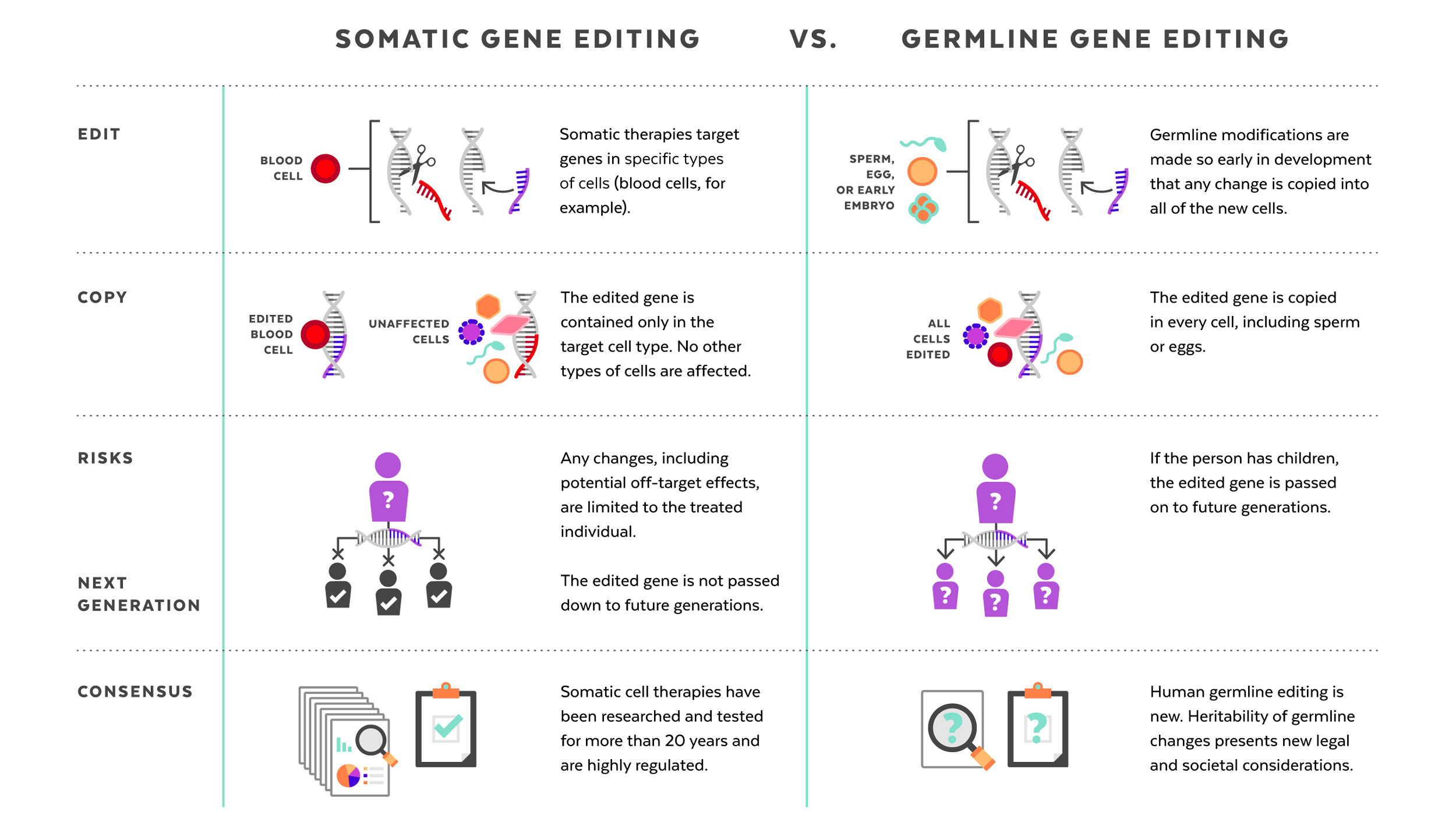
Professors at the University’s schools of medicine, law, business, and government saw He’s announcement as a turning point in the discussion about heritable gene therapies and shared their perspectives on the future of this technology with the Gazette.
Here are their thoughts, issue by issue:
Aside from the safety risks, human genome editing poses some hefty ethical questions. For families who have watched their children suffer from devastating genetic diseases, the technology offers the hope of editing cruel mutations out of the gene pool. For those living in poverty, it is yet another way for the privileged to vault ahead. One open question is where to draw the line between disease treatment and enhancement, and how to enforce it, considering differing attitudes toward conditions such as deafness.
Robert Truog , director of the Center for Bioethics at Harvard Medical School (HMS), provided context:
“This question is not as new as it seems. Evolution progresses by random mutations in the genome, which dwarf what can be done artificially with CRISPR. These random mutations often cause serious problems, and people are born with serious defects. In addition, we have been manipulating our environment in so many ways and exposing ourselves to a lot of chemicals that cause unknown changes to our genome. If we are concerned about making precise interventions to cure disease, we should also be interested in that.
“To me, the conversation around Dr. He is not about the fundamental merits of germline gene editing, which in the long run will almost certainly be highly beneficial. Instead, it’s about the oversight of science. The concern is that with technologies that are relatively easy to use, like CRISPR, how does the scientific community regulate itself? If there’s a silver lining to this cloud, I think it is that the scientific community did pull together to be critical of this work, and took the responsibility seriously to use the tools available to them to regulate themselves.”
When asked what the implications of He’s announcement are for the emerging field of precision medicine, Richard Hamermesh, faculty co-chair of the Harvard Business School/Kraft Precision Medicine Accelerator, said:
“Before we start working on embryos, we have a long way to go, and civilization has to think long and hard about it. There’s no question that gene editing technologies are potentially transformative and are the ultimate precision medicine. If you could precisely correct or delete genes that are causing problems — mutating or aberrant genes — that is the ultimate in precision. It would be so transformative for people with diseases caused by a single gene mutation, like sickle cell anemia and cystic fibrosis. Developing safe, effective ways to use gene editing to treat people with serious diseases with no known cures has so much potential to relieve suffering that it is hard to see how anyone could be against it.
“There is also commercial potential and that will drive it forward. A lot of companies are getting venture funding for interesting gene therapies, but they’re all going after tough medical conditions where there is an unmet need — [where] nothing is working — and they’re trying to find gene therapies to cure those diseases. Why should we stop trying to find cures?
“But anything where you’re going to be changing human embryos, it’s going to take a long time for us to figure out what is appropriate and what isn’t. That has to be done with great care in terms of ethics.”
George Q. Daley is dean of HMS, the Caroline Shields Walker Professor of Medicine, and a leader in stem cell science and cancer biology. As a spokesperson for the organizing committee of the Second International Summit on Human Genome Editing, he responded swiftly to He’s announcement in Hong Kong. Echoing those remarks, he said:
“It’s time to formulate what a clinical path to translation might look like so that we can talk about it. That does not mean that we’re ready to go into the clinic — we are not. We need to specify what the hurdles would be if one were to move forward responsibly and ethically. If you can’t surmount those hurdles, you don’t move forward.
“There are stark distinctions between editing genes in an embryo to prevent a baby from being born with sickle cell anemia and editing genes to alter the appearance or intelligence of future generations. There is a whole spectrum of considerations to be debated. The prospect includes an ultimate decision that we not go forward, that we decide that the benefits do not outweigh the costs.”
Asked how to prevent experiments like He’s while preserving academic freedom, Daley replied:
“For the past 15 years, I have been involved in efforts to establish international standards of professional conduct for stem cell research and its clinical translation, knowing full well that there could be — and has been — a growing number of independent practitioners directly marketing unproven interventions to vulnerable patients through the internet. We advocated so strongly for professional standards in an attempt to ward off the risks of an unregulated industry. Though imperfect, our efforts to encourage a common set of professional practices have been influential.
“You can’t control rogue scientists in any field. But with strongly defined guidelines for responsible professional conduct in place, such ethical violations like those of Dr. He should remain a backwater, because most practitioners will adhere to generally accepted norms. Scientists have a responsibility to come together to articulate professional standards and live by them. One has to raise the bar very high to define what the standards of safety and efficacy are, and what kind of oversight and independent judgment would be required for any approval.
“We have called for an ongoing international forum on human genome editing, and that could take many shapes. We’ve suggested that the national academies of more countries come together — the National Academy of Sciences in the U.S. and the Royal Society in the U.K. are very active here — because these are the groups most likely to have the expertise to convene these kinds of discussions and keep them going.”
Cohen , speaking to the legal consequences of germline human genome editing, said:
“I think we should slow down in our reaction to this case. It is not clear that the U.S. needs to react to Dr. He’s announcement with regulation. The FDA [Food and Drug Administration] already has a strong policy on germline gene editing in place. A rider in the Consolidated Appropriations Act of 2016 — since renewed — would have blocked the very same clinical application of human germline editing He announced, had it been attempted in the U.S.
“The scientific community has responded in the way I’d have liked it to. There is a difference between ‘governance’ and ‘self-governance.’ Where government uses law, the scientific community uses peer review, public censure, promotions, university affiliations, and funding to regulate themselves. In China, in Dr. He’s case, you have someone who’s (allegedly) broken national law and scientific conventions. That doesn’t mean you should halt research being done by everyone who’s law-abiding.
“Public policy or ethical discussion that’s divorced from how science is progressing is problematic. You need to bring everyone together to have robust discussions. I’m optimistic that this is happening, and has happened. It’s very hard to deal with a transnational problem with national legislation, but it would be great to reach international consensus on this subject. These efforts might not succeed, but ultimately they are worth pursuing.”
Professor Kevin Eggan of Harvard’s Department of Stem Cell and Regenerative Biology said, “The question we should focus on is: Will this be safe and help the health of a child? Can we demonstrate that we can fix a mutation that will cause a terrible health problem, accurately and without the risk of harming their potential child? If the answer is yes, then I believe germline human genome editing is likely to gain acceptance in time.
“There could be situations where it could help a couple, but the risks of something going wrong are real. But at this point, it would be impossible to make a risk-benefit calculation in a responsible manner for that couple. Before we could ever move toward the clinic, the scientific community must come to a consensus on how to measure success, and how to measure off-target effects in animal models.
“Even as recently as this past spring and fall, the results of animal studies using CRISPR — the same techniques Dr. He claimed to have used — generated a lot of confusion. There is disagreement about both the quality of the data and how to interpret it. Until we can come to agreement about what the results of animal experiments mean, how could we possibly move forward with people?
“As happened in England with mitochondrial replacement therapy, we should be able to come to both a scientific and a societal consensus of when and how this approach should be used. That’s missing.”
According to Catherine Racowsky, professor of obstetrics, gynecology and reproductive biology at Brigham and Women’s Hospital, constraints on the use of embryos in federally funded research pose barriers to studying the risks and benefits of germline editing in humans. She added:
“Until the work is done, carefully and with tight oversight, to understand any off-target effects of replacing or removing a particular gene, it is inappropriate to apply the technology in the clinical field. My understanding of Dr. He’s case is that there wasn’t a known condition in these embryos, and by editing the genes involved with HIV infection, he could also have increased the risks of susceptibility to influenza and West Nile viruses.
“We need a sound oversight framework, and it needs to be established globally. This is a technology that holds enormous promise, and it is likely to be applied to the embryo, but it should only be applied for clinical purposes after the right work has been done. That means we must have consensus on what applications are acceptable, that we have appropriate regulatory oversight, and, perhaps most importantly, that it is safe. The only way we’re going to be able to determine that these standards are met is to proceed cautiously, with reassessments of the societal and health benefits and the risks.”
Asked about public dialogue around germline human genome editing, George Church , Robert Winthrop Professor of Genetics at HMS, said:
“With in vitro fertilization (IVF), ‘test tube babies’ was an intentionally scary term. But after Louise Brown, the first IVF baby, was born healthy 40 years ago, attitudes changed radically. Ethics flipped 180 degrees, from it being a horrifying idea to being unacceptable to prevent parents from having children by this new method. If these edited twins are proven healthy, very different discussions will arise. For example, is a rate of 900,000 deaths from HIV infection per year a greater risk than West Nile virus, or influenza? How effective is each vaccine?”
Science, technology, and society
Sheila Jasanoff , founding director of the Science, Technology, and Society program at HKS, has been calling for a “global observatory” on gene editing, an international network of scholars and organizations dedicated to promoting exchange across disciplinary and cultural divides. She said:
“The notion that the only thing we should care about is the risk to individuals is very American. So far, the debate has been fixated on potential physical harm to individuals, and not anything else. This is not a formulation shared with other countries in the world, including practically all of Europe. Considerations of risk have equally to do with societal risk. That includes the notion of the family, and what it means to have a ‘designer baby.’
“These were not diseased babies Dr. He was trying to cure. The motivation for the intervention was that they live in a country with a high stigma attached to HIV/AIDS, and the father had it and agreed to the intervention because he wanted to keep his children from contracting AIDS. AIDS shaming is a fact of life in China, and now it won’t be applied to these children. So, are we going to decide that it’s OK to edit as-yet-to-be children to cater to this particular idea of a society?
“It’s been said that ‘the genie is out of the bottle’ with germline human genome editing. I just don’t think that’s true. After all, we have succeeded in keeping ‘nuclear’ inside the bottle. Humanity doesn’t lack the will, intelligence, or creativity to come up with ways for using technology for good and not ill.
“We don’t require students to learn the moral dimensions of science and technology, and that has to change. I think we face similar challenges in robotics, artificial intelligence, and all kinds of frontier fields that have the potential to change not just individuals but the entirety of what it means to be a human being.
“Science has this huge advantage over most professional thought in that it has a universal language. Scientists can hop from lab to lab internationally in a way that lawyers cannot because laws are written in many languages and don’t translate easily. It takes a very long time for people to understand each other across these boundaries. A foundational concept for human dignity? It would not be the same thing between cultures.
“I would like to see a ‘global observatory’ that goes beyond gene editing and addresses emerging technologies more broadly.”
To learn more:
Technology and Public Purpose project, Belfer Center for Science and International Affairs, Harvard Kennedy School of Government, https://www.belfercenter.org/tapp/person
Concluding statement from the Second International Summit on Human Genome Editing. http://www8.nationalacademies.org/onpinews/newsitem.aspx?RecordID=11282018b
A global observatory for gene editing: Sheila Jasanoff and J. Benjamin Hurlbut call for an international network of scholars and organizations to support a new kind of conversation. https://www.nature.com/articles/d41586-018-03270-w
Building Capacity for a Global Genome Editing Observatory: Institutional Design. http://europepmc.org/abstract/MED/29891181
Glenn Cohen’s blog: How Scott Gottlieb is Wrong on the Gene Edited Baby Debacle. http://blog.petrieflom.law.harvard.edu/2018/11/29/how-scott-gottlieb-is-wrong-on-the-gene-edited-baby-debacle/
Gene-Editing: Interpretation of Current Law and Legal Policy. https://www.ncbi.nlm.nih.gov/pmc/articles/PMC5651701/
Forum: Harvard T.H. Chan School of Public Health event on the promises and challenges of gene editing, May 2017: https://theforum.sph.harvard.edu/events/gene-editing/
Petrie-Flom Center Annual Conference: Consuming Genetics: Ethical and Legal Considerations of New Technologies: http://petrieflom.law.harvard.edu/events/details/2019-petrie-flom-center-annual-conference
Share this article
You might like.

Harvard digital atlas plots patterns from history ancient and modern

One judge’s track record — with and without algorithm — surprises researchers
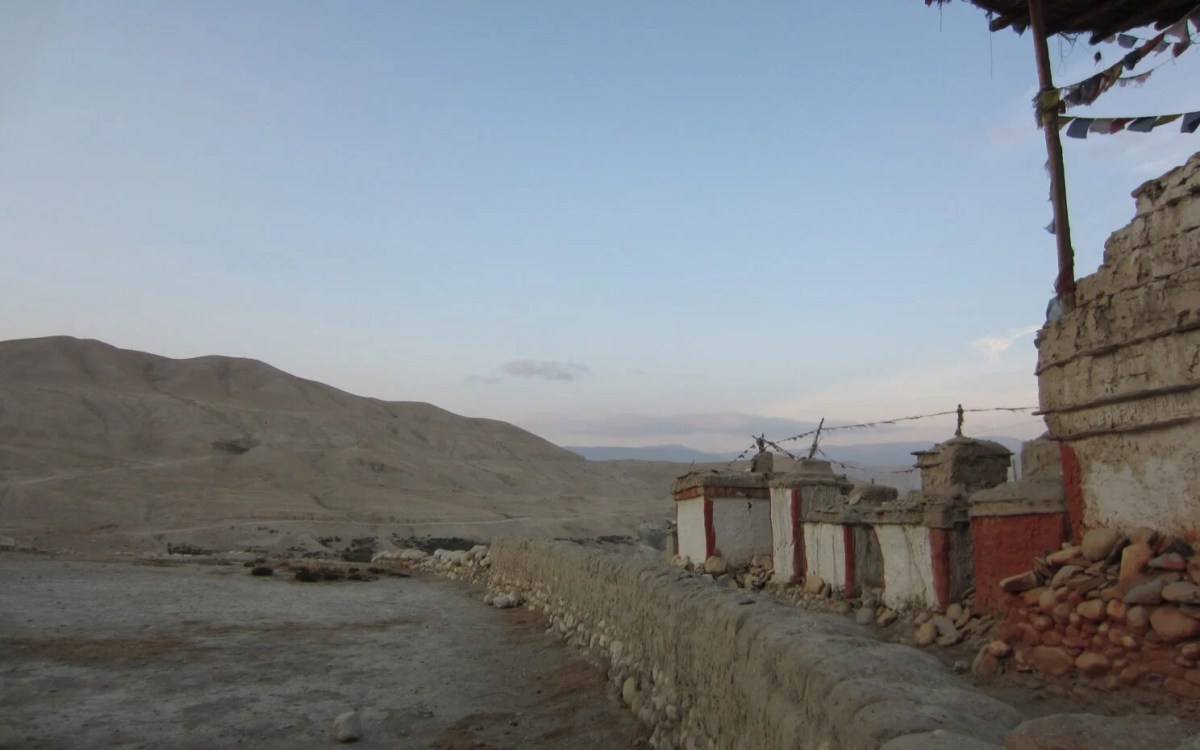
New study of ancient genomes tracks disease over 5,500 years, factors in spread, including trade, warfare, colonialism, and slavery
When should Harvard speak out?
Institutional Voice Working Group provides a roadmap in new report
Had a bad experience meditating? You're not alone.
Altered states of consciousness through yoga, mindfulness more common than thought and mostly beneficial, study finds — though clinicians ill-equipped to help those who struggle
College sees strong yield for students accepted to Class of 2028
Financial aid was a critical factor, dean says

Home > ETD > DISSERTATIONS > AAI9638129
Genetic engineering of rice: Agrobacterium tumefaciens-mediated transformation of rice and evaluation of a corn pollen-specific promoter using thegusA gene in transgenic rice
Rhodora Romero Aldemita , Purdue University
Rice genetic engineering permits access to an unlimited gene pool through the transfer of desirable genes between any two species of interest, irrespective of their evolutionary or taxonomic relationships. However, there are major problems to be considered for a successful foreign gene introgression into rice. Two of the major problems were considered in this thesis: first is the development of an efficient transformation method for foreign gene transfer to rice, and the second is the identification of conditions that will allow expression of the transgene in the proper developmental and tissue-specific manner. Agrobacterium-mediated transformation of rice was studied following reports of its successes in transformation of dicots and its potential in transforming monocots. Conditions for successful Agrobacterium-mediated transformation of rice was studied based on transient GUS expression, including the type and age of explant, preinduction and co-cultivation conditions, acetosyringone concentrations, the media (co-cultivation and selection), the promoter and the Agrobacterium strains. A protocol was etablished which resulted in the production of stably transformed calli at high efficiency (36.1% for Nortai and 5.9% for TCS10) using immature embryos treated with At656 (pCNL56). Transgenic plants were not obtained which may be due to the long duration of exposure of inoculated embryos and calli to high concentrations of G418 during selection. Following reports of Hiei et al. (1994) in obtaining high efficiency of transformed rice plants using scutellar tissue infection with LBA4404 (pTOK233), immature embryos of indica (IR54, IR72, and TCS10) and a japonica variety (Radon) were infected with the same Agrobacterium strain, using the protocol established in this study with minor modifications based on Hiei et al. (1994). Transgenic plants were obtained after hygromycin selection at a high efficiency for Radon (27.0%), and at a lower but acceptable efficiency for TCS10 (0.5% to 1.2%) and IR72 (1.9% to 8.3%). Southern blot analysis of genomic DNA from transgenic Radon, TCS10, and IR72 plants showed very few integration events. Fertility of the T$\rm\sb{o}$ plants was comparable to the seed-derived plants. Progeny analyses of seeds from T$\rm\sb{o}$ plants exhibited a 3:1 ratio of GUS$\sp+$ to GUS$\sp-$ plants, indicative of gene integration at a single locus. The second major problem addressed was identification of conditions that would allow the expression of a transgene in the proper developmental and tissue-specific manner. This problem was dealt with using the promoter of a corn pollen-specific gene Zmg13 in order to develop a system to genetically engineer rice whose fertility and sterility can be controlled. Rice protoplasts were co-transformed with the plasmid pCPPGUS containing the Zmg13 promoter starting from the $-$260 position in front of the $\beta$-glucuronidase gene, and a bar gene-containing plasmid (p35SBarB) using polyethylene glycol-mediated transformation. Transgenic plants were obtained after selection with phosphinothricin, which produced high selection and co-transformation efficiencies. Transgenic plants exhibited GUS activity in a pollen-specific manner depending on the transgene copy number and the physiological state of the anther/pollen.
Hodges, Purdue University.
Subject Area
Botany|Plant propagation|Genetics
Off-Campus Purdue Users: To access this dissertation, please log in to our proxy server .
Since October 23, 2006
Advanced Search
- Notify me via email or RSS
- Purdue Libraries
- Purdue University Press Open Access Collections
Links for Authors
- Policies and Help Documentation
- Collections
- Disciplines
Home | About | FAQ | My Account | Accessibility Statement
Privacy Copyright

Research Topics & Ideas
Biotechnology and Genetic Engineering
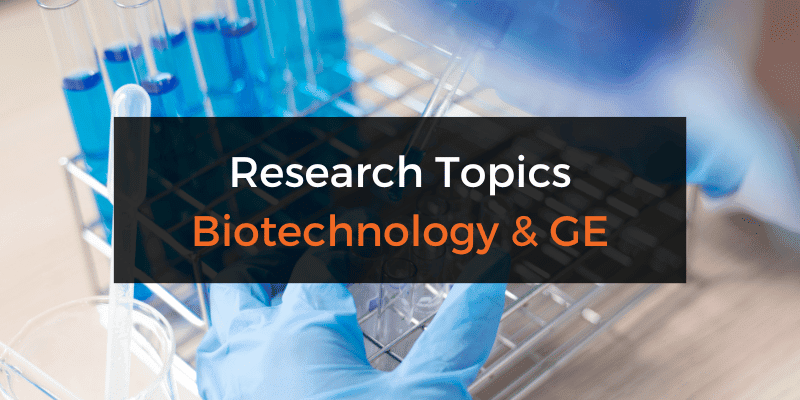
If you’re just starting out exploring biotechnology-related topics for your dissertation, thesis or research project, you’ve come to the right place. In this post, we’ll help kickstart your research topic ideation process by providing a hearty list of research topics and ideas , including examples from recent studies.
PS – This is just the start…
We know it’s exciting to run through a list of research topics, but please keep in mind that this list is just a starting point . To develop a suitable research topic, you’ll need to identify a clear and convincing research gap , and a viable plan to fill that gap.
If this sounds foreign to you, check out our free research topic webinar that explores how to find and refine a high-quality research topic, from scratch. Alternatively, if you’d like hands-on help, consider our 1-on-1 coaching service .
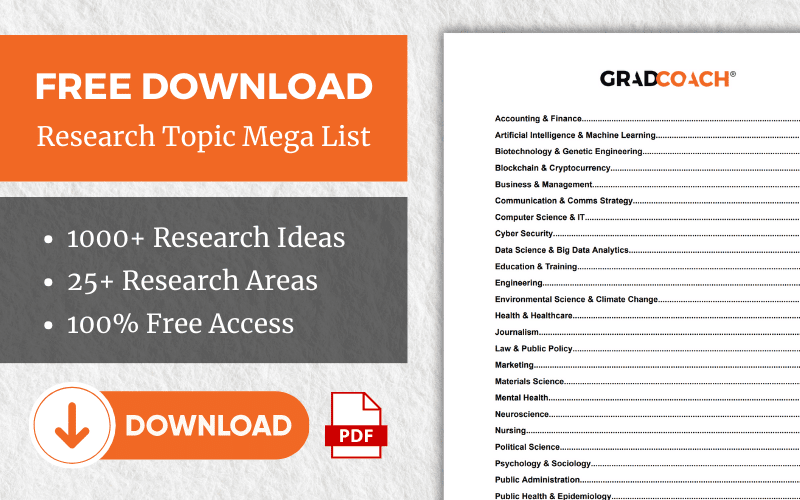
Biotechnology Research Topic Ideas
Below you’ll find a list of biotech and genetic engineering-related research topics ideas. These are intentionally broad and generic , so keep in mind that you will need to refine them a little. Nevertheless, they should inspire some ideas for your project.
- Developing CRISPR-Cas9 gene editing techniques for treating inherited blood disorders.
- The use of biotechnology in developing drought-resistant crop varieties.
- The role of genetic engineering in enhancing biofuel production efficiency.
- Investigating the potential of stem cell therapy in regenerative medicine for spinal cord injuries.
- Developing gene therapy approaches for the treatment of rare genetic diseases.
- The application of biotechnology in creating biodegradable plastics from plant materials.
- The use of gene editing to enhance nutritional content in staple crops.
- Investigating the potential of microbiome engineering in treating gastrointestinal diseases.
- The role of genetic engineering in vaccine development, with a focus on mRNA vaccines.
- Biotechnological approaches to combat antibiotic-resistant bacteria.
- Developing genetically engineered organisms for bioremediation of polluted environments.
- The use of gene editing to create hypoallergenic food products.
- Investigating the role of epigenetics in cancer development and therapy.
- The application of biotechnology in developing rapid diagnostic tools for infectious diseases.
- Genetic engineering for the production of synthetic spider silk for industrial use.
- Biotechnological strategies for improving animal health and productivity in agriculture.
- The use of gene editing in creating organ donor animals compatible with human transplantation.
- Developing algae-based bioreactors for carbon capture and biofuel production.
- The role of biotechnology in enhancing the shelf life and quality of fresh produce.
- Investigating the ethics and social implications of human gene editing technologies.
- The use of CRISPR technology in creating models for neurodegenerative diseases.
- Biotechnological approaches for the production of high-value pharmaceutical compounds.
- The application of genetic engineering in developing pest-resistant crops.
- Investigating the potential of gene therapy in treating autoimmune diseases.
- Developing biotechnological methods for producing environmentally friendly dyes.
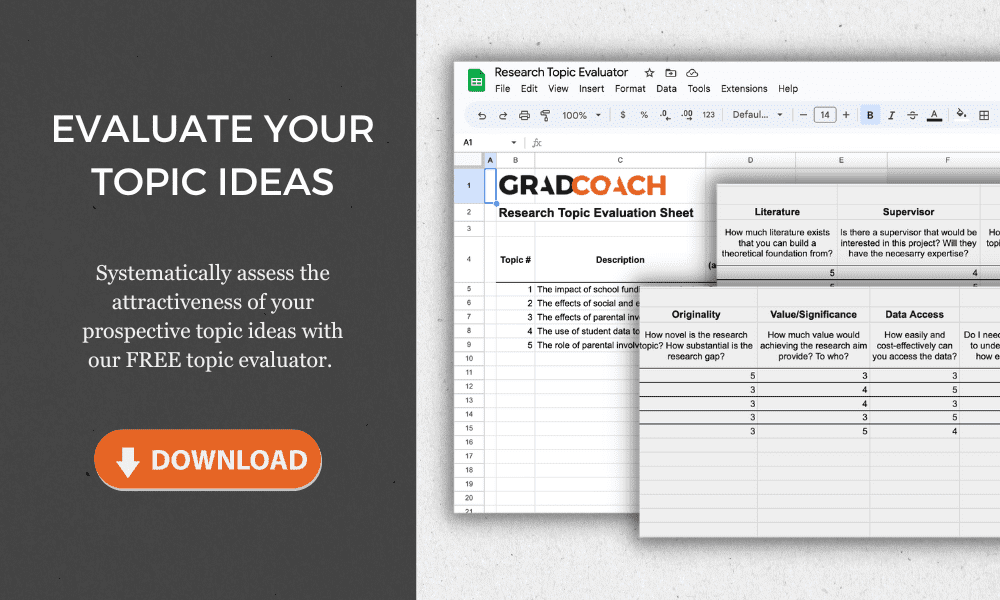
Biotech & GE Research Topic Ideas (Continued)
- The use of genetic engineering in enhancing the efficiency of photosynthesis in plants.
- Biotechnological innovations in creating sustainable aquaculture practices.
- The role of biotechnology in developing non-invasive prenatal genetic testing methods.
- Genetic engineering for the development of novel enzymes for industrial applications.
- Investigating the potential of xenotransplantation in addressing organ donor shortages.
- The use of biotechnology in creating personalised cancer vaccines.
- Developing gene editing tools for combating invasive species in ecosystems.
- Biotechnological strategies for improving the nutritional quality of plant-based proteins.
- The application of genetic engineering in enhancing the production of renewable energy sources.
- Investigating the role of biotechnology in creating advanced wound care materials.
- The use of CRISPR for targeted gene activation in regenerative medicine.
- Biotechnological approaches to enhancing the sensory qualities of plant-based meat alternatives.
- Genetic engineering for improving the efficiency of water use in agriculture.
- The role of biotechnology in developing treatments for rare metabolic disorders.
- Investigating the use of gene therapy in age-related macular degeneration.
- The application of genetic engineering in developing allergen-free nuts.
- Biotechnological innovations in the production of sustainable and eco-friendly textiles.
- The use of gene editing in studying and treating sleep disorders.
- Developing biotechnological solutions for the management of plastic waste.
- The role of genetic engineering in enhancing the production of essential vitamins in crops.
- Biotechnological approaches to the treatment of chronic pain conditions.
- The use of gene therapy in treating muscular dystrophy.
- Investigating the potential of biotechnology in reversing environmental degradation.
- The application of genetic engineering in improving the shelf life of vaccines.
- Biotechnological strategies for enhancing the efficiency of mineral extraction in mining.
Recent Biotech & GE-Related Studies
While the ideas we’ve presented above are a decent starting point for finding a research topic in biotech, they are fairly generic and non-specific. So, it helps to look at actual studies in the biotech space to see how this all comes together in practice.
Below, we’ve included a selection of recent studies to help refine your thinking. These are actual studies, so they can provide some useful insight as to what a research topic looks like in practice.
- Genetic modifications associated with sustainability aspects for sustainable developments (Sharma et al., 2022)
- Review On: Impact of Genetic Engineering in Biotic Stresses Resistance Crop Breeding (Abebe & Tafa, 2022)
- Biorisk assessment of genetic engineering — lessons learned from teaching interdisciplinary courses on responsible conduct in the life sciences (Himmel et al., 2022)
- Genetic Engineering Technologies for Improving Crop Yield and Quality (Ye et al., 2022)
- Legal Aspects of Genetically Modified Food Product Safety for Health in Indonesia (Khamdi, 2022)
- Innovative Teaching Practice and Exploration of Genetic Engineering Experiment (Jebur, 2022)
- Efficient Bacterial Genome Engineering throughout the Central Dogma Using the Dual-Selection Marker tetAOPT (Bayer et al., 2022)
- Gene engineering: its positive and negative effects (Makrushina & Klitsenko, 2022)
- Advances of genetic engineering in streptococci and enterococci (Kurushima & Tomita, 2022)
- Genetic Engineering of Immune Evasive Stem Cell-Derived Islets (Sackett et al., 2022)
- Establishment of High-Efficiency Screening System for Gene Deletion in Fusarium venenatum TB01 (Tong et al., 2022)
- Prospects of chloroplast metabolic engineering for developing nutrient-dense food crops (Tanwar et al., 2022)
- Genetic research: legal and ethical aspects (Rustambekov et al., 2023). Non-transgenic Gene Modulation via Spray Delivery of Nucleic Acid/Peptide Complexes into Plant Nuclei and Chloroplasts (Thagun et al., 2022)
- The role of genetic breeding in food security: A review (Sam et al., 2022). Biotechnology: use of available carbon sources on the planet to generate alternatives energy (Junior et al., 2022)
- Biotechnology and biodiversity for the sustainable development of our society (Jaime, 2023) Role Of Biotechnology in Agriculture (Shringarpure, 2022)
- Plants That Can be Used as Plant-Based Edible Vaccines; Current Situation and Recent Developments (İsmail, 2022)
As you can see, these research topics are a lot more focused than the generic topic ideas we presented earlier. So, in order for you to develop a high-quality research topic, you’ll need to get specific and laser-focused on a specific context with specific variables of interest. In the video below, we explore some other important things you’ll need to consider when crafting your research topic.
Get 1-On-1 Help
If you’re still unsure about how to find a quality research topic, check out our Research Topic Kickstarter service, which is the perfect starting point for developing a unique, well-justified research topic.
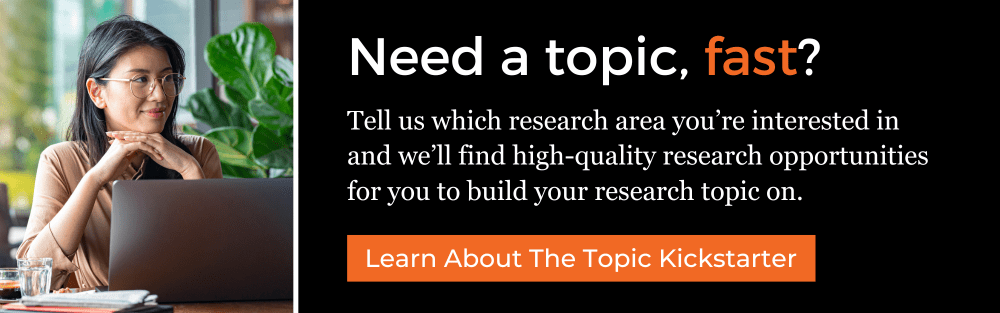
You Might Also Like:
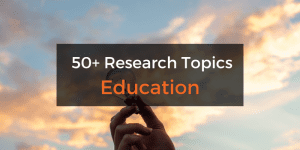
Submit a Comment Cancel reply
Your email address will not be published. Required fields are marked *
Save my name, email, and website in this browser for the next time I comment.
- Print Friendly

An official website of the United States government
The .gov means it’s official. Federal government websites often end in .gov or .mil. Before sharing sensitive information, make sure you’re on a federal government site.
The site is secure. The https:// ensures that you are connecting to the official website and that any information you provide is encrypted and transmitted securely.
- Publications
- Account settings
Preview improvements coming to the PMC website in October 2024. Learn More or Try it out now .
- Advanced Search
- Journal List
- HHS Author Manuscripts


Development and Applications of CRISPR-Cas9 for Genome Engineering
Patrick d. hsu.
1 Broad Institute of MIT and Harvard, 7 Cambridge Center, Cambridge, MA 02141, USA
2 McGovern Institute for Brain Research, Department of Brain and Cognitive Sciences, Department of Biological Engineering, Massachusetts Institute of Technology, Cambridge, MA 02139, USA
3 Department of Molecular and Cellular Biology, Harvard University, Cambridge, MA 02138, USA
Eric S. Lander
Associated data.
Recent advances in genome engineering technologies based on the CRISPR-associated RNA-guided endonuclease Cas9 are enabling the systematic interrogation of mammalian genome function. Analogous to the search function in modern word processors, Cas9 can be guided to specific locations within complex genomes by a short RNA search string. Using this system, DNA sequences within the endogenous genome and their functional outputs are now easily edited or modulated in virtually any organism of choice. Cas9-mediated genetic perturbation is simple and scalable, empowering researchers to elucidate the functional organization of the genome at the systems level and establish causal linkages between genetic variations and biological phenotypes. In this Review, we describe the development and applications of Cas9 for a variety of research or translational applications while highlighting challenges as well as future directions. Derived from a remarkable microbial defense system, Cas9 is driving innovative applications from basic biology to biotechnology and medicine.
Introduction
The development of recombinant DNA technology in the 1970s marked the beginning of a new era for biology. For the first time, molecular biologists gained the ability to manipulate DNA molecules, making it possible to study genes and harness them to develop novel medicine and biotechnology. Recent advances in genome engineering technologies are sparking a new revolution in biological research. Rather than studying DNA taken out of the context of the genome, researchers can now directly edit or modulate the function of DNA sequences in their endogenous context in virtually any organism of choice, enabling them to elucidate the functional organization of the genome at the systems level, as well as identify causal genetic variations.
Broadly speaking, genome engineering refers to the process of making targeted modifications to the genome, its contexts (e.g., epigenetic marks), or its outputs (e.g., transcripts). The ability to do so easily and efficiently in eukaryotic and especially mammalian cells holds immense promise to transform basic science, biotechnology, and medicine ( Figure 1 ).

Genetic and epigenetic control of cells with genome engineering technologies is enabling a broad range of applications from basic biology to biotechnology and medicine. (Clockwise from top) Causal genetic mutations or epigenetic variants associated with altered biological function or disease phenotypes can now be rapidly and efficiently recapitulated in animal or cellular models (Animal models, Genetic variation). Manipulating biological circuits could also facilitate the generation of useful synthetic materials, such as algae-derived, silicabased diatoms for oral drug delivery (Materials). Additionally, precise genetic engineering of important agricultural crops could confer resistance to environmental deprivation or pathogenic infection, improving food security while avoiding the introduction of foreign DNA (Food). Sustainable and cost-effective biofuels are attractive sources for renewable energy, which could be achieved by creating efficient metabolic pathways for ethanol production in algae or corn (Fuel). Direct in vivo correction of genetic or epigenetic defects in somatic tissue would be permanent genetic solutions that address the root cause of genetically encoded disorders (Gene surgery). Finally, engineering cells to optimize high yield generation of drug precursors in bacterial factories could significantly reduce the cost and accessibility of useful therapeutics (Drug development).
For life sciences research, technologies that can delete, insert, and modify the DNA sequences of cells or organisms enable dissecting the function of specific genes and regulatory elements. Multiplexed editing could further allow the interrogation of gene or protein networks at a larger scale. Similarly, manipulating transcriptional regulation or chromatin states at particular loci can reveal how genetic material is organized and utilized within a cell, illuminating relationships between the architecture of the genome and its functions. In biotechnology, precise manipulation of genetic building blocks and regulatory machinery also facilitates the reverse engineering or reconstruction of useful biological systems, for example, by enhancing biofuel production pathways in industrially relevant organisms or by creating infection-resistant crops. Additionally, genome engineering is stimulating a new generation of drug development processes and medical therapeutics. Perturbation of multiple genes simultaneously could model the additive effects that underlie complex polygenic disorders, leading to new drug targets, while genome editing could directly correct harmful mutations in the context of human gene therapy ( Tebas et al., 2014 ).
Eukaryotic genomes contain billions of DNA bases and are difficult to manipulate. One of the breakthroughs in genome manipulation has been the development of gene targeting by homologous recombination (HR), which integrates exogenous repair templates that contain sequence homology to the donor site ( Figure 2A ) ( Capecchi, 1989 ). HR-mediated targeting has facilitated the generation of knockin and knockout animal models via manipulation of germline competent stem cells, dramatically advancing many areas of biological research. However, although HR-mediated gene targeting produces highly precise alterations, the desired recombination events occur extremely infrequently (1 in 10 6 –10 9 cells) ( Capecchi, 1989 ), presenting enormous challenges for large-scale applications of gene-targeting experiments.

(A) DNA double-strand breaks (DSBs) are typically repaired by nonhomologous end-joining (NHEJ) or homology-directed repair (HDR). In the error-prone NHEJ pathway, Ku heterodimers bind to DSB ends and serve as a molecular scaffold for associated repair proteins. Indels are introduced when the complementary strands undergo end resection and misaligned repair due to micro-homology, eventually leading to frameshift mutations and gene knockout. Alternatively, Rad51 proteins may bind DSB ends during the initial phase of HDR, recruiting accessory factors that direct genomic recombination with homology arms on an exogenous repair template. Bypassing the matching sister chromatid facilitates the introduction of precise gene modifications.
(B) Zinc finger (ZF) proteins and transcription activator-like effectors (TALEs) are naturally occurring DNA-binding domains that can be modularly assembled to target specific sequences. ZF and TALE domains each recognize 3 and 1 bp of DNA, respectively. Such DNA-binding proteins can be fused to the FokI endonuclease to generate programmable site-specific nucleases.
(C) The Cas9 nuclease from the microbial CRISPR adaptive immune system is localized to specific DNA sequences via the guide sequence on its guide RNA (red), directly base-pairing with the DNA target. Binding of a protospacer-adjacent motif (PAM, blue) downstream of the target locus helps to direct Cas9-mediated DSBs.
To overcome these challenges, a series of programmable nuclease-based genome editing technologies have been developed in recent years, enabling targeted and efficient modification of a variety of eukaryotic and particularly mammalian species. Of the current generation of genome editing technologies, the most rapidly developing is the class of RNA-guided endonucleases known as Cas9 from the microbial adaptive immune system CRISPR (clustered regularly interspaced short palindromic repeats), which can be easily targeted to virtually any genomic location of choice by a short RNA guide. Here, we review the development and applications of the CRISPR-associated endonuclease Cas9 as a platform technology for achieving targeted perturbation of endogenous genomic elements and also discuss challenges and future avenues for innovation.
Programmable Nucleases as Tools for Efficient and Precise Genome Editing
A series of studies by Haber and Jasin ( Rudin et al., 1989 ; Plessis et al., 1992 ; Rouet et al., 1994 ; Choulika et al., 1995 ; Bibikova et al., 2001 ; Bibikova et al., 2003 ) led to the realization that targeted DNA double-strand breaks (DSBs) could greatly stimulate genome editing through HR-mediated recombination events. Subsequently, Carroll and Chandrasegaran demonstrated the potential of designer nucleases based on zinc finger proteins for efficient, locus-specific HR ( Bibikova et al., 2001 , 2003 ). Moreover, it was shown in the absence of an exogenous homology repair template that localized DSBs can induce insertions or deletion mutations (indels) via the error-prone nonhomologous end-joining (NHEJ) repair pathway ( Figure 2A ) ( Bibikova et al., 2002 ). These early genome editing studies established DSB-induced HR and NHEJ as powerful pathways for the versatile and precise modification of eukaryotic genomes.
To achieve effective genome editing via introduction of site-specific DNA DSBs, four major classes of customizable DNA-binding proteins have been engineered so far: meganucleases derived from microbial mobile genetic elements ( Smith et al., 2006 ), zinc finger (ZF) nucleases based on eukaryotic transcription factors ( Urnov et al., 2005 ; Miller et al., 2007 ), transcription activator-like effectors (TALEs) from Xanthomonas bacteria ( Christian et al., 2010 ; Miller et al., 2011 ; Boch et al., 2009 ; Moscou and Bogdanove, 2009 ), and most recently the RNA-guided DNA endonuclease Cas9 from the type II bacterial adaptive immune system CRISPR ( Cong et al., 2013 ; Mali et al., 2013a ).
Meganuclease, ZF, and TALE proteins all recognize specific DNA sequences through protein-DNA interactions. Although meganucleases integrate its nuclease and DNA-binding domains, ZF and TALE proteins consist of individual modules targeting 3 or 1 nucleotides (nt) of DNA, respectively ( Figure 2B ). ZFs and TALEs can be assembled in desired combinations and attached to the nuclease domain of FokI to direct nucleolytic activity toward specific genomic loci. Each of these platforms, however, has unique limitations.
Meganucleases have not been widely adopted as a genome engineering platform due to lack of clear correspondence between meganuclease protein residues and their target DNA sequence specificity. ZF domains, on the other hand, exhibit context-dependent binding preference dueto crosstalk between adjacent modules when assembled into a larger array ( Maeder et al., 2008 ). Although multiple strategies have been developed to account for these limitations ( Gonzaelz et al., 2010 ; Sander et al., 2011 ), assembly of functional ZFPs with the desired DNA binding specificity remains a major challenge that requires an extensive screening process. Similarly, although TALE DNA-binding monomers are for the most part modular, they can still suffer from context-dependent specificity ( Juillerat et al., 2014 ), and their repetitive sequences render construction of novel TALE arrays labor intensive and costly.
Given the challenges associated with engineering of modular DNA-binding proteins, new modes of recognition would significantly simplify the development of custom nucleases. The CRISPR nuclease Cas9 is targeted by a short guide RNA that recognizes the target DNA via Watson-Crick base pairing ( Figure 2C ). The guide sequence within these CRISPR RNAs typically corresponds to phage sequences, constituting the natural mechanism for CRISPR antiviral defense, but can be easily replaced by a sequence of interest to retarget the Cas9 nuclease. Multiplexed targeting by Cas9 can now be achieved at unprecedented scale by introducing a battery of short guide RNAs rather than a library of large, bulky proteins. The ease of Cas9 targeting, its high efficiency as a site-specific nuclease, and the possibility for highly multiplexed modifications have opened up a broad range of biological applications across basic research to biotechnology and medicine.
The utility of customizable DNA-binding domains extends far beyond genome editing with site-specific endonucleases. Fusing them to modular, sequence-agnostic functional effector domains allows flexible recruitment of desired perturbations, such as transcriptional activation, to a locus of interest ( Xu and Bestor, 1997 ; Beerli et al., 2000a ; Konermann et al., 2013 ; Maeder et al., 2013a ; Mendenhall et al., 2013 ). In fact, any modular enzymatic component can, in principle, be substituted, allowing facile additions to the genome engineering toolbox. Integration of genome- and epigenome-modifying enzymes with inducible protein regulation further allows precise temporal control of dynamic processes ( Beerli et al., 2000b ; Konermann et al., 2013 ).
CRISPR-Cas9: From Yogurt to Genome Editing
The recent development of the Cas9 endonuclease for genome editing draws upon more than a decade of basic research into understanding the biological function of the mysterious repetitive elements now known as CRISPR ( Figure 3 ), which are found throughout the bacterial and archaeal diversity. CRISPR loci typically consist of a clustered set of CRISPR-associated (Cas) genes and the signature CRISPR array—a series of repeat sequences (direct repeats) interspaced by variable sequences (spacers) corresponding to sequences within foreign genetic elements (protospacers) ( Figure 4 ). Whereas Cas genes are translated into proteins, most CRISPR arrays are first transcribed as a single RNA before subsequent processing into shorter CRISPR RNAs (crRNAs), which direct the nucleolytic activity of certain Cas enzymes to degrade target nucleic acids.

Cas9 has also been referred to as Cas5, Csx12, and Csn1 in literature prior to 2012. For clarity, we exclusively adopt the Cas9 nomenclature throughout this Review. CRISPR, clustered regularly interspaced short palindromic repeats; Cas, CRISPR-associated; crRNA, CRISPR RNA; DSB, double-strand break; tracrRNA, trans -activating CRISPR RNA.

Following invasion of the cell by foreign genetic elements from bacteriophages or plasmids (step 1: phage infection), certain CRISPR-associated (Cas) enzymes acquire spacers from the exogenous protospacer sequences and install them into the CRISPR locus within the prokaryotic genome (step 2: spacer acquisition). These spacers are segregated between direct repeats that allow the CRISPR system to mediate self and nonself recognition. The CRISPR array is a noncoding RNA transcript that is enzymatically maturated through distinct pathways that are unique to each type of CRISPR system (step 3: crRNA biogenesis and processing).
In types I and III CRISPR, the pre-crRNA transcript is cleaved within the repeats by CRISPR-associated ribonucleases, releasing multiple small crRNAs. Type III crRNA intermediates are further processed at the 3′ end by yet-to-be-identified RNases to produce the fully mature transcript. In type II CRISPR, an associated trans -activating CRISPR RNA (tracrRNA) hybridizes with the direct repeats, forming an RNA duplex that is cleaved and processed by endogenous RNase III and other unknown nucleases. Maturated crRNAs from type I and III CRISPR systems are then loaded onto effector protein complexes for target recognition and degradation. In type II systems, crRNA-tracrRNA hybrids complex with Cas9 to mediate interference.
Both type I and III CRISPR systems use multiprotein interference modules to facilitate target recognition. In type I CRISPR, the Cascade complex is loaded with a crRNA molecule, constituting a catalytically inert surveillance complex that recognizes target DNA. The Cas3 nuclease is then recruited to the Cascade-bound R loop, mediating target degradation. In type III CRISPR, crRNAs associate either with Csm or Cmr complexes that bind and cleave DNA and RNA substrates, respectively. In contrast, the type II system requires only the Cas9 nuclease to degrade DNA matching its dual guide RNA consisting of a crRNA-tracrRNA hybrid.
The CRISPR story began in 1987. While studying the iap enzyme involved in isozyme conversion of alkaline phosphatase in E. coli , Nakata and colleagues reported a curious set of 29 nt repeats downstream of the iap gene ( Ishino et al., 1987 ). Unlike most repetitive elements, which typically take the form of tandem repeats like TALE repeat monomers, these 29 nt repeats were interspaced by five intervening 32 nt nonrepetitive sequences. Over the next 10 years, as more microbial genomes were sequenced, additional repeat elements were reported from genomes of different bacterial and archaeal strains. Mojica and colleagues eventually classified interspaced repeat sequences as a unique family of clustered repeat elements present in >40% of sequenced bacteria and 90% of archaea ( Mojica et al., 2000 ).
These early findings began to stimulate interest in such microbial repeat elements. By 2002, Jansen and Mojica coined the acronym CRISPR to unify the description of microbial genomic loci consisting of an interspaced repeat array ( Jansen et al., 2002 ; Barrangou and van der Oost, 2013 ). At the same time, several clusters of signature CRISPR-associated ( cas ) genes were identified to be well conserved and typically adjacent to the repeat elements ( Jansen et al., 2002 ), serving as a basis for the eventual classification of three different types of CRISPR systems (types I–III) ( Haft et al., 2005 ; Makarova et al., 2011b ). Types I and III CRISPR loci contain multiple Cas proteins, now known to form complexes with crRNA (CASCADE complex for type I; Cmr or Csm RAMP complexes for type III) to facilitate the recognition and destruction of target nucleic acids ( Brouns et al., 2008 ; Hale et al., 2009 ) ( Figure 4 ). In contrast, the type II system has a significantly reduced number of Cas proteins. However, despite increasingly detailed mapping and annotation of CRISPR loci across many microbial species, their biological significance remained elusive.
A key turning point came in 2005, when systematic analysis of the spacer sequences separating the individual direct repeats suggested their extrachromosomal and phage-associated origins ( Mojica et al., 2005 ; Pourcel et al., 2005 ; Bolotin et al., 2005 ). This insight was tremendously exciting, especially given previous studies showing that CRISPR loci are transcribed ( Tang et al., 2002 ) and that viruses are unable to infect archaeal cells carrying spacers corresponding to their own genomes ( Mojica et al., 2005 ). Together, these findings led to the speculation that CRISPR arrays serve as an immune memory and defense mechanism, and individual spacers facilitate defense against bacteriophage infection by exploiting Watson-Crick base-pairing between nucleic acids ( Mojica et al., 2005 ; Pourcel et al., 2005 ). Despite these compelling realizations that CRISPR loci might be involved in microbial immunity, the specific mechanism of how the spacers act to mediate viral defense remained a challenging puzzle. Several hypotheses were raised, including thoughts that CRISPR spacers act as small RNA guides to degrade viral transcripts in a RNAi-like mechanism ( Makarova et al., 2006 ) or that CRISPR spacers direct Cas enzymes to cleave viral DNA at spacer-matching regions ( Bolotin et al., 2005 ).
Working with the dairy production bacterial strain Streptococcus thermophilus at the food ingredient company Danisco, Horvath and colleagues uncovered the first experimental evidence for the natural role of a type II CRISPR system as an adaptive immunity system, demonstrating a nucleic-acid-based immune system in which CRISPR spacers dictate target specificity while Cas enzymes control spacer acquisition and phage defense ( Barrangou et al., 2007 ). A rapid series of studies illuminating the mechanisms of CRISPR defense followed shortly and helped to establish the mechanism as well as function of all three types of CRISPR loci inadaptive immunity. By studying the type I CRISPR locus of Escherichia coli , van der Oost and colleagues showed that CRISPR arrays are transcribed and converted into small crRNAs containing individual spacers to guide Cas nuclease activity ( Brouns et al., 2008 ). In the same year, CRISPR-mediated defense by a type III-A CRISPR system from Staphylococcus epidermidis was demonstrated to block plasmid conjugation, establishing the target of Cas enzyme activity as DNA rather than RNA ( Marraffini and Sontheimer, 2008 ), although later investigation of a different type III-B system from Pyrococcus furiosus also revealed crRNA-directed RNA cleavage activity ( Hale et al., 2009 , 2012 ).
As the pace of CRISPR research accelerated, researchers quickly unraveled many details of each type of CRISPR system ( Figure 4 ). Building on an earlier speculation that protospacer adjacent motifs (PAMs) may direct the type II Cas9 nuclease to cleave DNA ( Bolotin et al., 2005 ), Moineau and colleagues highlighted the importance of PAM sequences by demonstrating that PAM mutations in phage genomes circumvented CRISPR interference ( Deveau et al., 2008 ). Additionally, for types I and II, the lack of PAM within the direct repeat sequence within the CRISPR array prevents self-targeting by the CRISPR system. In type III systems, however, mismatches between the 5′ end of the crRNA and the DNA target are required for plasmid interference ( Marraffini and Sontheimer, 2010 ).
By 2010, just 3 years after the first experimental evidence for CRISPR in bacterial immunity, the basic function and mechanisms of CRISPR systems were becoming clear. A variety of groups had begun to harness the natural CRISPR system for various biotechnological applications, including the generation of phage-resistant dairy cultures ( Quiberoni et al., 2010 ) and phylogenetic classification of bacterial strains ( Horvath et al., 2008 , 2009 ). However, genome editing applications had not yet been explored.
Around this time, two studies characterizing the functional mechanisms of the native type II CRISPR system elucidated the basic components that proved vital for engineering a simple RNA-programmable DNA endonuclease for genome editing. First, Moineau and colleagues used genetic studies in Streptococcus thermophilus to reveal that Cas9 (formerly called Cas5, Csn1, or Csx12) is the only enzyme within the cas gene cluster that mediates target DNA cleavage ( Garneau et al., 2010 ). Next, Charpentier and colleagues revealed a key component in the biogenesis and processing of crRNA in type II CRISPR systems—a noncoding trans -activating crRNA (tracrRNA) that hybridizes with crRNA to facilitate RNA-guided targeting of Cas9 ( Deltcheva et al., 2011 ). This dual RNA hybrid, together with Cas9 and endogenous RNase III, is required for processing the CRISPR array transcript into mature crRNAs ( Deltcheva et al., 2011 ). These two studies suggested that there are at least three components (Cas9, the mature crRNA, and tracrRNA) that are essential for reconstituting the type II CRISPR nuclease system. Given the increasing importance of programmable site-specific nucleases based on ZFs and TALEs for enhancing eukaryotic genome editing, it was tantalizing to think that perhaps Cas9 could be developed into an RNA-guided genome editing system. From this point, the race to harness Cas9 for genome editing was on.
In 2011, Siksnys and colleagues first demonstrated that the type II CRISPR system is transferrable, in that transplantation of the type II CRISPR locus from Streptococcus thermophilus into Escherichia coli is able to reconstitute CRISPR interference in a different bacterial strain ( Sapranauskas et al., 2011 ). By 2012, biochemical characterizations by the groups of Charpentier, Doudna, and Siksnys showed that purified Cas9 from Streptococcus thermophilus or Streptococcus pyogenes can be guided by crRNAs to cleave target DNA in vitro ( Jinek et al., 2012 ; Gasiunas et al., 2012 ), in agreement with previous bacterial studies ( Garneau et al., 2010 ; Deltcheva et al., 2011 ; Sapranauskas et al., 2011 ). Furthermore, a single guide RNA (sgRNA) can be constructed by fusing a crRNA containing the targeting guide sequence to a tracrRNA that facilitates DNA cleavage by Cas9 in vitro ( Jinek et al., 2012 ).
In 2013, a pair of studies simultaneously showed how to successfully engineer type II CRISPR systems from Streptococcus thermophilus ( Cong et al., 2013 ) and Streptococcus pyogenes ( Cong et al., 2013 ; Mali et al., 2013a ) to accomplish genome editing in mammalian cells. Heterologous expression of mature crRNA-tracrRNA hybrids ( Cong et al., 2013 ) as well as sgRNAs ( Cong et al., 2013 ; Mali et al., 2013a ) directs Cas9 cleavage within the mammalian cellular genome to stimulate NHEJ or HDR-mediated genome editing. Multiple guide RNAs can also be used to target several genes at once. Since these initial studies, Cas9 has been used by thousands of laboratories for genome editing applications in a variety of experimental model systems ( Sander and Joung, 2014 ). The rapid adoption of the Cas9 technology was also greatly accelerated through a combination of open-source distributors such as Addgene, as well as a number of online user forums such as http://www.genome-engineering.org and http://www.egenome.org .
Structural Organization and Domain Architecture of Cas9
The family of Cas9 proteins is characterized by two signature nuclease domains, RuvC and HNH, each named based on homology to known nuclease domain structures ( Figure 2C ). Though HNH is a single nuclease domain, the full RuvC domain is divided into three subdomains across the linear protein sequence, with RuvC I near the N-terminal region of Cas9 and RuvC II/III flanking the HNH domain near the middle of the protein. Recently, a pair of structural studies shed light on the structural mechanism of RNA-guided DNA cleavage by Cas9.
First, single-particle EM reconstructions of the Streptococcus pyogenes Cas9 (SpCas9) revealed a large structural rearrangement between apo-Cas9 unbound to nucleic acid and Cas9 in complex with crRNA and tracrRNA, forming a central channel to accommodate the RNA-DNA heteroduplex ( Jinek et al., 2014 ). Second, a high-resolution structure of SpCas9 in complex with sgRNA and the complementary strand of target DNA further revealed the domain organization to comprise of an α-helical recognition (REC) lobe and a nuclease (NUC) lobe consisting of the HNH domain, assembled RuvC subdomains, and a PAM-interacting (PI) C-terminal region ( Nishimasu et al., 2014 ) ( Figure 5A and Movie S1 ).

(A) Crystal structure of Streptococcus pyogenes Cas9 in complex with guide RNA and target DNA.
(B) Canonical CRISPR locus organization from type II CRISPR systems, which can be classified into IIA-IIC based on their cas gene clusters. Whereas type IIC CRISPR loci contain the minimal set of cas9, cas1 , and cas2 , IIA and IIB retain their signature csn2 and cas4 genes, respectively.
(C) Histogram displaying length distribution of known Cas9 orthologs as described in UniProt, HAMAP protein family profile MF_01480.
(D) Phylogenetic tree displaying the microbial origin of Cas9 nucleases from the type II CRISPR immune system. Taxonomic information was derived from greengenes 16S rRNA gene sequence alignment, and the tree was visualized using the Interactive Tree of Life tool (iTol).
(E) Four Cas9 orthologs from families IIA, IIB, and IIC were aligned by ClustalW (BLOSUM). Domain alignment is based on the Streptococcus pyogenes Cas9, whereas residues highlighted in red indicate highly conserved catalytic residues within the RuvC I and HNH nuclease domains.
Together, these two studies support the model that SpCas9 unbound to target DNA or guide RNA exhibits an autoinhibited conformation in which the HNH domain active site is blocked by the RuvC domain and is positioned away from the REC lobe ( Jinek et al., 2014 ). Binding of the RNA-DNA heteroduplex would additionally be sterically inhibited by the orientation of the C-terminal domain. Asaresult, apo-Cas9 likely cannot bind nor cleave target DNA. Like many ribonucleoprotein complexes, the guide RNA serves as a scaffold around which Cas9 can fold and organize its various domains ( Nishimasu et al., 2014 ).
The crystal structure of SpCas9 incomplex with an sgRNA and target DNA also revealed how the REC lobe facilitates target binding. An arginine-rich bridge helix (BH) within the REC lobe is responsible for contacting the 3′ 8–12 nt of the RNA-DNA heteroduplex ( Nishimasu et al., 2014 ), which correspond with the seed sequence identified through guide sequence mutation experiments ( Jinek et al., 2012 ; Cong et al., 2013 ; Fu et al., 2013 ; Hsu et al., 2013 ; Pattanayak et al., 2013 ; Mali et al., 2013b ).
The SpCas9 structure also provides a useful scaffold for engineering or refactoring of Cas9 and sgRNA. Because the REC2 domain of SpCas9 is poorly conserved in shorter orthologs, domain recombination or truncation is a promising approach for minimizing Cas9 size. SpCas9 mutants lacking REC2 retain roughly 50% of wild-type cleavage activity, which could be partly attributed to their weaker expression levels ( Nishimasu et al., 2014 ). Introducing combinations of orthologous domain recombination, truncation, and peptide linkers could facilitate the generation of a suite of Cas9 mutant variants optimized for different parameters such as DNA binding, DNA cleavage, or overall protein size.
Metagenomic, Structural, and Functional Diversity of Cas9
Cas9 is exclusively associated with the type II CRISPR locus and serves as the signature type II gene. Based on the diversity of associated Cas genes, type IICRISPR loci are further subdivided into three subtypes (IIA–IIC) ( Figure 5B ) ( Makarova et al., 2011a ; Chylinski et al., 2013 ). Type II CRISPR loci mostly consist of the cas9, cas1 , and cas2 genes, as well as a CRISPR array and tracrRNA. Type IIC CRISPR systems contain only this minimal set of cas genes, whereas types IIA and IIB have an additional signature csn2 or cas4 gene, respectively ( Chylinski et al., 2013 ).
Subtype classification of type II CRISPR loci is based on the architecture and organization of each CRISPR locus. For example, type IIA and IIB loci usually consist of four cas genes, whereas type IIC loci only contain three cas genes. However, this classification does not reflect the structural diversity of Cas9 proteins, which exhibit sequence homology and length variability irrespective of the subtype classification of their parental CRISPR locus. Of >1,000 Cas9 nucleases identified from sequence databases (UniProt) based on homology, protein length israther heterogeneous, roughly ranging from 900 to 1600 amino acids ( Figure 5C ). The length distribution of most Cas9 proteins can be divided into two populations centered around 1,100 and 1,350 amino acids in length. It is worth noting that a third population of large Cas9 proteins belonging to subtype IIA, formerly called Csx12, typically contain around 1500 amino acids.
Despite the apparent diversity of protein length, all Cas9 proteins share similar domain architecture ( Makarova et al., 2011a ; Chylinski et al., 2013 , 2014; Fonfara et al., 2014 ), consisting of the RuvC and HNH nuclease domains and the REC domain, an α-helix-rich region with an Arg-rich bridge helix. Unlike type I and III CRISPR systems, which are found in both bacteria and archaea, type II CRISPRs have so far only been found in bacterial strains ( Chylinski et al., 2013 ). The majority of Cas9 orthologs in fact belong to the phyla of Bacteroidetes, Proteobacteria , and Firmicutes ( Figure 5D ).
The length difference among Cas9 proteins largely results from variable conservation of the REC domain ( Figure 5E ), which associates with the sgRNA and target DNA. For example, the type IIC Actinomyces naeslundii Cas9, which is more compact than its Streptococcus pyogenes ortholog, has a much smaller REC lobe with substantially different orientation ( Jinek et al., 2014 ).
Protospacer Adjacent Motif: Cas9 Target Range and Search Mechanism
A critical feature of the Cas9 system is the protospacer-adjacent motif (PAM), which flanks the 3′ end of the DNA target site ( Figure 2C ) and dictates the DNA target search mechanism of Cas9. In addition to facilitating self versus non-self discrimination by Cas9 ( Shah et al., 2013 ), because direct repeats do not contain PAM sites, biochemical and structural characterization of SpCas9 suggested that PAM recognition is involved in triggering the transition between Cas9 target binding and cleavage conformations ( Sternberg et al., 2014 ; Jinek et al., 2014 ; Nishimasu et al., 2014 ).
Single-molecule imaging indicated that Cas9-crRNA-tracrRNA complexes first associate with PAM sequences throughout the genome, allowing Cas9 to initiate DNA strand separation via unknown mechanisms ( Sternberg et al., 2014 ). DNA competitor cleavage assays additionally suggested that formation of the RNA-DNA heteroduplex is initiated at the PAM site before proceeding PAM distally by interrogating the target site upstream of the PAM for guide sequence complementarity ( Sternberg et al., 2014 ). Binding of the PAM and a matching target then triggers Cas9 nuclease activity by activating the HNH and RuvC domains, supported by the observation of HNH domain flexibility within the Cas9-sgRNA-DNA ternary complex ( Nishimasu et al., 2014 ).
The complexity of the PAM sequences also determines the overall DNA targeting space of Cas9. For example, the 5′-NGG of SpCas9 allows it to target, on average, every 8 bp within the human genome ( Cong et al., 2013 ; Hsu et al., 2013 ). Additionally, SpCas9 can target sites flanked by 5′-NAG PAMs ( Jiang et al., 2013 ; Hsu et al., 2013 ), albeit at a lower efficiency, further expanding its editing versatility. The PAM is specific to each Cas9 ortholog, even within the same species, such as 5′-NNA GAAW for Streptococcus thermophilus CRISPR1 ( Deveau et al., 2008 ) and 5′-NGGNG for Streptococcus thermophilus CRISPR3 ( Horvath et al., 2008 ). Another Cas9 from Neisseria meningitidis with a 5′-NNNNGATT PAM requirement ( Zhang et al., 2013 ) was recently applied in human pluripotent stem cells ( Hou et al., 2013 ).
Computational ( Chylinski et al., 2013 , 2014 ; Fonfara et al., 2014 ) or metagenomic analysis of bacteria and archaea containing CRISPR loci could lead to the discovery of Cas9 nucleases with additional PAMs to expand the targeting range of the Cas9 toolkit. Delivery of multiple Cas9 proteins with different PAM requirements facilitates orthogonal genome engineering, in which independent but simultaneous functions are applied at different loci within the same cell or cell population. NmCas9 and SpCas9, for example, can be employed for independent transcriptional repression and nuclease activity ( Esvelt et al., 2013 ).
PAM specificity can also be modified. For instance, orthologous replacement of the PAM-interacting (PI) domain from the Streptococcus thermophilus CRISPR3 Cas9 with the corresponding domain from Streptococcus pyogenes Cas9 successfully altered PAM recognition from 5′-NGGNG to 5′-NGG ( Nishimasu et al., 2014 ). PAM engineering strategies could also be exploited to generate short Cas9 orthologs with flexible 5′-NGG or 5′-NG PAM domains.
Genome Editing Using CRISPR-Cas9 in Eukaryotic Cells
To date, the Streptococcus pyogenes Cas9 (SpCas9) has been used broadly to achieve efficient genome editing in a variety of species and cell types, including human cell lines, bacteria, zebrafish, yeast, mouse, fruit fly, roundworm, rat, common crops, pig, and monkey (see Sander and Joung [2014] for a detailed list). SpCas9 is also dramatically expanding the catalog of genetically tractable model organisms, for example, by enabling the introduction of multiplex mutations in cynomolgus monkeys ( Niu et al., 2014 ).
SpCas9 can be targeted either with a pair of crRNA and tracrRNA ( Cong et al., 2013 ) or with a chimeric sgRNA ( Cong et al., 2013 ; Mali et al., 2013a ; Cho et al., 2013 ; Jinek et al., 2013 ), as the crRNA or sgRNA contains a 20 nt guide sequence that directly matches the target site. The only requirement for the selection of Cas9 target sites is the presence of a protospacer-adjacent motif (PAM) immediately downstream of the target site.
An early discrepancy in the use of SpCas9 editing of the human genome was the drastically higher levels of NHEJ-induced indels given the same target site, when using the engineered dual guide RNA system ( Cong et al., 2013 ) compared to the engineered sgRNA(+48) scaffold, which only contained up to the 48th base of tracrRNA. Although sgRNA(+48) is sufficient for cleaving DNA in vitro ( Jinek et al., 2012 ), extension of the 3′ tracrRNA sequence preserved several hairpin structures (sgRNA(+72) and sgRNA(+84)) that were critical for effective sgRNA-mediated genome editing in vivo ( Mali et al., 2013a ; Hsu et al., 2013 ). The additional stem loops enhance the stability of the sgRNA ( Hsu et al., 2013 ) and are important for proper Cas9-sgRNA-DNA ternary complex formation ( Nishimasu et al., 2014 ). These analyses of the sgRNA structure and function indicate that careful sgRNA design is critical for optimal Cas9 activity, especially for testing novel Cas9 candidates derived from metagenomic analysis.
One hallmark of the natural CRISPR-Cas9 system is its inherent ability to efficiently cleave multiple distinct target sequences in parallel ( Barrangou et al., 2007 ; Garneau et al., 2010 ) by converting a pre-crRNA transcript containing many spacers into individual guide RNAs duplexes (mature crRNA and tracrRNA) through hybridization with tracrRNA ( Deltcheva et al., 2011 ). Harnessing this unique aspect of CRISPR interference would enable highly scalable multiplex perturbations. Indeed, coexpression of a CRISPR array containing spacers targeting different genes ( Cong et al., 2013 ) or a battery of several sgRNAs ( Mali et al., 2013a ; Wang et al., 2013 ) together with SpCas9 has led to efficient multiplex editing in mammalian cells. Surprisingly, CRISPR arrays containing direct repeats interspaced by designer spacers were processed into mature guide RNAs without the introduction of bacterial RNase III. Because RNase III is required for crRNA maturation in prokaryotic cells ( Deltcheva et al., 2011 ), it is likely that endogenous mammalian RNases play compensatory roles ( Cong et al., 2013 ).
Specificity of Cas9 Nucleases
Because genome editing leads to permanent modifications within the genome, the targeting specificity of Cas9 nucleases is of particular concern, especially for clinical applications and gene therapy. A combination of in vitro and in vivo assays has been typically used to characterize the specificity of ZFNs and TALENs ( Gabriel et al., 2011 ), but systematic analysis has remained challenging due to difficulties in synthesizing large libraries of proteins with varying sequence specificity. However, Cas9 target recognition is dictated by the Watson-Crick base-pairing interactions of an RNA guide with its DNA target, enabling experimentally tractable and systematic evaluation of the effect of guide RNA-target DNA mismatches on Cas9 activity.
Streptococcus pyogenes Cas9 specificity has been extensively characterized by multiple groups using mismatched guide RNA libraries, in vitro selection, and reporter assays ( Fu et al., 2013 ; Hsu et al., 2013 ; Mali et al., 2013b ; Pattanayak et al., 2013 ). In contrast to previous studies that suggested a seed sequence model for Cas9 specificity, wherein the first 8–12 PAM-proximal guide sequence bases determine specificity ( Jinek et al., 2012 ; Cong et al., 2013 ), these studies collectively demonstrate that Cas9 tolerates mismatches throughout the guide sequence in a manner that is sensitive to the number, position, and distribution of the mismatches ( Fu et al., 2013 ; Hsu et al., 2013 ; Mali et al., 2013b ; Pattanayak et al., 2013 ). Although the PAM-distal bases of the guide sequence are less important for specificity, meaning that mismatches at those positions often do not abolish Cas9 activity, all positions within the guide contribute to overall specificity. Importantly, off-target sites followed by the 5′-NAG PAM can also lead to off-target cleavage, demonstrating the importance of considering both NGG and NAG PAMs in off-target analysis.
Interestingly, Cas9 requires extensive homology between the guide RNA and target DNA in order to cleave but can remain semi-transiently bound with only a short stretch of complementary sequence between the guide RNA and target DNA. These observations suggest that Cas9 has many off-target binding sites but cleaves only a small fraction of them ( Wu et al., 2014 ). Thus, concerns about off-target activity could vary widely given a desired application that exploits Cas9 for its DNA binding or cleavage capabilities.
Enzymatic concentration is also an important factor in determining Cas9 off-target mutagenesis. This is particularly important because Cas9 can tolerate even five mismatches within the target site ( Fu et al., 2013 ). Mismatches appear to be better tolerated when Cas9 is present at high concentrations ( Hsu et al., 2013 ; Pattanayak et al., 2013 ), leading to higher off-target activity; decreasing Cas9 concentration significantly improves the on- to off-target ratio at the expense of the efficiency of on-target cleavage ( Hsu et al., 2013 ). The duration of Cas9 expression is likely an additional factor that tunes off-target activity, though its contributions remain to be carefully investigated.
While potential off-target sites have typically been computationally determined by searching for genomic sequences with high sequence similarity to the desired target locus, wholegenome sequencing or other unbiased ways of labeling DNA DSBs genome-wide may illuminate off-target sites that are not predictable by first-order sequence comparison. Unbiased genome-wide characterizations have been previously used to characterize ZFN off-target mutagenesis ( Gabriel et al., 2011 ) and could easily be adapted for Cas9 nuclease activity. Such data, perhaps in combination with thermodynamic characterization of guide RNA and target DNA hybridization, will likely provide a quantitative framework for assessing and predicting the off-target activity of Cas9. Multiple groups now provide Cas9 target selection tools (e.g., http://tools.genome-engineering . org, http://zifit.partners.org , and http://www.e-crisp.org ).
Improving Cas9 Target Recognition Fidelity
Cas9 nucleases cleave DNA through the activity of their RuvC and HNH nuclease domains, each of which nicks a strand of DNA to generate a blunt-ended DSB ( Figure 2C ). SpCas9 can be converted into a DNA “nickase” that creates a single-stranded break (SSB) by catalytically inactivating the RuvC or HNH nuclease domains ( Gasiunas et al., 2012 ; Jinek et al., 2012 ; Sapranauskas et al., 2011 ) via point mutations ( Figure 6A ). Because DNA single-strand breaks are repaired via the high-fidelity base excision repair (BER) pathway ( Dianov and Hübscher, 2013 ), Cas9 nickases can be exploited for more specific NHEJ as well as HR.

(A) The Cas9 nuclease cleaves DNA via its RuvC and HNH nuclease domains, each ofwhich nicks a DNA strand to generate blunt-end DSBs. Either catalytic domain can be inactivated to generate nickase mutants that cause single-strand DNA breaks.
(B) Two Cas9 nickase complexes with appropriately spaced target sites can mimic targeted DSBs via cooperative nicks, doubling the length of target recognition without sacrificing cleavage efficiency.
(C) Expression plasmids encoding the Cas9 gene and a short sgRNA cassette driven by the U6 RNA polymerase III promoter can be directly transfected into cell lines of interest.
(D) Purified Cas9 protein and in vitro transcribed sgRNA can be microinjected into fertilized zygotes for rapid generation of transgenic animal models.
(E) For somatic genetic modification, high-titer viral vectors encoding CRISPR reagents can be transduced into tissues or cells of interest.
(F) Genome-scale functional screening can be facilitated by mass synthesis and delivery of guide RNA libraries.
(G) Catalytically dead Cas9 (dCas9) can be converted into a general DNA-binding domain and fused to functional effectors such as transcriptional activators or epigenetic enzymes. The modularity of targeting and flexible choice of functional domains enable rapid expansion of the Cas9 toolbox.
(H) Cas9 coupled to fluorescent reporters facilitates live imaging of DNA loci for illuminating the dynamics of genome architecture.
(I) Reconstituting split fragments of Cas9 via chemical or optical induction of heterodimer domains, such as the cib1/cry2 system from Arabidopsis , confers temporal control of dynamic cellular processes.
To improve on-target DSB specificity, a double-nicking approach analogous to dimeric ZFNs or TALENs can be used to increase the overall number of bases that are specifically recognized in the target DNA. Using pairs of guide RNAs ( Mali et al., 2013b ; Ran et al.,2013)and an SpCas9 HNH + /RuvC − nickase mutant (D10A), properly spaced cooperative nicks can mimic DSBs and mediate efficient indel formation ( Figure 6B ). Because off-target nick sites are precisely repaired, this multiplexed nicking strategy can improve specificity by up to 1,500× relative to the wild-type Cas9 ( Ran et al., 2013 ).
Because Cas9 nuclease or multiplex nicking activity both stimulate NHEJ, a population of cells cotargeted with a homology donor would eventually possess a mix of indel mutants and donor integrants. Single DNA nicks, however, are also able to mediate donor recombination, albeit at a lower level than with DSBs ( Hsu et al., 2013 ). Cas9 nickases with single sgRNAs can thus be used to mediate HR rather than NHEJ. Furthermore, off-target integration is highly unlikely due to long homology arms flanking the donor cassette.
In addition to the double-nicking strategy, sgRNAs truncated by 2 or 3 nt have been reported to significantly increase targeting specificity of SpCas9, potentially due to greater mismatch sensitivity ( Fu et al., 2014 ). These truncated sgRNAs can be combined with multiplex nicking to further reduce off-target mutagenesis ( Fu et al., 2014 ). Future structure-function analyses and Cas9 and protein engineering via rational design or directed evolution may lead to further improvements in Cas9 specificity.
Applications of Cas9 in Research, Medicine, and Biotechnology
Cas9 can be used to facilitate a wide variety of targeted genome engineering applications. The wild-type Cas9 nuclease has enabled efficient and targeted genome modification in many species that have been intractable using traditional genetic manipulation techniques. The ease of retargeting Cas9 by simply designing a short RNA sequence also enables large-scale unbiased genome perturbation experiments to probe gene function or elucidate causal genetic variants. In addition to facilitating co-valent genome modifications, the wild-type Cas9 nuclease can also be converted into a generic RNA-guided homing device (dCas9) by inactivating the catalytic domains. The use of effector fusions can greatly expand the repertoire of genome engineering modalities achievable using Cas9. For example, a variety of proteins or RNAs can be tethered to Cas9 or sgRNA to alter transcription states of specific genomic loci, monitor chromatin states, or even rearrange the three-dimensional organization of the genome.
Rapid Generation of Cellular and Animal Models
Cas9-mediated genome editing has enabled accelerated generation of transgenic models and expands biological research beyond traditional, genetically tractable animal model organisms ( Sander and Joung, 2014 ). By recapitulating genetic mutations found in patient populations, CRISPR-based editing could be used to rapidly model the causal roles of specific genetic variations instead of relying on disease models that only phenocopy a particular disorder. This could be applied to develop novel transgenic animal models ( Wang et al., 2013 ; Niu et al., 2014 ), to engineer isogenic ES and iPS cell disease models with specific mutations introduced or corrected, respectively, or in vivo and ex vivo gene correction ( Schwank et al., 2013 ; Wu et al., 2013 ).
For generation of cellular models, Cas9 can be easily introduced into the target cells using transient transfection of plasmids carrying Cas9 and the appropriately designed sgRNA ( Figure 6C ). Additionally, the multiplexing capabilities of Cas9 offer a promising approach for studying common human diseases—such as diabetes, heart disease, schizophrenia, and autism—that are typically polygenic. Large-scale genome-wide association studies (GWAS), for example, have identified haplotypes that show strong association with disease risk. However, it is often difficult to determine which of several genetic variants in tight linkage disequilibrium with the haplotype or which of several genes in the region are responsible for the phenotype. Using Cas9, one could study the effect of each individual variant or test the effect of manipulating each individual gene on an isogenic background by editing stem cells and differentiating them into cell types of interest.
For generation of transgenic animal models, Cas9 protein and transcribed sgRNA can be directly injected into fertilized zygotes to achieve heritable gene modification at one or multiple alleles in models such as rodents and monkeys ( Wang et al., 2013 ; Li et al., 2013 ; Yang et al., 2013 ; Niu et al., 2014 ) ( Figure 6D ). By bypassing the typical ES cell targeting stage in generating transgenic lines, the generation time for mutant mice and rats can be reduced from more than a year to only several weeks. Such advances will facilitate cost-effective and large-scale in vivo mutagenesis studies in rodent models and can be combined with highly specific editing ( Fu et al., 2014 ; Ran et al., 2013 ) to avoid confounding off-target mutagenesis. Successful multiplex targeting in cynomolgus monkey models was also recently reported ( Niu et al., 2014 ), suggesting the potential for establishing more accurate modeling of complex human diseases such as neuropsychiatric disorders using primate models. Additionally, Cas9 could be harnessed for direct modification of somatic tissue, obviating the need for embryonic manipulation ( Figure 6E ) as well as enabling therapeutic use for gene therapy.
One outstanding challenge with transgenic animal models generated via zygotic injection of CRISPR reagents is genetic mosaicism, partly due to a slow rate of nuclease-induced mutagenesis. Studies to date have typically relied on the injection of Cas9 mRNA into zygotes (fertilized embryos at the single-cell stage). However, because transcription and translation activity is suppressed in the mouse zygote, Cas9 mRNA translation into active enzymatic form is likely delayed until after the first cell division ( Oh et al., 2000 ). Because NHEJ-mediated repair is thought to introduce indels of random length, this translation delay likely plays a major role in contributing to genetic mosaicism in CRISPR-modified mice. To overcome this limitation, Cas9 protein and sgRNA could be directly injected into single-cell fertilized embryos. The high rate of nonmutagenic repair by the NHEJ process may additionally contribute to undesired mosaicism because introducing indels that mutate the Cas9 recognition site would then have to compete with zygotic division rates. To increase the mutagenic activity of NHEJ, a pair of sgRNAs flanking a small fragment of the target gene may be used to increase the probability of gene disruption.
Functional Genomic Screens
The efficiency of genome editing with Cas9 makes it possible to alter many targets in parallel, thereby enabling unbiased genome-wide functional screens to identify genes that play an important role in a phenotype of interest. Lentiviral delivery of sgRNAs directed against all genes (either together with Cas9 or to cells already expressing Cas9) can be used to perturb thousands of genomic elements in parallel. Recent papers have demonstrated the ability to perform robust negative and positive selection screens in human cells ( Wang et al., 2014 ; Shalem et al., 2014 ) by introducing loss-of-function mutations into early, constitutive coding exons of a different gene in each cell ( Figure 6F ). Genome-wide loss-of-function screens have previously used RNAi, but this approach leads to only partial knockdown, has extensive off-target effects, and is limited to transcribed (and usually protein-coding) genes. By contrast, Cas9-mediated pooled sgRNA screens have been shown to provide increased screening sensitivity as well as consistency and can be designed to target nearly any DNA sequence ( Shalem et al., 2014 ).
Future applications of single sgRNA libraries may also enable the perturbation of noncoding genetic elements, while multiplex sgRNA delivery may be used to dissect the function of large genomic regions through tiled microdeletions. For example, systematic targeting of gene regulatory regions could facilitate the discovery of distant enhancers, general promoter architectures, and any additional regulatory elements that have an effect on protein levels. An additional application could be to dissect large, uncharacterized genomic regions that are implicated in sequencing studies or GWAS.
Tethering dCas9 to different effector domains may also facilitate genomic screens beyond loss-of-function phenotypes. dCas9 fused to epigenetic modifiers, for instance, could be used to study the effects of methylation or certain chromatin states on cellular differentiation or disease pathologies, whereas transcriptional activators allow screening for gain-of-function phenotypes. Using truncated sgRNAs or building redundancy with several sgRNAs targeting each locus would be important design principles for filtering out false positive signals and improving the interpretability of screening data.
Transcriptional Modulation
dCas9 binding alone to DNA elements may repress transcription by sterically hindering RNA polymerase machinery ( Qi et al., 2013 ), likely by stalling transcriptional elongation. This CRISPR-based interference, or CRISPRi, works efficiently in prokaryotic genomes but is less effective in eukaryotic cells ( Gilbert et al., 2013 ). The repressive function of CRISPRi can be enhanced by tethering dCas9 to transcriptional repressor domains such as KRAB or SID effectors, which promote epigenetic silencing ( Gilbert et al., 2013 ; Konermann et al., 2013 ). However, dCas9-mediated transcriptional repression needs to be further improved—in the current generation of dCas9-based eukaryotic transcription repressors, even the addition of helper functional domains results in only partial transcriptional knockdown ( Gilbert et al., 2013 ; Konermann et al., 2013 ).
Cas9 can also be converted into a synthetic transcriptional activator by fusing it to VP16/VP64 or p65 activation domains ( Figure 6G ). In general, targeting Cas9 activators with a single sgRNA to a particular endogenous gene promoter leads to only modest transcriptional upregulation ( Konermann et al., 2013 ; Maeder et al., 2013b; Perez-Pinera et al., 2013 ; Mali et al., 2013b ). By tiling a promoter with multiple sgRNAs, several groups have reported strong synergistic effects with nonlinear increases in activation ( Perez-Pinera et al., 2013 ; Maeder et al., 2013b ; Mali et al., 2013b ). Although the requirement for multiple sgRNAs to achieve efficient transcription activation is potentially advantageous for increased specificity, screening applications employing libraries of sgRNAs will require highly efficient and specific transcriptional control using individual guide RNAs.
Epigenetic Control
Complex genome functions are defined by the highly dynamic landscape of epigenetic states. Epigenetic modifications that tune histones are thus crucial for transcriptional regulation and play important roles in a variety of biological functions. These marks, such as DNA methylation or histone acetylation, are established and maintained in mammalian cells by a variety of enzymes that are recruited to specific genomic loci either directly or indirectly through scaffolding proteins.
Previously, zinc finger proteins and TAL effectors have been used in a small number of proof-of-concept studies to achieve locus-specific targeting of epigenetic modifying enzymes ( Beerli et al., 2000a ; Konermann et al., 2013 ; Maeder et al., 2013a ; Mendenhall et al., 2013 ). Cas9 epigenetic effectors (epiCas9s) that can artificially install or remove specific epigenetic marks at specific loci would serve as a more flexible platform to probe the causal effects of epigenetic modifications in shaping the regulatory networks of the genome ( Figure 6G ). Of course, the potential for off-target activity or crosstalk between effector domains and endogenous epigenetic complexes would need to be carefully characterized. One solution could be to harness prokaryotic epigenetic enzymes to develop orthogonal epigenetic regulatory systems that minimize crosstalk with endogenous proteins.
Live Imaging of the Cellular Genome
The spatial organization of functional and structural elements within the cell contribute to the functional output of genomes, which can be amplified or suppressed dynamically. However, the way that genomes are modified and how their structural organization in vivo modulates functional output remain unclear. Genomic loci located megabases apart or on entirely different chromosomes could be brought into close proximity given appropriate chromosomal organization, thus mediating long-range trans interactions.
Studying the interactions of specific genes given changing chromatin states would require a robust method to visualize DNA in living cells. Traditional techniques for labeling DNA, such as fluorescence in situ hybridization (FISH), require sample fixation and are thus unable to capture live processes. Fluorescently tagged Cas9 labeling of specific DNA loci was recently developed as a powerful live-cell-imaging alternative to DNA-FISH ( Chen et al., 2013 ) ( Figure 6H ). Advances in orthogonal Cas9 proteins or modified sgRNAs will build out multi-color and multi-locus capabilities to enhance the utility of CRISPR-based imaging for studying complex chromosomal architecture and nuclear organization.
Inducible Regulation of Cas9 Activity
By exploiting the bilobed structure of Cas9, it may be possible to split the protein into two units and control their reassembly via small-molecule or light-inducible heterodimeric domains ( Figure 6I ). Small-molecule induction would facilitate systemic control of Cas9 in patients or animal models, whereas optical regulation enables more spatially precise perturbation. For example, the light-inducible dimerization domains CIB1 and CRY2 or chemically inducible analogs ABI and PYL, which have been successfully used to construct inducible TALEs (Konermann et al., 2013), may be adapted to engineer inducible Cas9 systems.
Future Development of Cas9-Based Genome Engineering Technologies
Unbiased analysis of cas9 binding and cleavage.
Despite the rapid adoption of Cas9 as a platform technology for genetic and epigenetic perturbation and significant progress in understanding and improving Cas9 specificity, its on- and off-target DNA binding and cleavage profiles still need to be thor oughly evaluated. Studies to date characterizing Cas9 off-target activity have relied on in silico computational prediction or in vitro selection. As a result, they have been unable to account for the likelihood of Cas9 activity that is unpredictable by sequence ho-mology to the sgRNA guide sequence.
Because the off-target activity of dCas9 binding for effector domain localization may be much more extensive than Cas9-mediated genome editing, unbiased profiling methods are needed to refine our understanding of “true positive” Cas9 off-target activity that actually leads to undesired functional outcomes. Cas9-based chromatin immunoprecipitation sequencing (ChIP-seq) analysis at multiple target sites could be a high-throughput solution for understanding binding degen eracy ( Wu et al., 2014 ), whereas techniques for detecting and labeling double-strand breaks ( Crosetto et al., 2013 ) will help to achieve a comprehensive map of Cas9-induced off-target indels.
These data together will help to generate predictive models for minimizing off-target activity in gene therapeutics or other applications requiring high levels of precision. Understanding Cas9 binding and cleavage in the context of chromatin accessibility and epigenetic states will also inform better computational evaluation of guide RNA specificity. For example, particular sgRNAs could be evaluated based on the genomic nature of its off-target sites, which would vary by guide sequence. Degenerate targeting of transcriptionally silent genes for a cell type or tissue of interest would likely be preferred to off-target sites in the coding region of essential housekeeping genes.
Although it is still unclear how Cas9 is affected by chromatin accessibility and heterochromatin versus euchromatin, dCas9 transcriptional activators can upregulate transcription at sites lacking DNase I hypersensitivity sites, indicating successful binding to inaccessible chromatin ( Perez-Pinera et al., 2013 ) ( Figure 6G ). CpG methylation does not appear to affect DNA cleavage in vitro, and Cas9 could introduce indels at a highly methylated promoter in vivo ( Hsu et al., 2013 ). It will be important to evaluate Cas9 binding and cleavage of genomic loci in relevant primary cells with different chromatin states, ideally in post-mitotic cells in which genomic architecture is stably defined.
Overall, these efforts aimed at improving our understanding of Cas9 binding and cleavage specificity will complement existing methods ( Mali et al., 2013b ; Ran et al., 2013 ; Fu et al., 2014 ) as well as future protein engineering and metagenomic mining efforts to improve Cas9 specificity and the selection of guide RNA target sites.
Development of Versatile Delivery and Expression Systems for Applications of Cas9
Viral vectors such as adeno-associated virus (AAV) or lentivirus are commonly used for delivering genes of interest in vivo or into cell types resistant to common transfection methods, such as immune cells. AAV vectors have been commonly used for attractive candidates for efficient gene delivery in vivo because of their low immunogenic potential, reduced oncogenic risk from host-genome integration, and well-characterized serotype specificity ( Figure 6E ). However, the most commonly used Cas9 nuclease-encoding gene from Streptococcus pyogenes is >4 kb in length, which is difficult to transduce using AAV due to its 4.7 kb packaging capacity. Non-viral approaches for introducing CRISPR reagents in vivo present a fertile ground for developing novel delivery strategies, from liposomes and aptamers to cell-penetrating peptides and the molecular Trojan horse ( Niewoehner et al., 2014 ).
However, viral approaches are still highly desirable due to their low immunogenicity and wide array of characterized tropisms. The size constraints of viral vectors can be sidestepped by using significantly smaller Cas9 orthologs derived from metagenomic discovery, several of which have already been characterized and validated in human cells ( Cong et al., 2013 ; Hou et al., 2013 ; Esvelt et al., 2013 ). Interestingly, short Cas9 variants reported to date recognize much longer PAM sequences than SpCas9 (5′-NNAGAAW from Streptococcus thermophilus CRISPR1 or 5′-NNNNGATT from Neisseria meningitidis ) ( Zhang et al., 2013 ; Garneau et al., 2010 ), whereas some longer orthologs have more relaxed PAMs (5′-NG from Francisella novicida ) ( Fonfara et al., 2014 ). Although the effect of PAM restriction on DNA targeting specificity remains to be investigated, the more limited overall targeting range of short Cas9 variants may be partially compensated for by decreasing the number of potential off-target substrates genome-wide.
Moving beyond Endogenous Cellular Repair
The current generation of genome editing technologies depends on the endogenous DNA repair machinery to introduce loss-of-function mutations or precise modifications ( Figure 2A ). Although Cas9 can be used to generate indel mutations via NHEJ with high efficiency, the absolute rate of HDR remains relatively low. Although it is sufficient for the generation of cell lines, especially when paired with drug selection or FACS enrichment, poor rates of recombination greatly limit the practical utility of Cas9-mediated targeted gene insertion in fertilized zygotes or somatic tissue. Homologous recombination proteins are also mainly expressed in the G2 phase of the cell cycle, making HDR-based gene editing difficult in postmitotic cells such as neurons or cardiac myocytes. As a result, methods for stimulating HDR-based repair or alternative strategies for efficient gene insertion are urgently needed. For instance, the highly efficient DNA damage repair system in Deinococcus radiodurans ( Zahradka et al., 2006 ) may be exploited to enable efficient genome editing in mitotic as well as postmitotic cells.
Furthermore, the majority of CRISPR-based technology development has focused on the signature Cas9 nuclease from type II CRISPR systems. However, there remains a wide diversity of CRISPR types and functions. Cas RAMP module (Cmr) proteins identified in Pyrococcus furiosus and Sulfolobus solfataricus ( Hale et al., 2012 ) constitute an RNA-targeting CRISPR immune system, forming a complex guided by small CRISPR RNAs that target and cleave complementary RNA instead of DNA. Cmr protein homologs can be found throughout bacteria and archaea, typically relying on a 5 ′ site tag sequence on the target-matching crRNA for Cmr-directed cleavage.
Unlike RNAi, whichis targeted largely by a 6 nt seed region and to a lesser extent 13 other bases, Cmr crRNAs contain 30–40 nt of target complementarity. Cmr-CRISPR technologies for RNA targeting are thus a promising target for orthogonal engineering and minimal off-target modification. Although the modularity of Cmr systems for RNA-targeting in mammalian cells remains to be investigated, Cmr complexes native to P. furiosus have already been engineered to target novel RNA substrates ( Hale et al., 2009 , 2012 ).
Cas9 as a Therapeutic Molecule for Treating Genetic Disorders
Although Cas9 has already been widely used as a research tool, a particularly exciting future direction is the development of Cas9 as a therapeutic technology for treating genetic disorders. For a monogenic recessive disorder due to loss-of-function mutations (such as cystic fibrosis, sickle-cell anemia, or Duchenne muscular dystrophy), Cas9 may be used to correct the causative mutation. This has many advantages over traditional methods of gene augmentation that deliver functional genetic copies via viral vector-mediated overexpression—particularly that the newly functional gene is expressed in its natural context. For dominant-negative disorders in which the affected gene is haplosufficient (such as transthyretin-related hereditary amyloidosis or dominant forms of retinitis pigmentosum), it may also be possible to use NHEJ to inactivate the mutated allele to achieve therapeutic benefit. For allele-specific targeting, one could design guide RNAs capable of distinguishing between single-nucleotide polymorphism (SNP) variations in the target gene, such as when the SNP falls within the PAM sequence.
Some monogenic diseases also result from duplication of genomic sequences. For these diseases, the multiplexing capability of Cas9 may be exploited for deletion of the duplicated elements. For example, trinucleotide repeat disorders could be treated using two simultaneous DSBs to excise the repeat region. The success of this strategy will likely be higher for diseases such as Friedreich's ataxia, in which duplications occur in noncoding regions of the target gene, because NHEJ-mediated repair may lead to imperfect or frameshifted repair junctions.
In addition to repairing mutations underlying inherited disorders, Cas9-mediated genome editing might be used to introduce protective mutations in somatic tissues to combat nongenetic or complex diseases. For example, NHEJ-mediated inactivation of the CCR5 receptor in lymphocytes ( Lombardo et al., 2007 ) may be a viable strategy for circumventing HIV infection, whereas deletion of PCSK9 ( Cohenet al., 2005 ) orangiopoietin ( Musunuru et al., 2010 ) may provide therapeutic effects against statin-resistant hypercholesterolemia or hyperlipidemia. Although these targets may be also addressed using siRNA-mediated protein knockdown, a unique advantage of NHEJ-mediated gene inactivation is the ability to achieve permanent therapeutic benefit without the need for continuing treatment. As with all gene therapies, it will of course be important to establish that each proposed therapeutic use has a favorable benefit-risk ratio.
Cas9 could be used beyond the direct genome modification of somatic tissue, such as for engineering therapeutic cells. Chimeric antigen receptor (CAR) T cells can be modified ex vivo and reinfused into a patient to specifically target certain cancers ( Couzin-Frankel, 2013 ). The ease of design and testing of Cas9 may also facilitate the treatment of highly rare genetic variants through personalized medicine. Supporting these tremendous possibilities are a number of animal model studies as well as clinical trials using programmable nucleases that already provide important insights into the future development of Cas9-based therapeutics.
Recently, hydrodynamic delivery of plasmid DNA encoding Cas9 and sgRNA along with a repair template into the liver of an adult mouse model of tyrosinemia was shown to be able to correct the mutant Fah gene and rescue expression of the wild-type Fah protein in ∼1 out of 250 cells ( Yin et al., 2014 ). In addition, clinical trials successfully used ZF nucleases to combat HIV infection by ex vivo knockout of the CCR5 receptor. In all patients, HIV DNA levels decreased, and in one out of four patients, HIV RNA became undetectable ( Tebas et al., 2014 ). Both of these results demonstrate the promise of programmable nucleases as a new therapeutic platform.
However, numerous challenges still lie ahead. Most importantly, successful clinical translation will depend on appropriate and efficacious delivery systems to target specific disease tissues. To achieve high levels of therapeutic efficacy and simultaneously address a broad spectrum of genetic disorders, homologous recombination efficiency will need to be significantly improved. Although permanent genome modification has advantages over monoclonal antibody or siRNA treatments, which require repeated administration of the therapeutic molecule, the long-term implications remain unclear. As researchers further develop and test Cas9 toward clinical translation, it will be paramount to thoroughly characterize the safety as well as physiological effects of Cas9 using a variety of preclinical models.
Conclusions
The story of how a mysterious prokaryotic viral defense system became one of the most powerful and versatile platforms for engineering biology highlights the importance of basic science research. Just as recombinant DNA technology benefited from basic investigation of the restriction enzymes that are central to warfare between phage and bacteria, the latest generation of Cas9-based genome engineering tools are also based on components from the microbial antiphage defense system. It is highly likely that the future solutions for efficient and precise gene modification will be found in as of yet unexplored corners of the rich biological diversity of nature.
Supplementary Material
Supplementary movie, acknowledgments.
We gratefully acknowledge Sigrid Knemeyer for help with illustration; Ian Slay-maker for structural guidance; Chengwei Luo for expertise in phylogenetic analysis; Emmanuelle Charpentier, Philippe Horvath, Charles Jennings, Ellen Law, Luciano Marraffini, Francisco Mojica, Hiroshi Nishimasu, Virginijus Siksnys, and Alexandro Trevino for discussion and comments; and the CRISPR community for this beautiful story. P.D.H. is a James Mills Pierce Fellow. This work is supported by the NIMH through a NIH Director's Pioneer Award (DP1-MH100706), the NINDS through a NIH Transformative R01 grant (R01-NS 07312401), NSF, the Keck, McKnight, Damon Runyon, Searle Scholars, Klingenstein, Vallee, Merkin, and Simons Foundations, and Bob Metcalfe. CRISPR reagents are available to the academic community through Addgene, and associated protocols, support forum, and computational tools are available via the Zhang lab website ( http://www.genome-engineering.org ).
Supplemental Information Supplemental Information includes one movie and can be found with this article at http://dx.doi.org/10.1016/j.cell.2014.05.010 .
- Barrangou R, van der Oost J. CRISPR-Cas Systems: RNA-Mediated Adaptive Immunity in Bacteria and Archaea. Heidelberg, Germany: Springer; 2013. [ Google Scholar ]
- Barrangou R, Fremaux C, Deveau H, Richards M, Boyaval P, Moineau S, Romero DA, Horvath P. CRISPR provides acquired resistance against viruses in prokaryotes. Science. 2007; 315 :1709–1712. [ PubMed ] [ Google Scholar ]
- Beerli RR, Dreier B, Barbas CF., 3rd Positive and negative regulation of endogenous genes by designed transcription factors. Proc Natl Acad Sci USA. 2000a; 97 :1495–1500. [ PMC free article ] [ PubMed ] [ Google Scholar ]
- Beerli RR, Schopfer U, Dreier B, Barbas CF., 3rd Chemically regulated zinc finger transcription factors. J Biol Chem. 2000b; 275 :32617–32627. [ PubMed ] [ Google Scholar ]
- Bibikova M, Carroll D, Segal DJ, Trautman JK, Smith J, Kim YG, Chandrasegaran S. Stimulation of homologous recombination through targeted cleavage by chimeric nucleases. Mol Cell Biol. 2001; 21 :289–297. [ PMC free article ] [ PubMed ] [ Google Scholar ]
- Bibikova M, Golic M, Golic KG, Carroll M. Targeted chromosomal cleavage and mutagenesis in Drosophila using zinc-finger nucleases. Genetics. 2002; 161 :1169–1175. [ PMC free article ] [ PubMed ] [ Google Scholar ]
- Bibikova M, Beumer K, Trautman JK, Carroll D. Enhancing gene targeting with designed zinc finger nucleases. Science. 2003; 300 :764. [ PubMed ] [ Google Scholar ]
- Boch J, Scholze H, Schornack S, Landgraf A, Hahn S, Kay S, Lahaye T, Nickstadt A, Bonas U. Breaking the code of DNA binding specificity of TAL-type III effectors. Science. 2009; 326 :1509–1512. [ PubMed ] [ Google Scholar ]
- Bolotin A, Quinquis B, Sorokin A, Ehrlich SD. Clustered regularly interspaced short palindrome repeats (CRISPRs) have spacers of extra-chromosomal origin. Microbiology. 2005; 151 :2551–2561. [ PubMed ] [ Google Scholar ]
- Brouns SJ, Jore MM, Lundgren M, Westra ER, Slijkhuis RJ, Snijders AP, Dickman MJ, Makarova KS, Koonin EV, van der Oost J. Small CRISPR RNAs guide antiviral defense in prokaryotes. Science. 2008; 321 :960–964. [ PMC free article ] [ PubMed ] [ Google Scholar ]
- Capecchi MR. Altering the genome by homologous recombination. Science. 1989; 244 :1288–1292. [ PubMed ] [ Google Scholar ]
- Chen B, Gilbert LA, Cimini BA, Schnitzbauer J, Zhang W, Li GW, Park J, Blackburn EH, Weissman JS, Qi LS, Huang B. Dynamic imaging of genomic loci in living human cells by an optimized CRISPR/Cas system. Cell. 2013; 155 :1479–1491. [ PMC free article ] [ PubMed ] [ Google Scholar ]
- Cho SW, Kim S, Kim JM, Kim JS. Targeted genome engineering in human cells with the Cas9 RNA-guided endonuclease. Nat Biotechnol. 2013; 31 :230–232. [ PubMed ] [ Google Scholar ]
- Choulika A, Perrin A, Dujon B, Nicolas JF. Induction of homologous recombination inmammalian chromosomesby using the I-SceI system of Saccharomyces cerevisiae. Mol Cell Biol. 1995; 15 :1968–1973. [ PMC free article ] [ PubMed ] [ Google Scholar ]
- Christian M, Cermak T, Doyle EL, Schmidt C, Zhang F, Hummel A, Bogdanove AJ, Voytas DF. Targeting DNA double-strand breaks with TAL effector nucleases. Genetics. 2010; 186 :757–761. [ PMC free article ] [ PubMed ] [ Google Scholar ]
- Chylinski K, Le Rhun A, Charpentier E. The tracrRNA and Cas9 families of type II CRISPR-Cas immunity systems. RNA Biol. 2013; 10 :726–737. [ PMC free article ] [ PubMed ] [ Google Scholar ]
- Chylinski K, Makarova KS, Charpentier E, Koonin EV. Classification and evolution of type II CRISPR-Cas systems. Nucleic Acids Res. 2014 Published online Apr 11, 2014. http://dx.doi.org/10.1093/nar/gku241 . [ PMC free article ] [ PubMed ]
- Cohen J, Pertsemlidis A, Kotowski IK, Graham R, Garcia CK, Hobbs HH. Low LDL cholesterol in individuals of African descent resulting from frequent nonsense mutations in PCSK9. Nat Genet. 2005; 37 :161–165. [ PubMed ] [ Google Scholar ]
- Cong L, Ran FA, Cox D, Lin S, Barretto R, Habib N, Hsu PD, Wu X, Jiang W, Marraffini LA, Zhang F. Multiplex genome engineering using CRISPR/Cas systems. Science. 2013; 339 :819–823. [ PMC free article ] [ PubMed ] [ Google Scholar ]
- Couzin-Frankel J. Breakthrough of the year 2013. Cancer immunotherapy. Science. 2013; 342 :1432–1433. [ PubMed ] [ Google Scholar ]
- Crosetto N, Mitra A, Silva MJ, Bienko M, Dojer N, Wang Q, Karaca E, Chiarle R, Skrzypczak M, Ginalski K, et al. Nucleotide-resolution DNA double-strand break mapping by next-generation sequencing. Nat Methods. 2013; 10 :361–365. [ PMC free article ] [ PubMed ] [ Google Scholar ]
- Deltcheva E, Chylinski K, Sharma CM, Gonzales K, Chao Y, Pirzada ZA, Eckert MR, Vogel J, Charpentier E. CRISPR RNA maturation by trans-encoded small RNA and host factor RNase III. Nature. 2011; 471 :602–607. [ PMC free article ] [ PubMed ] [ Google Scholar ]
- Deveau H, Barrangou R, Garneau JE, Labonté J, Fremaux C, Boyaval P, Romero DA, Horvath P, Moineau S. Phage response to CRISPR-encoded resistance in Streptococcus thermophilus. J Bacteriol. 2008; 190 :1390–1400. [ PMC free article ] [ PubMed ] [ Google Scholar ]
- Dianov GL, Hübscher U. Mammalian base excision repair: the forgotten archangel. Nucleic Acids Res. 2013; 41 :3483–3490. [ PMC free article ] [ PubMed ] [ Google Scholar ]
- Esvelt KM, Mali P, Braff JL, Moosburner M, Yaung SJ, Church GM. Orthogonal Cas9 proteins for RNA-guided gene regulation and editing. Nat Methods. 2013; 10 :1116–1121. [ PMC free article ] [ PubMed ] [ Google Scholar ]
- Fonfara I, Le Rhun A, Chylinski K, Makarova KS, Lécrivain AL, Bzdrenga J, Koonin EV, Charpentier E. Phylogeny of Cas9 determines functional exchangeability of dual-RNA and Cas9 among orthologous type II CRISPR-Cas systems. Nucleic Acids Res. 2014; 42 :2577–2590. [ PMC free article ] [ PubMed ] [ Google Scholar ]
- Fu Y, Foden JA, Khayter C, Maeder ML, Reyon D, Joung JK, Sander JD. High-frequency off-target mutagenesis induced by CRISPR-Cas nucleases in human cells. Nat Biotechnol. 2013; 31 :822–826. [ PMC free article ] [ PubMed ] [ Google Scholar ]
- Fu Y, Sander JD, Reyon D, Cascio VM, Joung JK. Improving CRISPR-Cas nuclease specificity using truncated guide RNAs. Nat Biotechnol. 2014; 32 :279–284. [ PMC free article ] [ PubMed ] [ Google Scholar ]
- Gabriel R, Lombardo A, Arens A, Miller JC, Genovese P, Kaeppel C, Nowrouzi A, Bartholomae CC, Wang J, Friedman G, et al. An unbiased genome-wide analysis of zinc-finger nuclease specificity. Nat Biotechnol. 2011; 29 :816–823. [ PubMed ] [ Google Scholar ]
- Garneau JE, Dupuis ME, Villion M, Romero DA, Barrangou R, Boyaval P, Fremaux C, Horvath P, Magadán AH, Moineau S. The CRISPR/Cas bacterial immune system cleaves bacteriophage and plasmid DNA. Nature. 2010; 468 :67–71. [ PubMed ] [ Google Scholar ]
- Gasiunas G, Barrangou R, Horvath P, Siksnys V. Cas9-crRNA ribonucleoprotein complex mediates specific DNA cleavage for adaptive immunity in bacteria. Proc Natl Acad Sci USA. 2012; 109 :E2579–E2586. [ PMC free article ] [ PubMed ] [ Google Scholar ]
- Gilbert LA, Larson MH, Morsut L, Liu Z, Brar GA, Torres SE, Stern-Ginossar N, Brandman O, Whitehead EH, Doudna JA, et al. CRISPR-mediated modular RNA-guided regulation of transcription in eukaryotes. Cell. 2013; 154 :442–451. [ PMC free article ] [ PubMed ] [ Google Scholar ]
- Gonzaelz B, Schwimmer LJ, Fuller RP, Ye Y, Asawapornmongkol L, Barbas CF. Modular system for the construction of zinc-finger libraries and proteins. Nat Protoc. 2010; 5 :791–810. [ PMC free article ] [ PubMed ] [ Google Scholar ]
- Haft DH, Selengut J, Mongodin EF, Nelson KE. A guild of 45 CRISPR-associated (Cas) protein families and multiple CRISPR/Cas subtypes exist in prokaryotic genomes. PLoS Comput Biol. 2005; 1 :e60. [ PMC free article ] [ PubMed ] [ Google Scholar ]
- Hale CR, Zhao P, Olson S, Duff MO, Graveley BR, Wells L, Terns RM, Terns MP. RNA-guided RNA cleavage by a CRISPR RNA-Cas protein complex. Cell. 2009; 139 :945–956. [ PMC free article ] [ PubMed ] [ Google Scholar ]
- Hale CR, Majumdar S, Elmore J, Pfister N, Compton M, Olson S, Resch AM, Glover CV, 3rd, Graveley BR, Terns RM, Terns MP. Essential features and rational design of CRISPR RNAs that function with the Cas RAMP module complex to cleave RNAs. Mol Cell. 2012; 45 :292–302. [ PMC free article ] [ PubMed ] [ Google Scholar ]
- Horvath P, Romero DA, Coûté -Monvoisin AC, Richards M, Deveau H, Moineau S, Boyaval P, Fremaux C, Barrangou R. Diversity, activity, and evolution of CRISPR loci in Streptococcus thermophilus. J Bacteriol. 2008; 190 :1401–1412. [ PMC free article ] [ PubMed ] [ Google Scholar ]
- Horvath P, Coûté -Monvoisin AC, Romero DA, Boyaval P, Fremaux C, Barrangou R. Comparative analysis of CRISPR loci in lactic acid bacteria genomes. Int J Food Microbiol. 2009; 131 :62–70. [ PubMed ] [ Google Scholar ]
- Hou Z, Zhang Y, Propson NE, Howden SE, Chu LF, Sontheimer EJ, Thomson JA. Efficient genome engineering in human pluripotent stem cells using Cas9 from Neisseria meningitidis. Proc Natl Acad Sci USA. 2013; 110 :15644–15649. [ PMC free article ] [ PubMed ] [ Google Scholar ]
- Hsu PD, Scott DA, Weinstein JA, Ran FA, Konermann S, Agarwala V, Li Y, Fine EJ, Wu X, Shalem O, et al. DNA targeting specificity of RNA-guided Cas9 nucleases. Nat Biotechnol. 2013; 31 :827–832. [ PMC free article ] [ PubMed ] [ Google Scholar ]
- Ishino Y, Shinagawa H, Makino K, Amemura M, Nakata A. Nucleotide sequence of the iap gene, responsible for alkaline phosphatase isozyme conversion in Escherichia coli, and identification of the gene product. J Bacteriol. 1987; 169 :5429–5433. [ PMC free article ] [ PubMed ] [ Google Scholar ]
- Jansen R, Embden JD, Gaastra W, Schouls LM. Identification of genes that are associated with DNA repeats in prokaryotes. Mol Microbiol. 2002; 43 :1565–1575. [ PubMed ] [ Google Scholar ]
- Jiang W, Bikard D, Cox D, Zhang F, Marraffini LA. RNA-guided editing of bacterial genomes using CRISPR-Cas systems. Nat Biotechnol. 2013; 31 :233–239. [ PMC free article ] [ PubMed ] [ Google Scholar ]
- Jinek M, Chylinski K, Fonfara I, Hauer M, Doudna JA, Charpentier E. A programmable dual-RNA-guided DNA endonuclease in adaptive bacterial immunity. Science. 2012; 337 :816–821. [ PMC free article ] [ PubMed ] [ Google Scholar ]
- Jinek M, East A, Cheng A, Lin S, Ma E, Doudna J. RNA-programmed genome editing in human cells. eLife. 2013; 2 :e00471. [ PMC free article ] [ PubMed ] [ Google Scholar ]
- Jinek M, Jiang F, Taylor DW, Sternberg SH, Kaya E, Ma E, Anders C, Hauer M, Zhou K, Lin S, et al. Structures of Cas9 endonucleases reveal RNA-mediated conformational activation. Science. 2014; 343 :1247997. [ PMC free article ] [ PubMed ] [ Google Scholar ]
- Juillerat A, Dubois G, Valton J, Thomas S, Stella S, Maréchal A, Langevin S, Benomari N, Bertonati C, Silva GH, et al. Comprehensive analysis of the specificity of transcription activator-like effector nucleases. Nucleic Acids Res. 2014; 42 :5390–5402. [ PMC free article ] [ PubMed ] [ Google Scholar ]
- Konermann S, Brigham MD, Trevino AE, Hsu PD, Heidenreich M, Cong L, Platt RJ, Scott DA, Church GM, Zhang F. Optical control of mammalian endogenous transcription and epigenetic states. Nature. 2013; 500 :472–476. [ PMC free article ] [ PubMed ] [ Google Scholar ]
- Li W, Teng F, Li T, Zhou Q. Simultaneous generation and germline transmission of multiple gene mutations in rat using CRISPR-Cas systems. Nat Biotechnol. 2013; 31 :684–686. [ PubMed ] [ Google Scholar ]
- Lombardo A, Genovese P, Beausejour CM, Colleoni S, Lee YL, Kim KA, Ando D, Urnov FD, Galli C, Gregory PD, et al. Gene editing in human stem cells using zinc finger nucleases and integrase-defective lentiviral vector delivery. Nat Biotechnol. 2007; 25 :1298–1306. [ PubMed ] [ Google Scholar ]
- Maeder ML, Thibodeau-Beganny S, Osiak A, Wright DA, Anthony RM, Eichtinger M, Jiang T, Foley JE, Winfrey RJ, Townsend JA, et al. Rapid “open-source” engineering of customized zinc-finger nucleases for highly efficient gene modification. Mol Cell. 2008; 31 :294–301. [ PMC free article ] [ PubMed ] [ Google Scholar ]
- Maeder ML, Angstman JF, Richardson ME, Linder SJ, Cascio VM, Tsai SQ, Ho QH, Sander JD, Reyon D, Bernstein BE, et al. Targeted DNA demethylation and activation of endogenous genes using programmable TALE-TET1 fusion proteins. Nat Biotechnol. 2013a; 31 :1137–1142. [ PMC free article ] [ PubMed ] [ Google Scholar ]
- Maeder ML, Linder SJ, Cascio VM, Fu Y, Ho QH, Joung JK. CRISPR RNA-guided activation of endogenous human genes. Nat Methods. 2013b; 10 :977–979. [ PMC free article ] [ PubMed ] [ Google Scholar ]
- Makarova KS, Grishin NV, Shabalina SA, Wolf YI, Koonin EV. A putative RNA-interference-based immune system in prokaryotes: computational analysis of the predicted enzymatic machinery, functional analogies with eukaryotic RNAi, and hypothetical mechanisms of action. Biol Direct. 2006; 1 :7. [ PMC free article ] [ PubMed ] [ Google Scholar ]
- Makarova KS, Aravind L, Wolf YI, Koonin EV. Unification of Cas protein families and a simple scenario for the origin and evolution of CRISPR-Cas systems. Biol Direct. 2011a; 6 :38. [ PMC free article ] [ PubMed ] [ Google Scholar ]
- Makarova KS, Haft DH, Barrangou R, Brouns SJ, Charpentier E, Horvath P, Moineau S, Mojica FJ, Wolf YI, Yakunin AF, et al. Evolution and classification of the CRISPR-Cas systems. Nat Rev Microbiol. 2011b; 9 :467–477. [ PMC free article ] [ PubMed ] [ Google Scholar ]
- Mali P, Yang L, Esvelt KM, Aach J, Guell M, DiCarlo JE, Norville JE, Church GM. RNA-guided human genome engineering via Cas9. Science. 2013a; 339 :823–826. [ PMC free article ] [ PubMed ] [ Google Scholar ]
- Mali P, Aach J, Stranges PB, Esvelt KM, Moosburner M, Kosuri S, Yang L, Church GM. CAS9 transcriptional activators for target specificity screening and paired nickases for cooperative genome engineering. Nat Biotechnol. 2013b; 31 :833–838. [ PMC free article ] [ PubMed ] [ Google Scholar ]
- Marraffini LA, Sontheimer EJ. CRISPR interference limits horizontal gene transfer in staphylococci by targeting DNA. Science. 2008; 322 :1843–1845. [ PMC free article ] [ PubMed ] [ Google Scholar ]
- Marraffini LA, Sontheimer EJ. Self versus non-self discrimination during CRISPR RNA-directed immunity. Nature. 2010; 463 :568–571. [ PMC free article ] [ PubMed ] [ Google Scholar ]
- Mendenhall EM, Williamson KE, Reyon D, Zou JY, Ram O, Joung JK, Bernstein BE. Locus-specific editing of histone modifications at endogenous enhancers. Nat Biotechnol. 2013; 31 :1133–1136. [ PMC free article ] [ PubMed ] [ Google Scholar ]
- Miller JC, Holmes MC, Wang J, Guschin DY, Lee YL, Rupniewski I, Beausejour CM, Waite AJ, Wang NS, Kim KA, et al. An improved zinc-finger nuclease architecture for highly specific genome editing. Nat Biotechnol. 2007; 25 :778–785. [ PubMed ] [ Google Scholar ]
- Miller JC, Tan S, Qiao G, Barlow KA, Wang J, Xia DF, Meng X, Paschon DE, Leung E, Hinkley SJ, et al. A TALE nuclease architecture for efficient genome editing. Nat Biotechnol. 2011; 29 :143–148. [ PubMed ] [ Google Scholar ]
- Mojica FJ, Díez-Villaseñor C, Soria E, Juez G. Biological significance of a family of regularly spaced repeats in the genomes of Archaea, Bacteria and mitochondria. Mol Microbiol. 2000; 36 :244–246. [ PubMed ] [ Google Scholar ]
- Mojica FJ, Díez-Villaseñor C, García-Martínez J, Soria E. Intervening sequences of regularly spaced prokaryotic repeats derive from foreign genetic elements. J Mol Evol. 2005; 60 :174–182. [ PubMed ] [ Google Scholar ]
- Moscou MJ, Bogdanove AJ. A simple cipher governs DNA recognition by TAL effectors. Science. 2009; 326 :1501. [ PubMed ] [ Google Scholar ]
- Musunuru K, Pirruccello JP, Do R, Peloso GM, Guiducci C, Sougnez C, Garimella KV, Fisher S, Abreu J, Barry AJ, et al. Exome sequencing, ANGPTL3 mutations, and familial combined hypolipidemia. N Engl J Med. 2010; 363 :2220–2227. [ PMC free article ] [ PubMed ] [ Google Scholar ]
- Niewoehner J, Bohrmann B, Collin L, Urich E, Sade H, Maier P, Rueger P, Stracke JO, Lau W, Tissot AC, et al. Increased brain penetration and potency of a therapeutic antibody using amonovalent molecular shuttle. Neuron. 2014; 81 :49–60. [ PubMed ] [ Google Scholar ]
- Nishimasu H, Ran FA, Hsu PD, Konermann S, Shehata SI, Dohmae N, Ishitani R, Zhang F, Nureki O. Crystal structure of Cas9 in complex with guide RNA and target DNA. Cell. 2014; 156 :935–949. [ PMC free article ] [ PubMed ] [ Google Scholar ]
- Niu Y, Shen B, Cui Y, Chen Y, Wang J, Wang L, Kang Y, Zhao X, Si W, Li W, et al. Generation of gene-modified cynomolgus monkey via Cas9/RNA-mediated gene targeting in one-cell embryos. Cell. 2014; 156 :836–843. [ PubMed ] [ Google Scholar ]
- Oh B, Hwang S, McLaughlin J, Solter D, Knowles BB. Timely translation during the mouse oocyte-to-embryo transition. Development. 2000; 127 :3795–3803. [ PubMed ] [ Google Scholar ]
- Pattanayak V, Lin S, Guilinger JP, Ma E, Doudna JA, Liu DR. High-throughput profiling of off-target DNA cleavage reveals RNA-programmed Cas9 nuclease specificity. Nat Biotechnol. 2013; 31 :839–843. [ PMC free article ] [ PubMed ] [ Google Scholar ]
- Perez-Pinera P, Kocak DD, Vockley CM, Adler AF, Kabadi AM, Polstein LR, Thakore PI, Glass KA, Ousterout DG, Leong KW, et al. RNA-guided gene activation by CRISPR-Cas9-based transcription factors. Nat Methods. 2013; 10 :973–976. [ PMC free article ] [ PubMed ] [ Google Scholar ]
- Plessis A, Perrin A, Haber JE, Dujon B. Site-specific recombination determined by I-SceI, a mitochondrial group I intron-encoded endonuclease expressed in the yeast nucleus. Genetics. 1992; 130 :451–460. [ PMC free article ] [ PubMed ] [ Google Scholar ]
- Pourcel C, Salvignol G, Vergnaud G. CRISPR elements in Yersinia pestis acquire new repeats by preferential uptake of bacteriophage DNA, and provide additional tools for evolutionary studies. Microbiology. 2005; 151 :653–663. [ PubMed ] [ Google Scholar ]
- Qi LS, Larson MH, Gilbert LA, Doudna JA, Weissman JS, Arkin AP, Lim WA. Repurposing CRISPR as an RNA-guided platform for sequence-specific control of gene expression. Cell. 2013; 152 :1173–1183. [ PMC free article ] [ PubMed ] [ Google Scholar ]
- Quiberoni A, Moineau S, Rousseau GM, Reinheimer J, Ackermann HW. Streptococcus thermophilus bacteriophages. Int Dairy J. 2010; 20 :657–664. [ Google Scholar ]
- Ran FA, Hsu PD, Lin CY, Gootenberg JS, Konermann S, Trevino AE, Scott DA, Inoue A, Matoba S, Zhang Y, Zhang F. Double nicking by RNA-guided CRISPR Cas9 for enhanced genome editing specificity. Cell. 2013; 154 :1380–1389. [ PMC free article ] [ PubMed ] [ Google Scholar ]
- Rouet P, Smih F, Jasin M. Introduction of double-strand breaks into the genome of mouse cells by expression of a rare-cutting endonuclease. Mol Cell Biol. 1994; 14 :8096–8106. [ PMC free article ] [ PubMed ] [ Google Scholar ]
- Rudin N, Sugarman E, Haber JE. Genetic and physical analysis of double-strand break repair and recombination in Saccharomyces cerevisiae. Genetics. 1989; 122 :519–534. [ PMC free article ] [ PubMed ] [ Google Scholar ]
- Sander JD, Joung JK. CRISPR-Cas systems for editing, regulating and targeting genomes. Nat Biotechnol. 2014; 32 :347–355. [ PMC free article ] [ PubMed ] [ Google Scholar ]
- Sander JD, Dahlborg EJ, Goodwin MJ, Cade L, Zhang F, Cifuentes D, Curtin SJ, Blackburn JS, Thibodeau-Beganny S, Qi Y, et al. Selection-free zinc-finger-nuclease engineering by context-dependent assembly (CoDA) Nat Methods. 2011; 8 :67–69. [ PMC free article ] [ PubMed ] [ Google Scholar ]
- Sapranauskas R, Gasiunas G, Fremaux C, Barrangou R, Horvath P, Siksnys V. The Streptococcus thermophilus CRISPR/Cas system provides immunity in Escherichia coli. Nucleic Acids Res. 2011; 39 :9275–9282. [ PMC free article ] [ PubMed ] [ Google Scholar ]
- Schwank G, Koo BK, Sasselli V, Dekkers JF, Heo I, Demircan T, Sasaki N, Boymans S, Cuppen E, van der Ent CK, et al. Functional repair of CFTR by CRISPR/Cas9 in intestinal stem cell organoids of cystic fibrosis patients. Cell Stem Cell. 2013; 13 :653–658. [ PubMed ] [ Google Scholar ]
- Shah SA, Erdmann S, Mojica FJ, Garrett RA. Protospacer recognition motifs: mixed identities and functional diversity. RNA Biol. 2013; 10 :891–899. [ PMC free article ] [ PubMed ] [ Google Scholar ]
- Shalem O, Sanjana NE, Hartenian E, Shi X, Scott DA, Mikkelsen TS, Heckl D, Ebert BL, Root DE, Doench JG, Zhang F. Genome-scale CRISPR-Cas9 knockout screening in human cells. Science. 2014; 343 :84–87. [ PMC free article ] [ PubMed ] [ Google Scholar ]
- Smith J, Grizot S, Arnould S, Duclert A, Epinat JC, Chames P, Prieto J, Redondo P, Blanco FJ, Bravo J, et al. A combinatorial approach to create artificial homing endonucleases cleaving chosen sequences. Nucleic Acids Res. 2006; 34 :e149. [ PMC free article ] [ PubMed ] [ Google Scholar ]
- Sternberg SH, Redding S, Jinek M, Greene EC, Doudna JA. DNA interrogation by the CRISPR RNA-guided endonuclease Cas9. Nature. 2014; 507 :62–67. [ PMC free article ] [ PubMed ] [ Google Scholar ]
- Tang TH, Bachellerie JP, Rozhdestvensky T, Bortolin ML, Huber H, Drungowski M, Elge T, Brosius J, Hüttenhofer A. Identification of 86 candidates for small non-messenger RNAs from the archaeon Archaeoglobus fulgidus. Proc Natl Acad Sci USA. 2002; 99 :7536–7541. [ PMC free article ] [ PubMed ] [ Google Scholar ]
- Tebas P, Stein D, Tang WW, Frank I, Wang SQ, Lee G, Spratt SK, Surosky RT, Giedlin MA, Nichol G, et al. Gene editing of CCR5 in autologous CD4 T cells of persons infected with HIV. N Engl J Med. 2014; 370 :901–910. [ PMC free article ] [ PubMed ] [ Google Scholar ]
- Urnov FD, Miller JC, Lee YL, Beausejour CM, Rock JM, Augustus S, Jamieson AC, Porteus MH, Gregory PD, Holmes MC. Highly efficient endogenous human gene correction using designed zinc-finger nucleases. Nature. 2005; 435 :646–651. [ PubMed ] [ Google Scholar ]
- Wang H, Yang H, Shivalila CS, Dawlaty MM, Cheng AW, Zhang F, Jaenisch R. One-step generation of mice carrying mutations in multiple genes by CRISPR/Cas-mediated genome engineering. Cell. 2013; 153 :910–918. [ PMC free article ] [ PubMed ] [ Google Scholar ]
- Wang T, Wei JJ, Sabatini DM, Lander ES. Genetic screens in human cells using the CRISPR-Cas9 system. Science. 2014; 343 :80–84. [ PMC free article ] [ PubMed ] [ Google Scholar ]
- Wu Y, Liang D, Wang Y, Bai M, Tang W, Bao S, Yan Z, Li D, Li J. Correction of a genetic disease in mouse via use of CRISPR-Cas9. Cell Stem Cell. 2013; 13 :659–662. [ PubMed ] [ Google Scholar ]
- Wu X, Scott DA, Kriz AJ, Chiu AC, Hsu PD, Dadon DB, Cheng AW, Trevino AE, Konermann S, Chen S, et al. Genome-wide binding of the CRISPR endonuclease Cas9 in mammalian cells. Nature Biotechnol. 2014 Published online Apr 20, 2014. http://dx.doi.org/10.1038/nbt.2889 . [ PMC free article ] [ PubMed ]
- Xu GL, Bestor TH. Cytosine methylation targetted to pre-determined sequences. Nat Genet. 1997; 17 :376–378. [ PubMed ] [ Google Scholar ]
- Yang H, Wang H, Shivalila CS, Cheng AW, Shi L, Jaenisch R. One-step generation of mice carrying reporter and conditional alleles by CRISPR/Cas-mediated genome engineering. Cell. 2013; 154 :1370–1379. [ PMC free article ] [ PubMed ] [ Google Scholar ]
- Yin H, Xue W, Chen S, Bogorad RL, Benedetti E, Grompe M, Koteliansky V, Sharp PA, Jacks T, Anderson DG. Genome editing with Cas9 in adult mice corrects a disease mutation and phenotype. Nature Biotechnol. 2014 Published online Mar 30, 2014. http://dx.doi.org/10.1038/nbt.2884 . [ PMC free article ] [ PubMed ]
- Zahradka K, Slade D, Bailone A, Sommer S, Averbeck D, Petranovic M, Lindner AB, Radman M. Reassembly of shattered chromosomes in Deinococcus radiodurans. Nature. 2006; 443 :569–573. [ PubMed ] [ Google Scholar ]
- Zhang Y, Heidrich N, Ampattu BJ, Gunderson CW, Seifert HS, Schoen C, Vogel J, Sontheimer EJ. Processing-independent CRISPR RNAs limit natural transformation in Neisseria meningitidis. Mol Cell. 2013; 50 :488–503. [ PMC free article ] [ PubMed ] [ Google Scholar ]
Ph.D. Program
Graduate studies.
The Genetics Ph.D. program provides opportunities for graduate study in all major areas of modern genetics, including identification and analysis of human disease genes, molecular evolution, gene therapy, statistical genetics, application of model organisms to problems in biology and medicine, and computational and experimental approaches to genome biology.
An underlying theme in our Department is that genetics is not merely a set of tools but a coherent and fruitful way of thinking about biology and medicine. To this end, we emphasize a spectrum of approaches based on molecules, organisms, populations, and genomes.
We provide training through laboratory rotations, dissertation research, seminar series, didactic and interactive coursework, and an annual three-day retreat.
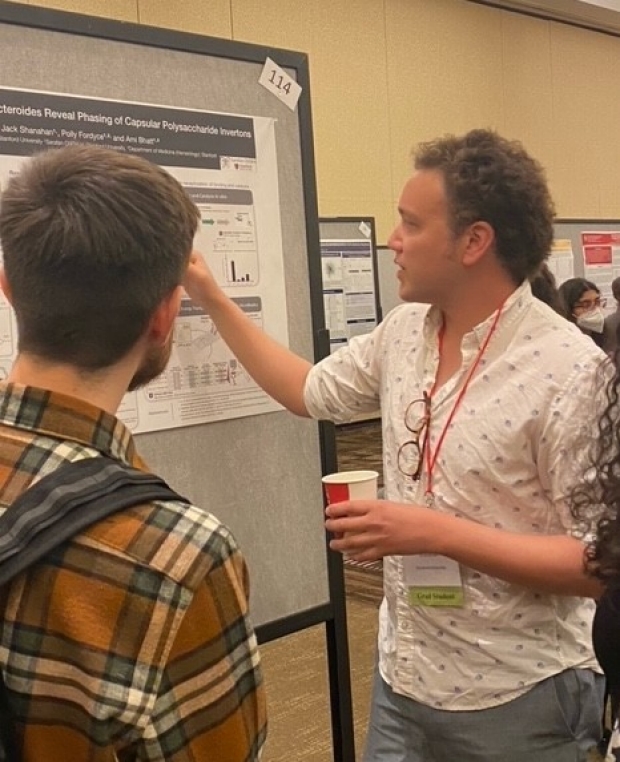
Students receive a competitive stipend ($51,600 for the 2023-24 Academic Year), tuition, health insurance, and a dental care stipend for a full four years. We also encourage students to seek additional fellowships, including but not limited to: NSF GRFP , NIH F31 , NDSEG , Stanford Bio-X fellowship , Stanford DARE , and Stanford CEHG Fellowship .
Lab Rotations
Students rotate through 3 laboratories during their first year in the Genetics Graduate Program. Rotations typically last one quarter each, but can be less and are contingent upon the faculty member agreeing to the rotation request. All Genetics students must rotate with at least 1 Genetics faculty member (primary or secondary appointment). Other rotations may be done with any Bioscience faculty.
While most students start in Fall Quarter, students are encouraged to consider participating in the Advance Summer Institute for a smoother early transition into graduate school. There is a nomination & selection process. The department nominates, so if you are interested please let the department student services officer know. The program is not meant to be a source of summer bridge funding or simply an early rotation opportunity. There are many components to the program that require commitment of time and effort; and the funding, reflects both the expectation of full participation and belief that participants should be compensated for these efforts. The Office of Graduate Education does the selection for ADVANCE. There is no guarantee that if you are nominated that you will be admitted into ADVANCE.
While students may select a thesis laboratory after completing their third rotation, you can do more. Selection of the dissertation research laboratory must be done with the faculty member's approval. Prior to committing to a dissertation laboratory, students are invited to discuss their selection with the Graduate Program Director. Students are welcome to join labs outside of the Genetics Department; if so, they will discuss with the Graduate Program Director whether transferring into that department would be beneficial.
Dissertation Research
Once a student selects a permanent laboratory, they begin their dissertation research that will last for approximately four years. All students are expected to publish at least one first-author paper about their research during this time period, and the work culminates with a thesis defense presentation and written dissertation. See the Genetics Student Handbook for more information.
Coursework, Qualifying Exams, and other Requirements
Students in the Genetics Graduate Program take the Qualifying Examination in the Fall Quarter of their second year of study. There are two parts to the exam, a written research proposal and an oral examination.

Ami Bhatt, Dylan Maghini, and collaborators from the University of the Witwatersrand visit with researchers and staff at the MRC/Wits Public Health and Health Transitions Research Unit in Agincourt, South Africa.

Ami Bhatt, Dylan Maghini, and collaborators tour the MRC/Wits Public Health and Health Transitions Research Unit labs and biobank facility in Agincourt, South Africa.
Service Requirement
Service and outreach are a critical component of a student’s development as a scientist, and offer unique opportunities to learn by interacting with individuals outside the Department. Students are expected to participate in a minimum of 60 hours of service and/or outreach work prior to defending their dissertation.
Supplementary Educational Activities
In addition to your courses, qualifying exams, and dissertation, the Genetics Department has arranged additional educational activities for students. These regularly occurring meetings are:
Current Issues in Genetics (CIG) Two people from the Genetics Department give 20-25 minute presentations about their current work at this weekly Friday meeting. Students in their third year and above are expected to present their work annually. This series gives students the chance to learn about the range of science going on in the department and provides a great opportunity to give formal presentations to peers and colleagues.
Graduate Student Journal Club This weekly journal club is organized completely by graduate students from the Genetics and Developmental Biology Departments. At each meeting, one or two graduate students lead 30 minute discussions on their choice of a recent journal article. For the first three years of the Ph.D. program, each student presents once per academic year.
Refreshments are provided by the graduate students and reimbursed up to the current year limit. Reimbursement requires an original receipt to the Student Services Coordinator.
Frontiers in Biology Every week, the Departments of Genetics, Developmental Biology, and Biochemistry host an external speaker through the “Frontiers in Biology” seminar series. First year students also take a course related to this seminar (GENE 215), where they discuss a relevant paper the day before and meet the speaker after the presentation.
Frontiers is held most Wednesdays at 4pm in Clark Auditorium.
Other Seminar Series There are many other regular seminar series on campus that students choose to attend. Some of the most popular include:
Center of Law and the Biosciences lunchtime talks. See CLB events calendar or subscribe to the listserv
Evolgenome (organized by CEHG). See CEHG website or subscribe to the listserv
Teaching and Mentoring Academy Events. See TMA website or subscribe to the listserv
Discussing Developmental Data (3D). See events schedule
Biomedical Seminars. See events schedule or subscribe to the listserv
Requirements
Important forms.
Service Requirement Form
Email Wendy Christiansen
- Write my thesis
- Thesis writers
- Buy thesis papers
- Bachelor thesis
- Master's thesis
- Thesis editing services
- Thesis proofreading services
- Buy a thesis online
- Write my dissertation
- Dissertation proposal help
- Pay for dissertation
- Custom dissertation
- Dissertation help online
- Buy dissertation online
- Cheap dissertation
- Dissertation editing services
- Write my research paper
- Buy research paper online
- Pay for research paper
- Research paper help
- Order research paper
- Custom research paper
- Cheap research paper
- Research papers for sale
- Thesis subjects
- How It Works
122 The Best Genetics Research Topics For Projects
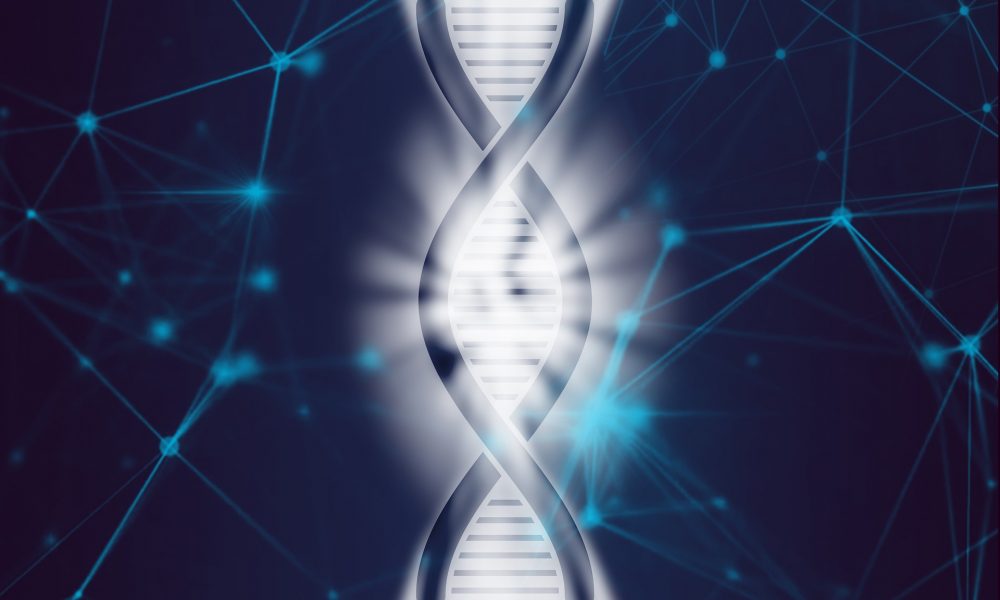
The study of genetics takes place across different levels of the education system in academic facilities all around the world. It is an academic discipline that seeks to explain the mechanism of heredity and genes in living organisms. First discovered back in the 1850s, the study of genetics has come a pretty long way, and it plays such an immense role in our everyday lives. Therefore, when you are assigned a genetics research paper, you should pick a topic that is not only interesting to you but one that you understand well.
Choosing Research Topics in Genetics
Even for the most knowledgeable person in the room, choosing a genetics topic for research papers can be, at times, a hectic experience. So we put together a list of some of the most exciting top in genetics to make the endeavor easier for you. However, note, while all the topics we’ve listed below will enable you to write a unique genetic project, remember what you choose can make or break your paper. So again, select a topic that you are both interested and knowledgeable on, and that has plenty of research materials to use. Without further ado, check out the topics below.
Interesting Genetics Topics for your Next Research Paper
- Genes and DNA: write a beginners’ guide to genetics and its applications
- Factors that contribute or/and cause genetic mutations
- Genetics and obesity, what do you need to know?
- Describe RNA information
- Is there a possibility of the genetic code being confidential?
- Are there any living cells present in the gene?
- Cancer and genetics
- Describe the role of genetics in the fight against Alzheimer’s disease
- What is the gene
- Is there a link between genetics and Parkinson’s disease? Explain your answer.
- Replacement of genes and artificial chromosomes
- Explain genetic grounds for obesity
- Development and disease; how can genetics dissect the developing process
- Analyzing gene expression – RNA
- Gene interaction; eye development
- Advances and developments in nanotechnology to enable therapeutic methods for the treatment of HIV and AIDS.
- Isolating and identifying the cancer treatment activity of special organic metal compounds.
- Analyzing the characteristics in certain human genes that can withstand heavy metals.
- A detailed analysis of genotypes that is both sensitive and able to endure heavy metals.
- Isolating special growth-inducing bacteria that can assist crops during heavy metal damage and identifying lipid directing molecules for escalating heavy metal endurance in plants.
Hot and Controversial Topics in Genetics
- Is there a link between genetics and homosexuality? Explain your answer
- Is it ethical and morally upright to grow human organs
- Can DNA changes beat aging
- The history and development of human cloning science
- How addictive substances alter our genes
- Are genetically modified foods safe for human and animal consumption?
- Is depression a genetically based condition?
- Genetic diagnosis of the fetus
- Genetic analysis of the DNA structure
- What impact does cloning have on future generations?
- What is the link between genetics and autism?
- Can artificial insemination have any sort of genetic impact on a person?
- The advancements in genetic research and the bioethics that come with them.
- Is human organ farming a possibility today?
- Can genetics allow us to design and build a human to our specifications?
- Is it ethical to try and tamper with human genetics in any way?
Molecular Genetics Topics
- Molecular techniques: How to analyze DNA(including genomes), RNA as well as proteins
- Stem cells describe their potential and shortcomings
- Describe molecular and genome evolution
- Describe DNA as the agent of heredity
- Explain the power of targeted mutagenesis
- Bacteria as a genetic system
- Explain how genetic factors increase cancer susceptibility
- Outline and describe recent advances in molecular cancer genetics
- Does our DNA sequencing have space for more?
- Terminal illness and DNA.
- Does our DNA determine our body structure?
- What more can we possibly discover about DNA?
Genetic Engineering Topics
- Define gene editing, and outline key gene-editing technologies, explaining their impact on genetic engineering
- The essential role the human microbiome plays in preventing diseases
- The principles of genetic engineering
- Project on different types of cloning
- What is whole genome sequencing
- Explain existing studies on DNA-modified organisms
- How cloning can impact medicine
- Does our genetics hold the key to disease prevention?
- Can our genetics make us resistant to certain bacteria and viruses?
- Why our genetics plays a role in chronic degenerative diseases.
- Is it possible to create an organism in a controlled environment with genetic engineering?
- Would cloning lead to new advancements in genetic research?
- Is there a possibility to enhance human DNA?
- Why do we share DNA with so many other animals on the planet?
- Is our DNA still evolving or have reached our biological limit?
- Can human DNA be manipulated on a molecular or atomic level?
- Do we know everything there is to know about our DNA, or is there more?
Controversial Human Genetic Topics
- Who owns the rights to the human genome
- Is it legal for parents to order genetically perfect children
- is genetic testing necessary
- What is your stand on artificial insemination vs. ordinary pregnancy
- Do biotech companies have the right to patent human genes
- Define the scope of the accuracy of genetic testing
- Perks of human genetic engineering
- Write about gene replacement and its relationship to artificial chromosomes.
- Analyzing DNA and cloning
- DNA isolation and nanotechnology methods to achieve it.
- Genotyping of African citizens.
- Greatly mutating Y-STRs and the isolated study of their genetic variation.
- The analytical finding of indels and their genetic diversity.
DNA Research Paper Topics
The role and research of DNA are so impactful today that it has a significant effect on our daily lives today. From health care to medication and ethics, over the last few decades, our knowledge of DNA has experienced a lot of growth. A lot has been discovered from the research of DNA and genetics.
Therefore, writing a good research paper on DNA is quite the task today. Choosing the right topic can make things a lot easier and interesting for writing your paper. Also, make sure that you have reliable resources before you begin with your paper.
- Can we possibly identify and extract dinosaur DNA?
- Is the possibility of cloning just around the corner?
- Is there a connection between the way we behave and our genetic sequence?
- DNA research and the environment we live in.
- Does our DNA sequencing have something to do with our allergies?
- The connection between hereditary diseases and our DNA.
- The new perspectives and complications that DNA can give us.
- Is DNA the reason all don’t have similar looks?
- How complex human DNA is.
- Is there any sort of connection between our DNA and cancer susceptibility and resistance?
- What components of our DNA affect our decision-making and personality?
- Is it possible to create DNA from scratch under the right conditions?
- Why is carbon such a big factor in DNA composition?
- Why is RNA something to consider in viral research and its impact on human DNA?
- Can we detect defects in a person’s DNA before they are born?
Genetics Topics For Presentation
The subject of genetics can be quite broad and complex. However, choosing a topic that you are familiar with and is unique can be beneficial to your presentation. Genetics plays an important part in biology and has an effect on everyone, from our personal lives to our professional careers.
Below are some topics you can use to set up a great genetics presentation. It helps to pick a topic that you find engaging and have a good understanding of. This helps by making your presentation clear and concise.
- Can we create an artificial gene that’s made up of synthetic chromosomes?
- Is cloning the next step in genetic research and engineering?
- The complexity and significance of genetic mutation.
- The unlimited potential and advantages of human genetics.
- What can the analysis of an individual’s DNA tell us about their genetics?
- Is it necessary to conduct any form of genetic testing?
- Is it ethical to possibly own a patent to patent genes?
- How accurate are the results of a genetics test?
- Can hereditary conditions be isolated and eliminated with genetic research?
- Can genetically modified food have an impact on our genetics?
- Can genetics have a role to play in an individual’s sexuality?
- The advantages of further genetic research.
- The pros and cons of genetic engineering.
- The genetic impact of terminal and neurological diseases.
Biotechnology Topics For Research Papers
As we all know, the combination of biology and technology is a great subject. Biotechnology still offers many opportunities for eager minds to make innovations. Biotechnology has a significant role in the development of modern technology.
Below you can find some interesting topics to use in your next biotechnology research paper. Make sure that your sources are reliable and engage both you and the reader.
- Settlements that promote sustainable energy technology maintenance.
- Producing ethanol through molasses emission treatment.
- Evapotranspiration and its different processes.
- Circular biotechnology and its widespread framework.
- Understanding the genes responsible for flora response to harsh conditions.
- Molecule signaling in plants responding to dehydration and increased sodium.
- The genetic improvement of plant capabilities in major crop yielding.
- Pharmacogenomics on cancer treatment medication.
- Pharmacogenomics on hypertension treating medication.
- The uses of nanotechnology in genotyping.
- How we can quickly detect and identify food-connected pathogens using molecular-based technology.
- The impact of processing technology both new and traditional on bacteria cultures linked to Aspalathus linearis.
- A detailed analysis of adequate and renewable sorghum sources for bioethanol manufacturing in South Africa.
- A detailed analysis of cancer treatment agents represented as special quinone compounds.
- Understanding the targeted administering of embelin to cancerous cells.
Tips for Writing an Interesting Genetics Research Paper
All the genetics research topics above are excellent, and if utilized well, could help you come up with a killer research paper. However, a good genetics research paper goes beyond the topic. Therefore, besides choosing a topic, you are most interested in, and one with sufficient research materials ensure you
Fully Understand the Research Paper Format
You may write on the most interesting genetics topics and have a well-thought-out set of ideas, but if your work is not arranged in an engaging and readable manner, your professor is likely to dismiss it, without looking at what you’ve written. That is the last thing you need as a person seeking to score excellent grades. Therefore, before you even put pen to paper, understand what research format is required.
Keep in mind that part of understanding the paper’s format is knowing what words to use and not to use. You can contact our trustful masters to get qualified assistance.
Research Thoroughly and Create an Outline
Whichever genetics research paper topics you decide to go with, the key to having excellent results is appropriately researching it. Therefore, embark on a journey to understand your genetics research paper topic by thoroughly studying it using resources from your school’s library and the internet.
Ensure you create an outline so that you can note all the useful genetic project ideas down. A research paper outline will help ensure that you don’t forget even one important point. It also enables you to organize your thoughts. That way, writing them down in the actual genetics research paper becomes smooth sailing. In other words, a genetics project outline is more like a sketch of the paper.
Other than the outline, it pays to have an excellent research strategy. In other words, instead of looking for information on any random source you come across, it would be wise to have a step-by-step process of looking for the research information.
For instance, you could start by reading your notes to see what they have to say about the topic you’ve chosen. Next, visit your school’s library, go through any books related to your genetics research paper topic to see whether the information on your notes is correct and for additional information on the topic. Note, you can visit the library either physically or via your school’s website. Lastly, browse educational sites such as Google Scholar, for additional information. This way, you’ll start your work with a bunch of excellent genetics project ideas, and at the same time, you’ll have enjoyed every step of the research process.
Get Down to Work
Now turn the genetics project ideas on your outline into a genetics research paper full of useful and factual information.
There is no denying writing a genetics research paper is one of the hardest parts of your studies. But with the above genetics topics and writing tips to guide you, it should be a tad easier. Good luck!
Leave a Reply Cancel reply
132 Genetic Engineering Essay Topic Ideas & Examples
Welcome to our list of genetic engineering essay topics! Here, you will find everything from trending research titles to the most interesting genetic engineering topics for presentation. Get inspired with our writing ideas and bonus samples!
🔝 Top 10 Genetic Engineering Topics for 2024
🏆 best genetic engineering topic ideas & essay examples, ⭐ good genetic engineering research topics, 👍 simple & easy genetic engineering essay topics, ❓ genetic engineering discussion questions, 🔎 genetic engineering research topics, ✅ genetic engineering project ideas.
- Ethical Issues of Synthetic Biology
- CRISPR-Cas9 and Its Applications
- Progress and Challenges in Gene Therapy
- Applications of Gene Editing in Animals
- The Process of Genetic Engineering in Plants
- Genetic Engineering for Human Enhancement
- Genetic Engineering for Improving Crop Yield
- Regulatory Issues of Genetic Editing of Embryos
- Gene Silencing in Humans through RNA Interference
- Gene Drive Technology for Controlling Invasive Species
- Future of Genetic Engineering and the Concept of “Franken-Foods” This is not limited to cows alone but extends to pigs, sheep, and poultry, the justification for the development of genetically modified food is based on the need to feed an ever growing population which […]
- The Ethical Issues of Genetic Engineering Many people have questioned the health risks that arise from genetically modified crops, thus it is the politicians who have to ensure that the interests of the people are met and their safety is assured. […]
- Gattaca: Ethical Issues of Genetic Engineering Although the world he lives in has determined that the only measure of a man is his genetic profile, Vincent discovers another element of man that science and society have forgotten.
- The Film “Gattaca” and Genetic Engineering In the film, it is convincing that in the near future, science and technology at the back of genetic engineering shall be developed up to the level which makes the film a reality.
- The Dangers of Genetic Engineering and the Issue of Human Genes’ Modification In this case, the ethics of human cloning and human genes’ alteration are at the center of the most heated debates. The first reason to oppose the idea of manipulation of human genes lies in […]
- Changing the world: Genetic Engineering Effects Genes used in genetic engineering have a high impact on health and disease, therefore the inclusion of the genetic process alters the genes that influence human behavior and traits.
- Human Genetic Engineering: Key Principles and Issues There are many options for the development of events in the field of genetic engineering, and not all of them have been studied. To conclude, human genetic engineering is one of the major medical breakthroughs, […]
- Mitochondrial Diseases Treatment Through Genetic Engineering Any disorders and abnormalities in the development of mitochondrial genetic information can lead to the dysfunction of these organelles, which in turn affects the efficiency of intracellular ATP production during the process of cellular respiration.
- Genetic Engineering: Is It Ethical to Manipulate Life? In the case of more complex operations, genetic engineering can edit existing genes to turn on or off the synthesis of a particular protein in the organism from which the gene was taken.
- Biotechnology and Genetic Engineering Apart from that, there are some experiments that cannot be ethically justified, at least in my opinion, for example, the cloning of human being or the attempts to find the gene for genius.
- Genetic Engineering in the Movie “Gattaca” by Niccol This would not be right at all since a person should be responsible for their own life and not have it dictated to them as a result of a societal construct created on the basis […]
- Religious vs Scientific Views on Genetic Engineering With the need to increase the global economy, the field of agriculture is one among the many that have been used to improve the commercial production to take care of the global needs for food […]
- Genetic Engineering Using a Pglo Plasmid The objective of this experiment is to understand the process and importance of the genetic transformation of bacteria in real time with the aid of extrachromosomal DNA, alternatively referred to as plasmids.
- Managing Diabetes Through Genetic Engineering Genetic engineering refers to the alteration of genetic make-up of an organism through the use of techniques to introduce a new DNA or eliminate a given hereditable material. What is the role of genetic engineering […]
- The Role of Plant Genetic Engineering in Global Security Although it can be conveniently stated that the adequacy, abundance and reliability of the global food supply has a major role to play in the enhancement of human life, in the long run, they influence […]
- Significance of Human Genetic Engineering The gene alteration strategy enables replacing the specific unwanted genes with the new ones, which are more resistant and freer of the particular ailment, hence an essential assurance of a healthy generation in the future.
- Is the World Ready for Genetic Engineering? The process of manipulating genes has brought scientists to important discoveries, among which is the technology of the production of new kinds of crops and plants with selected characteristics. The problem of the advantages and […]
- Genome: Bioethics and Genetic Engineering Additionally, towards the end of the documentary, the narrator and some of the interviewed individuals explain the problem of anonymity that is also related to genetic manipulations.
- Is Genetic Engineering an Environmentally Sound Way to Increase Food Production? According to Thomas & Earl and Barry, genetic engineering is environmentally unsound method of increasing food production because it threatens the indigenous species.
- A Major Milestone in the Field of Science and Technology: Should Genetic Engineering Be Allowed? The most controversial and complicated aspect of this expertise is Human Genetic Engineering- whereby the genotype of a fetus can be altered to produce desired results.
- Genetic Engineering Is Ethically Unacceptable However, the current application of genetic engineering is in the field of medicine particularly to treat various genetic conditions. However, this method of treatment has various consequences to the individual and the society in general.
- Designer Genes: Different Types and Use of Genetic Engineering McKibben speaks of Somatic Gene Therapy as it is used to modify the gene and cell structure of human beings so that the cells are able to produce certain chemicals that would help the body […]
- A Technique for Controlling Plant Characteristics: Genetic Engineering in the Agriculture A cautious investigation of genetic engineering is required to make sure it is safe for humans and the environment. The benefit credited to genetic manipulation is influenced through the utilization of herbicide-tolerant and pest-safe traits.
- Genetically Engineered Food Against World Hunger I support the production of GMFs in large quality; I hold the opinion that they can offer a lasting solution to food problems facing the world.
- Genetic Engineering in Food: Development and Risks Genetic engineering refers to the manipulation of the gene composition of organisms, to come up with organisms, which have different characteristics from the organic ones.
- Genetic Engineering in the Workplace The main purpose of the paper is to evaluate and critically discuss the ethical concerns regarding the implementation of genetic testing in the workplace and to provide potential resolutions to the dilemmas.
- Designer Babies Creation in Genetic Engineering The creation of designer babies is an outcome of advancements in technology hence the debate should be on the extent to which technology can be applied in changing the way human beings live and the […]
- Genetic Engineering and Eugenics Comparison The main idea in genetic engineering is to manipulate the genetic make-up of human beings in order to shackle their inferior traits. The concept of socially independent reproduction is replicated in both eugenics and genetic […]
- Ecological Effects of the Release of Genetically Engineered Organisms Beneficial soil organisms such as earthworms, mites, nematodes, woodlice among others are some of the soil living organisms that are adversely affected by introduction of genetically engineered organisms in the ecosystem since they introduce toxins […]
- Proposition 37 and Genetically Engineered Foods The discussion of Proposition 37 by the public is based on the obvious gap between the “law on the books” and the “law in action” because Food Safety Law which is associated with the Proposition […]
- Is Genetically Engineered Food the Solution to the World’s Hunger Problems? However, the acceptance of GMO’s as the solution to the world’s food problem is not unanimously and there is still a multitude of opposition and suspicion of their use.
- Benefits of Genetic Engineering as a Huge Part of People’s Lives Genetic Engineering is said to question whether man has the right to manipulate the course and laws of nature and thus is in constant collision with religion and the beliefs held by it regarding life.
- Perfect Society: The Effects of Human Genetic Engineering
- Genetic Engineering and Forensic Criminal Investigations
- Biotechnology Assignment and Genetic Engineering
- Genetic Engineering and Genetically Modified Organisms
- Bio-Ethics and the Controversy of Genetic Engineering
- Health and Environmental Risks of Genetic Engineering in Food
- Genetic Engineering and the Risks of Enforcing Changes on Organisms
- Genetic Engineering and How It Affects Globel Warming
- Cloning and Genetic Engineering in the Food Animal Industry
- Genetic Engineering and Its Impact on Society
- Embryonic Research, Genetic Engineering, & Cloning
- Genetic Engineering: Associated Risks and Possibilities
- Issues Concerning Genetic Engineering in Food Production
- Genetic Engineering, DNA Fingerprinting, Gene Therapy
- Cloning: The Benefits and Dangers of Genetic Engineering
- Genetic Engineering, History, and Future: Altering the Face of Science
- Islamic and Catholic Views on Genetic Engineering
- Gene Therapy and Genetic Engineering: Should It Be Approved in the US
- Exploring the Real Benefits of Genetic Engineering in the Modern World
- Genetic Engineering and Food Security: A Welfare Economics Perspective
- Identify the Potential Impact of Genetic Engineering on the Future Course of Human Immunodeficiency Virus
- Genetic Engineering and DNA Technology in Agricultural Productivity
- Human Genetic Engineering: Designing the Future
- Genetic Engineering and the Politics Behind It
- The Potential and Consequences of Genetic Engineering
- Genetic Engineering and Its Effect on Human Health
- The Moral and Ethical Controversies, Benefits, and Future of Genetic Engineering
- Gene Therapy and Genetic Engineering for Curing Disorders
- Genetic Engineering and the Human Genome Project
- Ethical Standards for Genetic Engineering
- Genetic Engineering and Cryonic Freezing: A Modern Frankenstein
- The Perfect Child: Genetic Engineering
- Genetic Engineering and Its Effects on Future Generations
- Agricultural Genetic Engineering: Genetically Modified Foods
- Genetic Engineering: The Manipulation or Alteration of the Genetic Structure of a Single Cell or Organism
- Analysing Genetic Engineering Regarding Plato Philosophy
- The Dangers and Benefits of Human Cloning and Genetic Engineering
- Genetic Engineering: Arguments of Both Proponents and Opponents and a Mediated Solution
- Genetic and How Genetic Engineering Is Diffusing Individualism
- Finding Genetic Harmony With Genetic Engineering
- What Is Genetic Engineering?
- Do You Think Genetically Modified Food Could Harm the Ecosystems of the Areas in Which They Grow?
- How Agricultural Research Systems Shape a Technological Regime That Develops Genetic Engineering?
- Can Genetic Engineering for the Poor Pay Off?
- How Does Genetic Engineering Affect Agriculture?
- Do You Think It’s Essential to Modify Genes to Create New Medicines?
- How Can Genetic Engineering Stop Human Suffering?
- Can Genetic Engineering Cure HIV/AIDS in Humans?
- How Has Genetic Engineering Revolutionized Science and the World?
- Do You Think Genetic Engineering Is Playing God and That We Should Leave Life as It Was Created?
- What Are Some Advantages and Disadvantages of Genetic Engineering?
- How Will Genetic Engineering Affect the Human Race?
- When Does Genetic Engineering Go Bad?
- What Are the Benefits of Human Genetic Engineering?
- Does Genetic Engineering Affect the Entire World?
- How Does the Christian Faith Contend With Genetic Engineering?
- What Are the Ethical and Social Implications of Genetic Engineering?
- How Will Genetic Engineering Impact Our Lives?
- Why Should Genetic Engineering Be Extended?
- Will Genetic Engineering Permanently Change Our Society?
- What Are People Worried About Who Oppose Genetic Engineering?
- Do You Worry About Eating GM (Genetically Modified) Food?
- What Do You Think of the Idea of Genetically Engineering New Bodily Organs to Replace Yours When You Are Old?
- Should Genetic Engineering Go Ahead to Eliminate Human Flaws, Such as Violence, Jealousy, Hate, Etc?
- Does the Government Have the Right to Limit How Far We Modify Ourselves?
- Why Is Genetic Food Not Well Accepted?
- What Is the Best in the Genetic Modification of Plants, Plant Cell, or Chloroplasts and Why?
- How Do You Feel About Human Gene Editing?
- Does Climate Change Make the Genetic Engineering of Crops Inevitable?
- What Do You Think About Plant Genetic Modification?
- Gene Drives and Pest Control
- The Benefits of Genetically Modified Organisms
- Challenges of Gene Editing for Rare Genetic Diseases
- The Use of Genetic Engineering to Treat Human Diseases
- Ethical Considerations and Possibilities of Designer Babies
- How Genetic Engineering Can Help Restore Ecosystems
- Basic Techniques and Tools for Gene Manipulation
- Latest Advancements in Genetic Engineering and Genome Editing
- Will Engineering Resilient Organisms Help Mitigate Climate Change?
- Creation of Renewable Resources through Genetic Engineering
- Genetic Engineering Approach to Drought and Pest Resistance
- Genetic Engineering Use in DNA Analysis and Identification
- Synthetic Microorganisms and Biofactories for Sustainable Bioproduction
- Stem Cells’ Potential for Regenerative Medicine
- The Role of Genetic Modification in Vaccine Development
- Can Genetic Engineering Help Eradicate Invasive Species Responsibly?
- Genetic Engineering for Enhancing the Body’s Defense Mechanisms
- Advancements in Transplantation Medicine and Creating Bioengineered Organs
- Genetic Editing of Microbes for Environmental Cleanup
- Is It Possible to Develop Living Detection Systems?
- Infertility Essay Topics
- Bioethics Titles
- Genetics Research Ideas
- Epigenetics Essay Titles
- Morality Research Ideas
- Stem Cell Essay Titles
- Biochemistry Research Topics
- Evolution Topics
- Chicago (A-D)
- Chicago (N-B)
IvyPanda. (2024, February 26). 132 Genetic Engineering Essay Topic Ideas & Examples. https://ivypanda.com/essays/topic/genetic-engineering-essay-topics/
"132 Genetic Engineering Essay Topic Ideas & Examples." IvyPanda , 26 Feb. 2024, ivypanda.com/essays/topic/genetic-engineering-essay-topics/.
IvyPanda . (2024) '132 Genetic Engineering Essay Topic Ideas & Examples'. 26 February.
IvyPanda . 2024. "132 Genetic Engineering Essay Topic Ideas & Examples." February 26, 2024. https://ivypanda.com/essays/topic/genetic-engineering-essay-topics/.
1. IvyPanda . "132 Genetic Engineering Essay Topic Ideas & Examples." February 26, 2024. https://ivypanda.com/essays/topic/genetic-engineering-essay-topics/.
Bibliography
IvyPanda . "132 Genetic Engineering Essay Topic Ideas & Examples." February 26, 2024. https://ivypanda.com/essays/topic/genetic-engineering-essay-topics/.

COMMENTS
Genetic engineer ing is the direct modification of an. org anism' s genome, which is the list of specific traits. (genes) s tored in the DNA. Changing the genome. enables engineer s to giv e ...
In The Case Against Perfection, political philosopher Michael Sandel, presents a moral explanation for this unease…. He makes the compelling case that genetic engineering to gain advantage for ourselves and our children is deeply disempowering, because it turns us away from the communal good, toward self-centered striving." —Anne Harding ...
Genetic Engineering: A Look into Perfection and a Definitive Line Joshua henstrom I n the wake of CRISPR's birth in 1987, widespread fear and excitement ... we can modestly extend Rakić's thesis by showing that genetic moral enhancement is not merely permissible, but a moral obligation. The paper will go as follows. In Part I, I will ...
Here, we describe principles of genetic engineering and detail: (1) how common elements of current technologies include the need for. chromosome break to occur, (2) the use of specific and sensitive genotyping assays to detect altered genomes, and (3) delivery modalities that impact characterization of gene modifications.
However, genetic engineering has been, so far, the most controversial application of technology to agriculture. Together with its potentialities rose a series of ethical concerns that can be summarized in the following three main categories; the third of which will be the focus of the present thesis. Firstly, food safety. Due to the fact that ...
30 years of progress from positional cloning to precision genome editing. Thirty years ago, I had the privilege of launching Nature Genetics, the first spin-off journal bearing the famous Nature ...
Genetic engineering is the act of modifying the genetic makeup of an organism. Modifications can be generated by methods such as gene targeting, nuclear transplantation, transfection of synthetic ...
genetic engineering, and the use of genetic engineering itself. Specifically, I am looking at the way that the concept of genetic engineering shapes a text in certain, theoretically significant ways. Genetic engineering, as it is used in the texts I study in this thesis, operates as a
genetic engineering. The motivation for focusing this thesis on cases of genetic engineering is the development of a "gene-editing technique called CRISPR-cas9"7 that is both promising to be able to edit different characteristics of DNA whilst being cheaper and more easily accessible than previous techniques.
dspace.mit.edu
When designing novel genetic tools, it is imperative to consider the final target community and their preferences. While the initial progress in plasmid engineering was built upon the use of restriction enzymes and DNA ligase, restriction‐free methods are becoming more and more popular in the synthetic biology community (Ellis et al., 2011).
3 A gene drive is a genetic engineering technology—adding, deleting, disrupting, or modifying genes—to rapidly spread a particular genetic trait to an entire offspring population. A gene drive ...
Genetic engineering opens new possibilities for biomedical enhancement requiring ethical, societal and practical considerations to evaluate its implications for human biology, human evolution and our natural environment. In this Commentary, we consider human enhancement, and in particular, we explore genetic enhancement in an evolutionary ...
Artificial manipulation of genes is a relatively new science, and a number of watershed moments have provided the foundation for the current state of genetic engineering. Researchers first discovered that nonspecific alterations to Drosophila DNA could be introduced using radiation 1 and chemicals 2 in 1927 and 1947, respectively.
Aside from the safety risks, human genome editing poses some hefty ethical questions. For families who have watched their children suffer from devastating genetic diseases, the technology offers the hope of editing cruel mutations out of the gene pool. For those living in poverty, it is yet another way for the privileged to vault ahead.
Genetic engineering of the chloroplast genome has been well established for another microalga Chlamydomonas reinhardtii. This system was exploited in three biotechnological applications: 1) generation of alkane producing strains by introducing genes encoding for acyl reductase and aldehyde decarbonylase.
Rice genetic engineering permits access to an unlimited gene pool through the transfer of desirable genes between any two species of interest, irrespective of their evolutionary or taxonomic relationships. However, there are major problems to be considered for a successful foreign gene introgression into rice. Two of the major problems were considered in this thesis: first is the development ...
If you're just starting out exploring biotechnology-related topics for your dissertation, thesis or research project, you've come to the right place. ... Genetic Engineering Technologies for Improving Crop Yield and Quality (Ye et al., 2022) Legal Aspects of Genetically Modified Food Product Safety for Health in Indonesia (Khamdi, 2022)
Additionally, precise genetic engineering of important agricultural crops could confer resistance to environmental deprivation or pathogenic infection, improving food security while avoiding the introduction of foreign DNA (Food). Sustainable and cost-effective biofuels are attractive sources for renewable energy, which could be achieved by ...
The Genetics Ph.D. program provides opportunities for graduate study in all major areas of modern genetics, including identification and analysis of human disease genes, molecular evolution, gene therapy, statistical genetics, application of model organisms to problems in biology and medicine, and computational and experimental approaches to ...
This thesis will look specifically at the more conservative Christian discourse on genetic editing and possible paths for genetic editing research that would be more accepted by Christian communities. The general understanding of the Christian stance on genetic editing is that the Christian tradition simply does not approve it.
Genetic Engineering Topics. Define gene editing, and outline key gene-editing technologies, explaining their impact on genetic engineering. The essential role the human microbiome plays in preventing diseases. The principles of genetic engineering. Project on different types of cloning.
Genetic Engineering Using a Pglo Plasmid. The objective of this experiment is to understand the process and importance of the genetic transformation of bacteria in real time with the aid of extrachromosomal DNA, alternatively referred to as plasmids. Managing Diabetes Through Genetic Engineering.