On this page
When to see a doctor, risk factors, complications.
Malaria is a disease caused by a parasite. The parasite is spread to humans through the bites of infected mosquitoes. People who have malaria usually feel very sick with a high fever and shaking chills.
While the disease is uncommon in temperate climates, malaria is still common in tropical and subtropical countries. Each year nearly 290 million people are infected with malaria, and more than 400,000 people die of the disease.
To reduce malaria infections, world health programs distribute preventive drugs and insecticide-treated bed nets to protect people from mosquito bites. The World Health Organization has recommended a malaria vaccine for use in children who live in countries with high numbers of malaria cases.
Protective clothing, bed nets and insecticides can protect you while traveling. You also can take preventive medicine before, during and after a trip to a high-risk area. Many malaria parasites have developed resistance to common drugs used to treat the disease.

Products & Services
- A Book: Mayo Clinic Family Health Book, 5th Edition
- Newsletter: Mayo Clinic Health Letter — Digital Edition
Signs and symptoms of malaria may include:
- General feeling of discomfort
- Nausea and vomiting
- Abdominal pain
- Muscle or joint pain
- Rapid breathing
- Rapid heart rate
Some people who have malaria experience cycles of malaria "attacks." An attack usually starts with shivering and chills, followed by a high fever, followed by sweating and a return to normal temperature.
Malaria signs and symptoms typically begin within a few weeks after being bitten by an infected mosquito. However, some types of malaria parasites can lie dormant in your body for up to a year.
Talk to your doctor if you experience a fever while living in or after traveling to a high-risk malaria region. If you have severe symptoms, seek emergency medical attention.
From Mayo Clinic to your inbox
Malaria is caused by a single-celled parasite of the genus plasmodium. The parasite is transmitted to humans most commonly through mosquito bites.
Mosquito transmission cycle
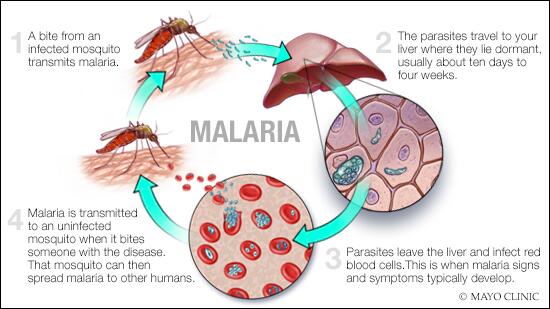
- Malaria transmission cycle
Malaria spreads when a mosquito becomes infected with the disease after biting an infected person, and the infected mosquito then bites a noninfected person. The malaria parasites enter that person's bloodstream and travel to the liver. When the parasites mature, they leave the liver and infect red blood cells.
- Uninfected mosquito. A mosquito becomes infected by feeding on a person who has malaria.
- Transmission of parasite. If this mosquito bites you in the future, it can transmit malaria parasites to you.
- In the liver. Once the parasites enter your body, they travel to your liver — where some types can lie dormant for as long as a year.
- Into the bloodstream. When the parasites mature, they leave the liver and infect your red blood cells. This is when people typically develop malaria symptoms.
- On to the next person. If an uninfected mosquito bites you at this point in the cycle, it will become infected with your malaria parasites and can spread them to the other people it bites.
Other modes of transmission
Because the parasites that cause malaria affect red blood cells, people can also catch malaria from exposure to infected blood, including:
- From mother to unborn child
- Through blood transfusions
- By sharing needles used to inject drugs
The greatest risk factor for developing malaria is to live in or to visit areas where the disease is common. These include the tropical and subtropical regions of:
- Sub-Saharan Africa
- South and Southeast Asia
- Pacific Islands
- Central America and northern South America
The degree of risk depends on local malaria control, seasonal changes in malaria rates and the precautions you take to prevent mosquito bites.
Risks of more-severe disease
People at increased risk of serious disease include:
- Young children and infants
- Older adults
- Travelers coming from areas with no malaria
- Pregnant women and their unborn children
In many countries with high malaria rates, the problem is worsened by lack of access to preventive measures, medical care and information.
Immunity can wane
Residents of a malaria region may be exposed to the disease enough to acquire a partial immunity, which can lessen the severity of malaria symptoms. However, this partial immunity can disappear if you move to a place where you're no longer frequently exposed to the parasite.
Malaria can be fatal, particularly when caused by the plasmodium species common in Africa. The World Health Organization estimates that about 94% of all malaria deaths occur in Africa — most commonly in children under the age of 5.
Malaria deaths are usually related to one or more serious complications, including:
- Cerebral malaria. If parasite-filled blood cells block small blood vessels to your brain (cerebral malaria), swelling of your brain or brain damage may occur. Cerebral malaria may cause seizures and coma.
- Breathing problems. Accumulated fluid in your lungs (pulmonary edema) can make it difficult to breathe.
- Organ failure. Malaria can damage the kidneys or liver or cause the spleen to rupture. Any of these conditions can be life-threatening.
- Anemia. Malaria may result in not having enough red blood cells for an adequate supply of oxygen to your body's tissues (anemia).
- Low blood sugar. Severe forms of malaria can cause low blood sugar (hypoglycemia), as can quinine — a common medication used to combat malaria. Very low blood sugar can result in coma or death.
Malaria may recur
Some varieties of the malaria parasite, which typically cause milder forms of the disease, can persist for years and cause relapses.
If you live in or are traveling to an area where malaria is common, take steps to avoid mosquito bites. Mosquitoes are most active between dusk and dawn. To protect yourself from mosquito bites, you should:
- Cover your skin. Wear pants and long-sleeved shirts. Tuck in your shirt, and tuck pant legs into socks.
- Apply insect repellent to skin. Use an insect repellent registered with the Environmental Protection Agency on any exposed skin. These include repellents that contain DEET, picaridin, IR3535, oil of lemon eucalyptus (OLE), para-menthane-3,8-diol (PMD) or 2-undecanone. Do not use a spray directly on your face. Do not use products with oil of lemon eucalyptus (OLE) or p-Menthane-3,8-diol (PMD) on children under age 3.
- Apply repellent to clothing. Sprays containing permethrin are safe to apply to clothing.
- Sleep under a net. Bed nets, particularly those treated with insecticides, such as permethrin, help prevent mosquito bites while you are sleeping.
Preventive medicine
If you'll be traveling to a location where malaria is common, talk to your doctor a few months ahead of time about whether you should take drugs before, during and after your trip to help protect you from malaria parasites.
In general, the drugs taken to prevent malaria are the same drugs used to treat the disease. What drug you take depends on where and how long you are traveling and your own health.
The World Health Organization has recommended a malaria vaccine for use in children who live in countries with high numbers of malaria cases.
Researchers are continuing to develop and study malaria vaccines to prevent infection.
Feb 09, 2023
- AskMayoExpert. Malaria. Rochester, Minn.: Mayo Foundation for Medical Education and Research; 2018.
- Jameson JL, et al., eds. Malaria. In: Harrison's Principles of Internal Medicine. 20th ed. New York, N.Y.: The McGraw-Hill Companies; 2018. https://accessmedicine.mhmedical.com. Accessed Oct. 9, 2018.
- Tintinalli JE, et al., eds. Malaria. In: Tintinalli's Emergency Medicine: A Comprehensive Study Guide. 8th ed. New York, N.Y.: McGraw-Hill Education; 2016. http://www.accessmedicine.mhmedical.com. Accessed Oct. 9, 2018.
- Malaria. Merck Manual Professional Version. http://www.merckmanuals.com/professional/infectious-diseases/extraintestinal-protozoa/malaria. Accessed Oct. 9, 2018.
- Malaria. Centers for Disease Control and Prevention. http://wwwnc.cdc.gov/travel/diseases/malaria. Accessed Nov. 6, 2015.
- Breman JG. Clinical manifestations of malaria in nonpregnant adults and children. https://www.uptodate.com/contents/search. Accessed Oct. 9, 2018.
- Daily J. Treatment of uncomplicated falciparum malaria in nonpregnant adults and children. https://www.uptodate.com/contents/search. Accessed Oct. 9, 2018.
- Key points: World malaria report 2017. World Health Organization. https://www.who.int/malaria/media/world-malaria-report-2017/en/. Accessed Oct. 9, 2018.
- Malaria. World Health Organization. https://www.who.int/malaria/en/. Accessed Oct. 9, 2018.
- Mutebi JP, et al. Protection against mosquitoes, ticks, & other arthropods. In: CDC Yellow Book 2018: Health Information for International Travelers. https://wwwnc.cdc.gov/travel/yellowbook/2018/the-pre-travel-consultation/protection-against-mosquitoes-ticks-other-arthropods. Accessed Oct. 27, 2018.
- Diseases & Conditions
- Malaria symptoms & causes
News from Mayo Clinic
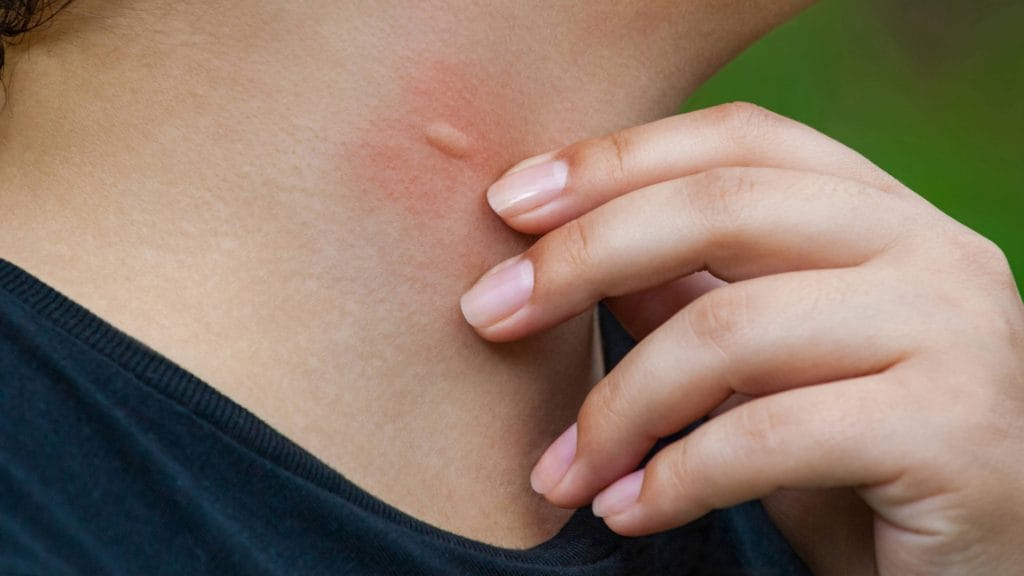
More Information
CON-XXXXXXXX
Your gift holds great power – donate today!
Make your tax-deductible gift and be a part of the cutting-edge research and care that's changing medicine.
Malaria Journal
- Most accessed
- Collections
Considerations for first field trials of low-threshold gene drive for malaria vector control
Authors: John B. Connolly, Austin Burt, George Christophides, Abdoulaye Diabate, Tibebu Habtewold, Penelope A. Hancock, Anthony A. James, Jonathan K. Kayondo, Dickson Wilson Lwetoijera, Alphaxard Manjurano, Andrew R. McKemey, Michael R. Santos, Nikolai Windbichler and Filippo Randazzo
Population pharmacokinetics of primaquine and its metabolites in African males
Authors: Palang Chotsiri, Almahamoudou Mahamar, Halimatou Diawara, Pius S. Fasinu, Kalifa Diarra, Koualy Sanogo, Teun Bousema, Larry A. Walker, Joelle M. Brown, Alassane Dicko, Roly Gosling, Ingrid Chen and Joel Tarning
Assessment of environmental and spatial factors influencing the establishment of Anopheles gambiae larval habitats in the malaria endemic province of Woleu-Ntem, northern Gabon
Authors: Neil-Michel Longo-Pendy, Silas Lendzele Sevidzem, Boris Kevin Makanga, Saturnin Ndotit-Manguiengha, Stravensky Térence Boussougou-Sambe, Piazzy Obame Ondo Kutomy, Judicaël Obame-Nkoghe, Lynda-Chancelya Nkoghe-Nkoghe, Barclaye Ngossanga, Felicien Kassa Mvoubou, Christophe Roland Zinga Koumba, Ayôla Akim Adegnika, Abdul-Safiou Razack, Jacques François Mavoungou and Rodrigue Mintsa-Nguema
Field testing of user-friendly perennial malaria chemoprevention packaging in Benin, Côte d’Ivoire and Mozambique
Authors: Sylvain Landry Birane Faye, Maud Majeres Lugand, André Touré Offianan, Aurélie Dossou-Yovo, Dieudonné Kouakou M’Bra Kouadio and Felix Pinto
Exploring existing malaria services and the feasibility of implementing community engagement approaches amongst conflict-affected communities in Cameroon: a qualitative study
Authors: Margaret Ebob Besem E.O, Elisabeth G. Chestnutt, Laura Donovan, Ann-Sophie Stratil, Helen Counihan, Claude Ngwayu Nkfusai, Helen Hawkings, Blanka Homolova, Kolawole Maxwell, Kevin Baker, Yakouba Zoungrana, Elvis Asangbeng Tanue, Glennise Ayuk, Noukeme Bibiche Modjenpa, Alain Metuge, Isabelle Nganmou…
Most recent articles RSS
View all articles
Plant-based insect repellents: a review of their efficacy, development and testing
Authors: Marta Ferreira Maia and Sarah J Moore
Quinine, an old anti-malarial drug in a modern world: role in the treatment of malaria
Authors: Jane Achan, Ambrose O Talisuna, Annette Erhart, Adoke Yeka, James K Tibenderana, Frederick N Baliraine, Philip J Rosenthal and Umberto D'Alessandro
Effectiveness of plant-based repellents against different Anopheles species: a systematic review
Authors: Amin Asadollahi, Mehdi Khoobdel, Alireza Zahraei-Ramazani, Sahar Azarmi and Sayed Hussain Mosawi
Foul wind, spirits and witchcraft: illness conceptions and health-seeking behaviour for malaria in the Gambia
Authors: Sarah O’Neill, Charlotte Gryseels, Susan Dierickx, Julia Mwesigwa, Joseph Okebe, Umberto d’Alessandro and Koen Peeters Grietens
Antibiotics in malaria therapy: which antibiotics except tetracyclines and macrolides may be used against malaria?
Authors: Tiphaine Gaillard, Marylin Madamet, Francis Foguim Tsombeng, Jérôme Dormoi and Bruno Pradines
Most accessed articles RSS
World Malaria Day - 2024
Cross Journal Collection
Elimination of infectious diseases of poverty as a key contribution to achieving the SDGs
Thematic series Primate malaria
Cross journal collections - closed
Contribution of climate change to the spread of infectious diseases
Human migration, conflict and infectious diseases
Spatial inequality, infectious diseases and disease control
Thematic series Malaria and COVID-19
Thematic series Malaria Elimination Demonstration Project, Mandla, India
Thematic series Durability Monitoring of Long-lasting Insecticidal Nets: Results and Learning from the VectorWorks Project
Thematic series Alternative interventions to facilitate malaria elimination
Thematic series A combined effect: using indoor residual spraying and insecticide-treated nets together for additional impact
Debate series The Malaria Journal debates Edited by Elizabeth Ashley
Thematic series Targeted next generation sequencing for malaria molecular epidemiology in Africa Edited by Jonathan Juliano
Thematic series Time to go for vivax Edited by: Marcus V Lacerda and Hernando A del Portillo
Thematic series Biomarkers of malaria
Cross Journal Collection 14th International Congress of Parasitology
Thematic series ACT now: anti-malarial market complexity one decade after the introduction of artemisinin combination therapy – evidence from sub-Saharan Africa and the Greater Mekong Sub-region
Thematic series Ivermectin to reduce malaria transmission
Thematic series Housing and malaria Edited by: Dr. Lucy Tusting, Dr. Jo Lines and Barbary Willey
Thematic series Re-imagining malaria – a platform for reflections to widen horizons in malaria control Edited by: Dr. Julian Eckl, Dr. Susanna Hausmann Muela
Cross journal collection Every day is Malaria Day
Cross journal collection Reviewer acknowledgements 2013
Thematic series WHO global malaria recommendations 2012 - 2015
Thematic series The ACTwatch project: monitoring anti-malarial markets in seven countries
Thematic series Travellers' malaria Edited by: Prof Patricia Schlagenhauf
Thematic series National malaria control programme (NMCP) Best Practice Sharing Edited by: Prof Robert William Snow
Thematic series Towards malaria elimination
Thematic series The world antimalarial resistance network (WARN)
Latest Tweets
Your browser needs to have JavaScript enabled to view this timeline
World Malaria Day 2024
Collections open for submissions
Cross journal collection
Alternative interventions to facilitate malaria elimination
Thematic series
Malaria Journal In Review - preprints
Malaria Journal , in partnership with Research Square, is offering In Review . Authors choosing this free optional service will be able to:
- Share their work with fellow researchers to read, comment on, and cite even before publication
- Showcase their work to funders and others with a citable DOI while it is still under review
- Track their manuscript - including seeing when reviewers are invited, and when reports are received
See what the Malaria Journal In Review platform looks like
Aims and Scope
Malaria Journal is aimed at the scientific community interested in malaria in its broadest sense. It is the only journal that publishes exclusively articles on malaria and, as such, it aims to bring together knowledge from the different specialties involved in this very broad discipline, from the bench to the bedside and to the field.
Editor-in-Chief - Marcel Hommel, University of Liverpool, UK
- Editorial Board
- Manuscript editing services
- SNAPP Editor link
- Instructions for Editors
- Contact Support for Editors
- Sign up for article alerts and news from this journal
- Follow us on Twitter
Annual Journal Metrics
2022 Citation Impact 3.0 - 2-year Impact Factor 3.2 - 5-year Impact Factor 1.148 - SNIP (Source Normalized Impact per Paper) 1.237 - SJR (SCImago Journal Rank)
2023 Speed 7 days submission to first editorial decision for all manuscripts (Median) 131 days submission to accept (Median)
2023 Usage 4,093,320 downloads 5,053 Altmetric mentions
- More about our metrics
ISSN: 1475-2875
- Submission enquiries: [email protected]
- Search Menu
- Advance articles
- Editor's Choice
- Special Issues
- Supplements
- Lessons from the field
- Open Access Articles
- Author Guidelines
- Submission Site
- Open Access
- For Reviewers
- About the Journal
- About the Royal Society of Tropical Medicine and Hygiene
- Editorial Board
- Advertising and Corporate Services
- Journals Career Network
- Self-Archiving Policy
- Dispatch Dates
- Journals on Oxford Academic
- Books on Oxford Academic
Article Contents
Introduction, authors’ contributions, acknowledgements, competing interests, ethical approval, data availability, disclaimers, climate change, malaria and neglected tropical diseases: a scoping review.

- Article contents
- Figures & tables
- Supplementary Data
Petra Klepac, Jennifer L Hsieh, Camilla L Ducker, Mohamad Assoum, Mark Booth, Isabel Byrne, Sarity Dodson, Diana L Martin, C Michael R Turner, Kim R van Daalen, Bernadette Abela, Jennifer Akamboe, Fabiana Alves, Simon J Brooker, Karen Ciceri-Reynolds, Jeremy Cole, Aidan Desjardins, Chris Drakeley, Dileepa S Ediriweera, Neil M Ferguson, Albis Francesco Gabrielli, Joshua Gahir, Saurabh Jain, Mbaraka R John, Elizabeth Juma, Priya Kanayson, Kebede Deribe, Jonathan D King, Andrea M Kipingu, Samson Kiware, Jan Kolaczinski, Winnie J Kulei, Tajiri L Laizer, Vivek Lal, Rachel Lowe, Janice S Maige, Sam Mayer, Lachlan McIver, Jonathan F Mosser, Ruben Santiago Nicholls, Cláudio Nunes-Alves, Junaid Panjwani, Nishanth Parameswaran, Karen Polson, Hale-Seda Radoykova, Aditya Ramani, Lisa J Reimer, Zachary M Reynolds, Isabela Ribeiro, Alastair Robb, Kazim Hizbullah Sanikullah, David R M Smith, GloriaSalome G Shirima, Joseph P Shott, Rachel Tidman, Louisa Tribe, Jaspreet Turner, Susana Vaz Nery, Raman Velayudhan, Supriya Warusavithana, Holly S Wheeler, Aya Yajima, Ahmed Robleh Abdilleh, Benjamin Hounkpatin, Dechen Wangmo, Christopher J M Whitty, Diarmid Campbell-Lendrum, T Déirdre Hollingsworth, Anthony W Solomon, Ibrahima Socé Fall, Climate change, malaria and neglected tropical diseases: a scoping review, Transactions of The Royal Society of Tropical Medicine and Hygiene , 2024;, trae026, https://doi.org/10.1093/trstmh/trae026
- Permissions Icon Permissions
To explore the effects of climate change on malaria and 20 neglected tropical diseases (NTDs), and potential effect amelioration through mitigation and adaptation, we searched for papers published from January 2010 to October 2023. We descriptively synthesised extracted data. We analysed numbers of papers meeting our inclusion criteria by country and national disease burden, healthcare access and quality index (HAQI), as well as by climate vulnerability score. From 42 693 retrieved records, 1543 full-text papers were assessed. Of 511 papers meeting the inclusion criteria, 185 studied malaria, 181 dengue and chikungunya and 53 leishmaniasis; other NTDs were relatively understudied. Mitigation was considered in 174 papers (34%) and adaption strategies in 24 (5%). Amplitude and direction of effects of climate change on malaria and NTDs are likely to vary by disease and location, be non-linear and evolve over time. Available analyses do not allow confident prediction of the overall global impact of climate change on these diseases. For dengue and chikungunya and the group of non-vector-borne NTDs, the literature privileged consideration of current low-burden countries with a high HAQI. No leishmaniasis papers considered outcomes in East Africa. Comprehensive, collaborative and standardised modelling efforts are needed to better understand how climate change will directly and indirectly affect malaria and NTDs.
Human activity is driving incremental changes in climate patterns globally. The burning of coal, oil and natural gas; clearing land by cutting down forests; and intensified agriculture all release greenhouse gases, primarily CO 2 , methane and N 2 O. Deforestation also reduces capacity for CO 2 absorption. Increases in atmospheric concentrations of greenhouse gases are driving mean global temperatures upwards, with consequent effects including rising sea levels, changes in rainfall and increases in the frequency and intensity of extreme weather events. 1
Climate change will perturb human health in profound and long-lasting ways, both directly and indirectly. 2 Multiple direct and indirect mechanisms will contribute. Human physiology can be affected directly by changes in air temperature, humidity, stress and diet. Human behaviour can be altered by changing weather, changing economic circumstances, migration, natural disasters and access to or quality of healthcare. Environmental conditions also influence pathogen transmission, reproduction, development and genetics; the reproduction, development, genetics, behaviour, range, longevity and predation of vectors, intermediate hosts and reservoir hosts; and the feasibility and effectiveness of interventions. In fact, there is already empirical evidence of climate change having amplified more than one-half of all known human infectious diseases. 3
The impact of climate change is likely to be disproportionately borne by the poorest people, who are also disproportionately affected by malaria and neglected tropical diseases (NTDs; Table 1 ). In part because of this association with poverty, malaria and NTDs are often co-endemic. Many of these diseases are currently suitable for coordinated control via integrated programmes. 4–7
Diseases and organisms in scope for this review. Included diseases and associated aetiological agents, vectors, reservoir and intermediate hosts and routes of transmission are those listed in the NTD road map 2021–2030, 13 plus malaria. Noma was added to the NTD list in December 2023, 61 and therefore is not included here.
a Ancylostoma ceylanicum is a soil-transmitted helminth species previously believed to only infect dogs but now recognised as a zoonosis that causes symptomatic infections in humans; it was not included in the NTD road map 2021–2030 13 and therefore was not included in this review.
Developing an understanding of the likely effects of climate change on the epidemiology of malaria and NTDs is critical to minimising their health implications. This scoping review explores current predictions of the effects of historical and future climate change on malaria and NTDs, and the potential amelioration of these effects through climate change mitigation and adaptation strategies.
A comprehensive scoping review was conducted. To complete the review efficiently but with high fidelity, we used automation and artificial intelligence-based tools to help define search terms, translate them across different platforms, de-duplicate search results and conduct title-and-abstract screening. 8 We did not otherwise use artificial intelligence assistance. This report adheres to the Preferred Reporting Items for Systematic Reviews and Meta-Analyses extension for Scoping Reviews guidance 9 ( Supplementary Table 1 ).
Search strategy
Searches were conducted in five electronic databases that index the published peer-reviewed and grey literature: PubMed, Scopus, Embase via Ovid, Global Index Medicus and the WHO's Institutional Repository for Information Sharing.
Databases were searched for papers published from 1 January 2010 to 12 October 2023. No language or other restrictions were applied. Using a combination of Medical Subject Heading (MeSH) and free-text terms, search vocabulary related to ‘climate change’, ‘malaria’ and ‘neglected tropical diseases’ was employed. Searches were designed and conducted in English and included scientific and lay expressions for diseases, pathogens, vectors, reservoir hosts and intermediate hosts; the search strategy was first constructed for PubMed, and the Systematic Review Accelerator Polyglot tool ( https://sr-accelerator.com/#/polyglot ) 10 was then used to translate strings into syntax appropriate for other databases. (Please see the Supplementary material for full search strings.) Using the citationchaser Shiny app ( https://www.eshackathon.org/software/citationchaser.html ), 11 forward and backward screening of relevant seed papers (e.g. reviews, commentaries) was undertaken in December 2023 to identify additional records. The review protocol was not published in advance.
Criteria for paper selection
Inclusion criteria applied to screening are shown in Table 2 . Papers not meeting these criteria were excluded.
Characteristics of included papers.
Papers were eligible for inclusion if they explicitly reported on the observed or modelled effects of historical or future climate change on outcomes framing the distribution, dynamics or transmission of malaria or NTDs; or the range, abundance or transmission potential of their vectors, reservoir hosts or intermediate hosts. Papers were also included if they explicitly reported on the observed or modelled effects of climate change mitigation and adaptation strategies on these outcomes. (‘Climate change mitigation’ was defined as actions intended to reduce the magnitude of climate change, and ‘adaptation’ as actions intended to reduce the vulnerability of human and natural systems to the effects of climate change or to cope with past or future climate change.)
Masters and PhD theses were eligible for inclusion if the analyses were not also presented elsewhere, but we did not specifically search thesis repositories.
Papers were excluded if (i) they did not include original analyses; (ii) they presented methods or protocols without results; (iii) they were clinical case reports; (iv) they were conference proceedings; (v) they were pre-prints (of papers being prepared for, or in the process of being considered by, peer-reviewed journals); or (vi) the full text could not be obtained. In contrast to the exclusion of clinical case reports, we included ecological case reports as potentially important indicators of pathogen or vector range expansion.
Screening and extraction procedures
The Covidence software package ( https://www.covidence.org/ ) was used to support record indexing, removal of duplicate records, title-and-abstract screening, full-text screening and compilation of extracted data.
After de-duplication, titles and abstracts of each record were independently screened by two researchers, with conflicts resolved by a third independent reviewer. Non-English records were translated or reviewed by a fluent speaker of that language.
The Covidence title-and-abstract screening module incorporates a machine-learning algorithm that ranks papers that are yet to be screened according to their acceptance likelihood: based on previous screening decisions, it pushes papers that are more likely to be accepted towards the top of the pile, for all reviewers. This accelerates the screening process by removing the need to screen all search results. The predetermined stopping rule was to discontinue title-and-abstract screening after three criteria were met: (i) more than twice the estimated fraction of relevant records from a previously published systematic review of climate change and NTDs 12 had been screened; (ii) all papers previously determined as relevant (‘seed’ papers) were identified; and (iii) two screeners had each rejected 50 papers in a row. The results of the backward and forward citation searches of seed papers were screened separately with the assistance of the same (trained) machine-learning algorithm, with screening of these records discontinued after a single screener had rejected 100 papers in a row.
Full texts were blind double-screened, with discrepant results arbitrated by an independent third reviewer.
Data from papers selected in the full-text review were independently extracted by one investigator using a pre-piloted standardised form, according to self-identified individual expertise, and checked by a second investigator. Data extraction was limited to the information provided in the published work; authors were not contacted for further clarification or provision of missing information. Extracted information included: first author, year, study title, diseases addressed in the publication, study design (cohort, field, model, laboratory research, ecological case report), whether the paper explicitly discussed adaptation or mitigation strategies, the time frame of the study (cross-sectional, prospective longitudinal, retrospective longitudinal; the length of the time series), location data, and the population, intervention/exposure, comparator and outcome (Table 2 ).
Structured assessment of the methodological limitations and risk of bias of included individual papers was not undertaken.
Data analyses and synthesis
Because of the heterogeneity of included studies in terms of designs, exposures, outcomes and quality, no meta-analyses were attempted. Data were narratively synthesised and thematically categorised to facilitate analysis.
Papers were grouped and synthesised by whether they (i) explored the effect of climate change on malaria and NTDs; or (ii) examined potential amelioration of these effects through climate change mitigation and adaptation. Studies that compared several future climate scenarios of different severities (different representative concentration pathways [RCPs], or different combinations of RCPs and shared socioeconomic pathways, for example; please see Box 1 and Figure 1 ) were defined as projections of the effect of mitigation.
![malaria disease essay Comparison of Intergovernmental Panel on Climate Change (IPCC) scenarios used in papers that met this review's inclusion criteria. The different categories of scenario (special report on emission scenarios [SRES; released in 2000]; representative concentration pathways [RCPs; 2014]; shared socioeconomic pathways [SSP; 2021]; scenarios from the IPCC Sixth Assessment Report Working Group III [AR6 WGIII; 2022]) are laid out in columns against the equivalent scenarios within the full RCP scheme, which are laid out in rows; some of the RCP scenarios (RCP1.9, RCP3.4 and RCP7) were added after the original 2014 publication of the RCP system. (The RCPs were the most commonly applied scenarios used in papers that met this review's inclusion criteria.) Further explanations of all of these scenario categories are included in Box 1.81–83](https://oup.silverchair-cdn.com/oup/backfile/Content_public/Journal/trstmh/PAP/10.1093_trstmh_trae026/3/m_trae026fig1.jpeg?Expires=1719361455&Signature=gLjf2SjLprYYeqCQJatjB1RZNgW2-adG9yXne9XZWB3AKXcFzdpO83F~ge6IF7W67Nz1k4z~SdBXTD-wTVtlbrh1G8ZGrve80M7jIzvrW1Tru9oEWCQ3IRWsnH~Uvy3AB3YFefg-VVwh8~DXqUJhe0uunF3CebLDCSVzFAJ4ah76nvSQs5xvuyvjmwH3Zi1K0hyqtp17ZhXmH5Iuis0jdTiEAXZ62GOP-Dln2aZB~0RTR5yQ7K2Hc6XcHqMSCwprFdJLMHY8HuNw8oh-6Ham67KG7YmBSkzqrbc9~TJuTmH7vgofJOZkOVwZHJfuRQ8CKUj2nHfI3Kro6HbvKE83Ng__&Key-Pair-Id=APKAIE5G5CRDK6RD3PGA)
Comparison of Intergovernmental Panel on Climate Change (IPCC) scenarios used in papers that met this review's inclusion criteria. The different categories of scenario (special report on emission scenarios [SRES; released in 2000]; representative concentration pathways [RCPs; 2014]; shared socioeconomic pathways [SSP; 2021]; scenarios from the IPCC Sixth Assessment Report Working Group III [AR6 WGIII; 2022]) are laid out in columns against the equivalent scenarios within the full RCP scheme, which are laid out in rows; some of the RCP scenarios (RCP1.9, RCP3.4 and RCP7) were added after the original 2014 publication of the RCP system. (The RCPs were the most commonly applied scenarios used in papers that met this review's inclusion criteria.) Further explanations of all of these scenario categories are included in Box 1 . 81–83
Climate change scenarios.
The Intergovernmental Panel on Climate Change (IPCC) has periodically published assessment reports on the scientific understanding of climate change, its projected effects and potential response strategies. As a part of these reports, the IPCC outlines future climate scenarios or pathways based on projections from Global Climate Models, for use in modelling. Papers meeting the inclusion criteria for this review used a variety of different future scenarios, so their outcomes and conclusions generally cannot be directly compared. Here, we summarise the history of IPCC climate scenarios, explain their interpretation and note how they relate to each other (Figure 1 ).
IP92 –the first global climate change scenarios, published by IPCC in 1992. These focused on projected future emissions of greenhouse gases (GHGs). 78
Special Report on Emission Scenarios (SRES)—released in 2000 and used in the IPCC's Third Assessment Report (TAR) and Fourth Assessment Report (AR4). The SRES covers a wide range of the main drivers of climate change, from demographic to technological and economic developments, and includes all relevant GHGs and sulphur, and their driving forces. 79 Four main narrative storylines with different contributions of drivers cover a range of possible outcomes:
A1: Rapid economic growth; population peaks mid-century then declines.
A2: Regionalised world; high population growth.
B1: Global sustainability focus; population peaks mid-century then declines.
B2: Local solutions emphasised; slow population growth.
Representative Concentration Pathways (RCPs)—Developed to replace the SRES, for use in climate models as part of the Fifth Assessment Report (AR5) of the IPCC in 2014. Rather than being based on storylines, RCPs describe possible GHG emissions and concentration levels in the atmosphere by 2100, ranging from very low (RCP2.6, radiative forcing peaks at 3 Wm −2 and then declines to 2.6 Wm −2 by 2100, also known as RCP3PD) to intermediate (RCP4.5 and RCP6.0, radiative forcing is stabilised at approximately 4.5 or 6.0 Wm −2 ), to very high (RCP8.5, radiative forcing continues to increase after 2100). RCPs use time series of emissions and concentrations of the full suite of GHGs, aerosols and chemically active gases, as well as projected land use/land cover and population growth. Compared with the SRES, RCP2.6 has no equivalent; RCP4.5 is similar to SRES B1 but median temperatures rise more quickly in RCP4.5 than in B1 in the first half of the twenty-first century, and then more slowly in the second half; RCP6.0 is similar to SRES B2, with median temperatures rising more quickly in RCP6.0 than in B2 from 2060–2090, but otherwise more slowly; and RCP8.5 is similar to SRES A1FI (A1 fossil-fuel intensive scenario), with median temperatures rising more slowly in RCP8.5 than in A1FI during 2035–2080, but more quickly during other periods. 80 , 81
Shared Socioeconomic Pathways (SSPs)—These scenarios were introduced in the IPCC's Sixth Assessment Report (AR6) published in 2021. SSPs are integrated scenarios that combine socioeconomic narratives with emission pathways, describing different plausible futures of societal development based on varying assumptions about demographic, economic, social and technological factors. Briefly, these include:
SSP1 (Sustainability): represents a future in which there is rapid economic growth, low population growth and widespread adoption of environmentally friendly technologies. It is also called ‘Taking the Green Road’ and emphasises sustainable development, international cooperation and a focus on environmental stewardship.
SSP2 (Middle of the Road): represents a future in which trends continue along historic trajectories with moderate economic growth, intermediate population growth and technological progress occurring at a moderate pace. It assumes some improvements in living standards and governance but also incorporates challenges and disparities.
SSP3 (Regional Rivalry): represents a future characterised by high population growth, slow economic development and fragmented governance. Also termed ‘A Rocky Road’, it envisions regions prioritising national interests over global cooperation, resulting in regional rivalries, conflicts and limited efforts to address climate change.
SSP4 (Inequality): represents a future marked by high population growth, slow economic development and high income inequality. It assumes limited international cooperation and emphasises national interests and security concerns over environmental issues. Also termed ‘A Road Divided’, this scenario suggests challenges in achieving sustainable development and addressing climate change due to social disparities.
SSP5 (Fossil-Fuelled Development): represents a future with high population growth, rapid economic development and reliance on fossil fuels. Also termed ‘Taking the Highway’, it envisions limited environmental regulations and technological innovation, leading to high GHG emissions and significant impact from climate change. 82
AR6 WGIII —scenario categories C1-C8 (published in 2022 by the IPCC's Sixth Assessment Report AR6 Working Group III) refer to potential mitigation pathways used to reduce GHG emissions and limit global warming. These scenarios relate to warming levels in the twenty-first century:
C1 (1.5°C, no/limited overshoot). Limits warming to 1.5°C by 2100 (with >50% likelihood). Roughly corresponds to SSP1-1.9 (very low).
C2 (1.5°C, high overshoot). After a high overshoot, warming returns to 1.5°C by 2100 (with >50% likelihood). Roughly corresponds to SSP1-2.6 (low).
C3 (Likely below 2°C). Limits warming to 2°C by 2100 (with >67% likelihood).
C4 (Below 2°C). Limits warming to 2°C by 2100 (with >50% likelihood).
C5 (Below 2.5°C). Limits warming to 2.5°C by 2100 (with >50% likelihood).
C6 (Below 3°C). Limits warming to 3°C by 2100 (with >50% likelihood). Roughly corresponds to SSP2-4.5 (intermediate).
C7 (Below 4°C). Limits warming to 4°C by 2100 (with >50% likelihood). Roughly corresponds to SSP3-7.0 (high).
C8 (Above 4°C). Exceeds warming of 4°C by 2100 (with >50% likelihood). Roughly corresponds to SSP5-8.5 (very high). 83
Based on the volume of literature meeting the inclusion criteria, papers were further grouped by disease into malaria, dengue and chikungunya, other vector-borne NTDs and other non-vector-borne NTDs. Dengue and chikungunya are bracketed as an NTD group in the 2021–2030 NTD road map. 13 We categorised as ‘vector-borne NTDs’ those for which vector control was a recommended control strategy in the 2021–2030 NTD road map. 13
We obtained data on the burden of malaria and NTDs by country, quantified as disability-adjusted life years (DALYs, the cumulative number of years lost by a defined population due to illness, disability and death from a particular cause or group of causes), from the 2019 Global Burden of Disease study. 14 To account for different national levels of health service delivery, we used the healthcare access and quality index (HAQI). This employs data on 32 causes of death from which mortality would be avoidable in the presence of effective healthcare; a high index is a marker of better healthcare. HAQIs were obtained from the 2019 Global Burden of Disease data. 15 To account for countries’ exposure, sensitivity and ability to adapt to the negative impacts of climate change, we used the 2021 vulnerability score from the Notre Dame Global Adaptation Initiative (ND-GAIN; available at: https://gain-new.crc.nd.edu/ranking/vulnerability ). The ND-GAIN measures vulnerability by considering six life-supporting sectors: food, water, health, ecosystem service, human habitat and infrastructure. Countries ranked higher in the index are those that are less vulnerable. After removing papers that examined outcomes at the global level, local polynomial regression was undertaken to predict the number of disease group-specific publications relevant to each country as a function of the country's (i) DALYs for that group of diseases, (ii) HAQI and (iii) climate vulnerability score. As there were <1000 datapoints available for each regression, we used locally estimated scatterplot smoothing (LOESS) for these analyses.
Database searches yielded 31 560 records. Forward and backward citation searching yielded an additional 11 133 (of which 8587 were within the specified publication range), producing a combined total of 42 693 records (40 147 within the specified range). After removal of 14 879 duplicates, 27 814 records were available for title-and-abstract screening. The stopping rules for title-and-abstract screening were met after 9013 records had been examined; of these 9013, 7442 (83%) were excluded on the basis that their titles and abstracts indicated a failure to meet the inclusion criteria. Full-text papers were therefore sought for 1571 records (17% of 9013), of which 1543 (98%) were able to be retrieved. Following full-text review, 511 papers (33% of 1543) were included for data extraction; these are summarised in Supplementary Table 2 . Extracted data are presented in full in Supplementary Table 3 . A flow diagram is included as Figure 2 .

Records included and excluded at each review stage.
Papers that met the inclusion criteria considered outcomes relevant to malaria (185 papers), dengue and chikungunya (181), the leishmaniases (53), schistosomiasis (29), Chagas disease (19), foodborne trematodiases (17), lymphatic filariasis (14), snakebite envenoming (11), rabies (9), human African trypanosomiasis (8), Buruli ulcer (6), echinococcosis (4), onchocerciasis (2), leprosy (1), scabies (1) and soil-transmitted helminthiases (1). No papers meeting the inclusion criteria considered outcomes relevant to dracunculiasis; mycetoma, chromoblastomycosis or other deep mycoses; taeniasis/cysticercosis; trachoma; or yaws (Figure 3A ).

Number of papers by year and total number of papers meeting the inclusion criteria for each (A) disease or disease group, and (B) type of study.
The vast majority of papers used modelling to study the effect of climate change (435 papers). There were 72 field study papers, 54 laboratory research reports, 28 ecological case reports, 10 cohort studies and seven other types of study (Figure 3 B; multiple study types were possible in a single paper). There was little variation in the numbers of papers from year to year (Figure 3 A and B, left plots).
Among papers meeting the inclusion criteria, a total of 174 (35%) considered the possible ameliorating effect of climate change mitigation for any outcome, 24 (5%) considered adaption strategies and two considered both. Sixty-nine papers considered mitigation in relation to malaria outcomes. Nine explicitly considered adaptation strategies for malaria outcomes. Fifty-three papers addressed climate change mitigation and nine considered adaptation strategies in relation to dengue and chikungunya; four of the papers, including mitigation analyses for dengue and chikungunya, also considered malaria outcomes in the light of climate change mitigation. Sixty other papers addressed climate change mitigation or adaptation with respect to NTD outcomes.
There was wide variation in the geographical coverage of papers by disease (Figure 4 ). Apart from malaria, dengue and chikungunya, and schistosomiasis, there were <10 papers per disease per country for every country, with many endemic countries not featured in any papers for several NTDs. We also observed distinct distribution patterns for malaria, dengue and chikungunya, and schistosomiasis. Most papers examining the impact of climate change on malaria focused on countries in Africa, Brazil, China or India. Dengue and chikungunya papers were focused on Australia, China, India, countries in Europe and the USA, many of which are countries where these diseases may spread in future years. Several schistosomiasis papers focused on China. For leishmaniasis, papers meeting our inclusion criteria considered countries that were widely distributed around the globe, with the exception of countries in East Africa.

Geographical coverage of papers by disease. Colours represent the total number of papers that met the inclusion criteria, per disease, across countries.
The observed variation in geographical coverage of papers across diseases prompted us to further investigate the links between study location, number of papers, disease burden and country vulnerability to climate change. Given the discrepancy in the number of papers between diseases, we grouped these into four categories: (i) malaria; (ii) dengue and chikungunya; (iii) other vector-borne NTDs (Chagas disease, dracunculiasis, human African trypanosomiasis, leishmaniasis, lymphatic filariasis, onchocerciasis, schistosomiasis, trachoma); and (iv) non-vector-borne NTDs (Buruli ulcer, echinococcosis, foodborne trematodiases, leprosy, rabies, scabies/tungiasis, soil-transmitted helminthiases, snakebite envenoming, taeniasis and cysticercosis).
Our analysis showed different patterns across groups of diseases. For malaria there were clear trends towards more papers covering countries with a high malaria DALY burden, low HAQI and high vulnerability to climate change (Figure 5 , first column). For dengue and chikungunya, there was a trend towards increasing numbers of papers covering areas with high burden, but at low DALY burden there was an increase in papers due to the relatively large number of studies looking at potential expansion of these diseases into new areas (Figure 5 , second column). This also meant that there was a relative abundance of papers studying these diseases in areas where there is good access to healthcare, and where the climate vulnerability score is low. For the remaining vector-borne NTDs (Figure 5 , third column), there was a suggestion of an increasing numbers of papers addressing countries with increasing burden and decreasing HAQI, but no suggestion that analyses were more commonly focused on areas with high climate vulnerability. For the non-vector-borne NTD group, papers more frequently considered countries with high DALY burden for that disease, high HAQI and low climate vulnerability (Figure 5 , fourth column).

Numbers of papers meeting the inclusion criteria by disease or disease group, compared with country-level (A) DALYs for the disease or disease group; (B) health access and quality index; and (C) climate vulnerability score. Studies with outcomes reported at global level (for all countries) were removed for these analyses. Each circle represents one country; superimposition of multiple circles makes some look darker than others. Lines show locally estimated scatterplot smoothing (LOESS)-generated local polynomial regression.
Detailed analysis of the full text of papers meeting the inclusion criteria revealed additional insights. For malaria, the consensus among papers that met the inclusion criteria was that global warming will extend the area in which transmission is possible to some areas where it was previously too cold for vector or parasite development, while conditions in some currently endemic areas may become too severe to maintain transmission. Zones suitable for transmission may shift both poleward and upwards in altitude. Future expansion could be balanced by more frequent droughts, making environmental conditions unsuitable for transmission in some previously endemic areas, including parts of the Sahel. 16 Other papers concluded that malaria transmission seasons will last longer, as more months of the year will have a suitable climate. 17 While these changes could place a greater proportion of the global population at risk, 18 the potential net impact of climate change on the global burden of malaria remains unclear; 19–22 papers that met the inclusion criteria were vastly different and difficult to compare with each other. Making predictions for regional and global populations was previously difficult because of a general lack of high-resolution monthly incidence data. 23 There is likely to be small-scale heterogeneity in effects on the ground.
One aspect of climate change that is already impacting malaria transmission is the increasing frequency of extreme weather events. Severe flooding in Pakistan in 2022 and cyclones in Mozambique and Madagascar in 2023 were accompanied by local spikes in malaria cases, driven by breeding of Anopheles mosquitos in flood waters. 24
For dengue and chikungunya, a significant proportion of papers that met our inclusion criteria described subnational studies with subdecadal time frames. Models with broader geographic and temporal boundaries predict dramatic expansion in the future range of relevant Aedes vector species, 25–27 in line with trends that are already being observed. 28–30 Some local retreat from geographies currently occupied by Aedes spp. is also predicted. 31 Alongside the overall increase in vector range, considerable increases are projected in the number of future dengue cases under more adverse climate change scenarios (Box 1 ) in some models. 30 , 32 Other models predict a plateauing of dengue in highly endemic regions by 2050. 28 There is undoubtedly uncertainty about the magnitude and direction of future geographic expansion.
For the leishmaniases, 53 papers that met the inclusion criteria considered relevant outcomes. However, there are many different pathogens and vector species contributing to this complex of diseases (Table 1 ), making a reliable, complete picture very difficult to draw. Several large-scale models predict changes in the range of relevant sandfly species around the Mediterranean and in the Americas, with transmission in the latter extending as far north as southern Canada in some future climate scenarios. 33–37 The number of people in North America living in areas in which leishmaniasis is transmitted could double from 2010 to 2080 under SRES B2. 37 The paucity of information on the potential impact of climate change on leishmaniasis in Africa (Figure 4 ) was striking.
For schistosomiasis, one model predicted increased prevalence and intensity of infection in some areas of East Africa over the next few decades, particularly in Rwanda, Burundi, south-west Kenya and eastern Zambia, with concurrent substantial decreases in risk in parts of Kenya, southern South Sudan and eastern Democratic Republic of the Congo. 38 Decreases in transmission are predicted for China, 39 although the possibility was also invoked that disease will re-emerge in parts of mainland China where it was previously eliminated. 40 This concern may account for the relative concentration of publications that focused on China (Figure 4 ).
There were few papers on the remaining NTDs, with a bias towards vector-borne diseases, possibly due to the existence of established methodologies for examining vector suitability and its links to climate. Climate impacts on these other NTDs, whether through environmental changes or societal ones, such as changes in time to diagnosis due to changes in access to health systems, 41 remain largely unexplored.
For thousands of years, societies have been shaped and reshaped by epidemics, with Ebola virus disease, COVID-19 and mpox presenting only the most recent striking examples. A distinct feature of the climate crisis is the pace of change in underlying global ecosystems. This generates uncertainty about the future epidemiology of multiple diseases: not only those that have historically manifested as epidemics, but also those formerly considered as stable and endemic, and those being driven towards elimination or eradication. Climate change will simultaneously reshape the epidemiology of many non-infectious diseases, threaten health infrastructure, affect the health workforce and alter other foundational determinants of human health. These parallel effects will exacerbate the challenge presented by the evolving epidemiology of infectious diseases. 42 , 43
Malaria and many NTDs have relatively complex life cycles, involving overlapping webs of interactions between humans and vertebrate and invertebrate animals. Multiple points of exposure to ecological, biological and social systems increase the probability that climate change will alter disease incidence or prevalence. 43 , 44 This environmental sensitivity makes prediction of future scenarios both difficult and important. The perceived difficulty is borne out in the literature identified here: for most of the outcomes in scope, projections of the effects of future climate change at large scale are scarce. Projections that do exist incorporate considerable uncertainty.
Of all potential outcomes framed for this review, those related to malaria, dengue and chikungunya, and the leishmaniases, were the most studied. Yet even for malaria, long-term projections of future transmission scenarios remain inadequate for robust planning. Beyond the expected short-term effects of extreme weather events on local disease incidence, 45 it is difficult to be definitive 46 –global incidence and attributable deaths may go up, down or stay about the same, depending on multiple factors, including the success or otherwise of nascent vaccination programmes. 6 The arboviral NTDs, dengue and chikungunya, on the other hand, are generally predicted to continue their current surge. 28 , 29 Global expansion in the population at risk of the leishmaniases seems likely. The predicted effect of climate change on these diseases suggests that effects on vectors of other vector-borne NTDs will be similarly important to understand.
For many of the NTDs that we grouped as ‘non-vector-borne’, however, limitations in our capacity for prediction stem in part from gaping deficiencies in our understanding of disease transmission at steady state. Data published only after the searches were conducted for this review pin responsibility for Mycobacterium ulcerans transmission in southeastern Australia on the mosquito Aedes notoscriptus , 47 whereas transmission by mosquitos was previously hypothesised but unproven, and analogous work has not yet been published for Buruli ulcer-endemic areas of the Americas, Japan, Papua New Guinea or West Africa. Postulated mechanisms for the transmission of leprosy are still based on circumstantial evidence. 13 The nematode worm Dracunculus medinensis , the target of a global eradication programme that began in 1980, was only relatively recently discovered to infect paratenic and definitive hosts other than humans. 48 These gaps in our knowledge have lingered because funding for research on NTDs is thin 49 and spread across a very large number of pathogens (Table 1 ), some of which are probably relatively rare. 50 , 51
Multiplying that disease-specific uncertainty are uncertainties surrounding future climate scenarios and their secondary impacts (including, for example, on conflict, migration and demography), which are further clouded by our joint hope that individual and collective behaviours will change sufficiently to allow greenhouse gas concentrations to fall and thereby effectively mitigate climate change. This uncertainty for virtually every parameter of NTD transmission models 52 makes decadal projections of future NTD prevalence or incidence feel ill-advised. Unfortunately, without those projections, NTDs are likely to continue to be given very limited attention in climate-related discussions, including, for example, in the Assessment Reports of the Intergovernmental Panel on Climate Change. 53 Better understanding of NTD transmission dynamics, and estimates—even if heavily caveated—of the potential impacts of climate change, are precisely what is needed now.
The current work builds on previously published reviews, 12 , 43 , 54 but continues to have some limitations. First, although we undertook screening of titles and abstracts by two independent observers, our use of tags to highlight certain characteristics of papers within the Covidence platform may have unmasked some second screeners to the first screener's decision.
Second, screening for mitigation and adaptation strategies was challenging; the process required considerable judgement. It is possible that relevant sources were overlooked in the >42 000 records that our searches identified.
Third, we did not critically quality-appraise the methodology used for each included paper (e.g. number, size and origin of datasets; appropriateness of climate models, such as use of downscaling; and inclusion of all potentially relevant variables). Many studies had low power, did not consider all potential explanatory variables or confounders, or were otherwise methodologically weak. To be useful as a summary of coverage within the published literature, our visualisations imply that all studies contribute equally to the evidence base, whereas they do not.
Fourth, under-ascertainment is an issue for many diseases, but is particularly problematic for malaria and NTDs; their concentration in impoverished populations means that patients with these diseases have HAQIs that are far from ideal. Under-ascertainment is a specifically identified issue for dengue, in which second infections may be considerably more likely to produce disease that leads to clinical presentation and therefore registration. 55
Fifth, to be included in this review, a paper had to explicitly juxtapose climate change and relevant outcomes. Some authors may have made tenuous arguments linking weather-related variables (such as temperature or rainfall) to climate change, resulting in inclusion; others may not have been explicit in framing climate change implications when doing so would have been justifiable.
Sixth, it is likely that source data were used more than once in groups of papers with the same or related outcome measures for overlapping geographies and overlapping timespans.
Seventh, we did not specifically look for the impact of climate change mediated through internal displacement and migration of people; 56 changes in institutional capacity and service provision; 57 , 58 vector microbiome, genetics or gene expression; or pathogen genetics or gene expression. 59 , 60 All of these mechanisms may be important.
Eighth, as for any review, our searches had a fixed date range and were not exhaustive within that range. Not all possible intermediate and reservoir hosts (e.g. for rabies) were specifically included. Insect species with postulated but unproven vectorial capacity were excluded; Culex pipiens is a known vector of lymphatic filariasis in Egypt but papers considering it in other contexts were set aside. Studies in press were excluded by design; we were aware of forthcoming work on the impact of climate change on several diseases that had been submitted for peer review but were not yet published when our searches closed. The December 2023 addition of noma to the WHO's list of NTDs 61 occurred too late for noma to be included in our searches.
Ninth, the high degree of heterogeneity (in questions examined, methods used and so on) precluded quantitative synthesis.
Tenth, projecting disease burdens forward over long timescales means that future changes in treatment and control strategies would ideally also be taken into account. This is difficult to do. Authors of studies from the early part of our 2010–2023 publication window may not have foreseen the scale-up in intervention coverage that has occurred for many diseases in the past 10–15 y.
Despite uncertainty around data, some general conclusions and recommendations are proposed here. It can be inferred from existing data, first, that climate change is likely to have profound direct and indirect implications for malaria, dengue and chikungunya, leishmaniasis and at least several other vector-borne NTDs, even if the amplitude and direction of the effects will probably vary by disease and location, be non-linear 22 , 62–64 and evolve with time. Changes of two kinds will be apparent: diseases will move around, and where endemicity is constant, there will be local increases or decreases in incidence or prevalence. There is a pressing need to safeguard previous global health gains by scaling up proven interventions and achieving impact before future changes render those interventions ineffective. 65 Second, the lack of predictability, even over relatively short timescales, calls for existing surveillance and intervention systems to be reinforced and regularly reviewed. Integrated surveillance and intervention systems, covering multiple diseases 66 and taking a One Health approach, 67 could offer efficiencies. Third, communities should be consulted and involved in these reviews of surveillance and intervention systems, and in research undertaken at the interface of infectious diseases and climate change, to maximise the relevance of such efforts despite changing human populations. Fourth, integrating climate resilience into health systems is critical. This should encompass investing in health infrastructure, fostering cross-sector collaboration, adapting to the needs of displaced populations, improving access to health products and accelerating research and development to fill known gaps. 68 A particular requirement is access to existing and new countermeasures to limit future expansions in disease burden. Fifth, we do not know enough.
Recommendations for future research.
Research to fill current knowledge gaps on the likely impacts of climate change, mitigation and adaptation strategies on malaria and NTDs should:
where projections are modelled, be based on clearly defined climate scenarios, and include multiple scenarios, ideally using the most recent categories defined by the IPCC, in order to facilitate comparisons across studies, diseases and geographies.
where projections are modelled, incorporate not only climate scenarios but also sociodemographic and population density projections. This may require developments in methodology to ensure that demographic transitions underpinning the epidemiological models are in line with those assumed in the projections.
where projections are modelled, ideally incorporate detailed analyses of the likely impact of climate change mitigation and adaptation strategies, which are currently rare, and the modelled effectiveness of existing and new interventions (vector control, vaccines, treatments) under multiple climate scenarios.
explore the potential impacts of climate change on a broader set of NTDs and geographies, prioritising places with the highest disease burdens and the people most vulnerable to the future impacts of climate change.
recognise that, because of the paucity of data, it will remain challenging to estimate the impact of climate change and other secular trends on NTDs, and to anticipate potential interactions between climate change and the impact of interventions. Therefore, new methodologies are needed, based on plausible biological assumptions. This will require distinct study types to prepare for modelling, including prospective population-based investigations, laboratory studies, biological experiments (e.g. mosquito or egg survival) and social science that can be performed in suitable locations to inform projections and provide a data source for future estimates of change. Investigating methods to extrapolate laboratory findings to field settings would be beneficial.
prioritise standardisation and collaboration, including across disciplines. Specifically for modelling, we propose the development and adoption of standardised frameworks for future projections, using, where possible, standardised survey or case data, and an open collaborative model in which source data and contributions to code can be tracked, to speed up and unify research while protecting the rights of countries that generate primary data and acknowledging all collaborators.
facilitate leadership of scientists in affected areas to undertake and communicate research and its implications. An understanding of local context and closer relationships with stakeholders will lead to higher quality analyses with increased uptake in local decision-making processes.
where projections are developed, provide actionable data for policymaking at national and subnational levels. For example, studies focusing on climate-driven dengue expansion to new locations (both in high-income and low- and middle-income countries) should investigate appropriate methods of surveillance, which could be targeted at high-risk areas in a cost-efficient manner. (Risk here could be interpreted in multiple ways: relating to the potential for increases in vector abundance, infection, severe disease or outcomes such as lost gross domestic product (GDP), for example.)
Based on our analysis, we also propose several key recommendations to guide future studies (Box 2 ). The most important of these is for standardisation and collaboration. Our scope included 21 diseases and disease groups, at least 76 distinct pathogens, 373 venomous snakes and humans everywhere; there is insufficient modelling capacity globally for this to be investigated on a competitive basis, particularly when the appropriate modelling methodologies change with the level of endemicity and results are needed at multiple scales. Indeed, our analysis suggests that existing studies may not be sufficiently focused on areas where the need to plan for adaptation may be greatest. We recommend holistic approaches to risk assessment, incorporating more of the available data and recruiting more of the available brainpower to undertake ensemble analyses with agreed best-practice methodology. Modelling efforts should incorporate consideration of humans, pathogens, vectors, intermediate and reservoir hosts and the effect of relevant interventions, as appropriate, to generate predictions over decadal time frames—and not ignore populations where these diseases are currently endemic. Collectively, these measures should reduce potential duplication and hopefully produce more complete and more accurate estimates of future vulnerability, exposure and impact. Open-source collaborative modelling platforms 17 , 23 could facilitate contributions from as many relevant stakeholders as are willing to engage, and allow tailoring of consistently high-quality outputs for specific audiences. Collaboration could include involvement of affected communities through citizen science: in vector surveillance, for example. Accessible global databases on disease and vector occurrence 69–75 should be harnessed and adapted to cover additional diseases. Broader use of remotely sensed climate data should be explored, particularly where locally acquired data are unavailable or microclimates are of relevance. Long-term time-series data should be pooled and re-analysed to tease out the relative contributions of deliberate interventions, secular trends, seasonality and climate change. Production of detailed risk and distribution maps should be facilitated to help plan local control and elimination efforts. The fact that many NTDs are targeted for eradication, interruption of transmission or elimination as a public health problem by 2030 should not dissuade us from taking a long-term view of this work; 43 global health ambitions are not always realised, and the best possible current understanding of counterfactual scenarios should help decision-makers to target resources and chart the most appropriate course.
Conclusions
It is difficult to have immersed ourselves in this literature as we have without acquiring a deepened sense of foreboding over the adverse influence that we as a species are visiting on our planet and its most vulnerable people. Adverse changes have already occurred in the incidence or prevalence of infectious diseases that cause death or profound morbidity. Women, children, older people, indigenous groups and ethnic minorities, migrants and the very poor have contributed least but are likely to experience most of the effects of the climate crisis, 76 notably including through any increase in the burden of malaria or NTDs. An emerging opportunity to correct this inequity arises through financial commitments to NTD control and elimination made at the 28th United Nations Climate Change Conference in December 2023. 77 Allocation of these resources should be guided by informed scenario analyses of current and future disease burden. The work described in this review is a start; convening stakeholders globally to advance the research agenda must be our next collective move.
CLD, TDH, AWS and ISF conceived the overall study design, which was elaborated by PK, JLH, CLD, MA, MB, IB, SD, DLM, CMRT, KRvD, TDH, AWS and ISF, with review by all authors; PK, JLH, CLD, MA, MB, IB, SD, CMRT, KRvD and AWS screened titles and abstracts, located full texts and undertook full-text review; PK and JLH identified seed papers and undertook backward and forward citation searches; PK, JLH, CLD, MA, MB, IB, SD, CMRT, KRvD, JA, AD, DSE, JG, MRJ, AMK, JK, WJK, TLL, JSM, JP, NP, AR, LJR, ZMR, GSGS and AWS extracted data; PK, JLH, KRvD, JFM, CNA, HSR, DRMS and TDH conducted quantitative analyses and constructed figures; PK, KRvD, KCR, CNA and AWS prepared the first draft of the manuscript, which was critically revised by all authors. All authors reviewed and approved the final manuscript. PK and AWS are the paper's guarantors.
We are grateful to Abdisalan M. Noor, lead author of the climate chapter of the World Malaria Report 2023, for his leadership in this space, and to Paul Courtright for assistance with translation from Korean to English.
This work was supported by Reaching the Last Mile; The Fred Hollows Foundation; Bill & Melinda Gates Foundation and the European Commission. PK, SK, CNA and TDH were supported by funding from the Bill & Melinda Gates Foundation [INV-030046], via the NTD Modelling Consortium. TDH is supported by funding from the Li Ka Shing Foundation. JLH acknowledges funding from USAID through an Interagency Agreement with CDC awarded to DLM. KRvD and RL acknowledge funding from the European Union's Horizon Europe research and innovation programme [grant agreement no. 101057554] (Horizon Europe project IDAlert, https://idalertproject.eu ); IDAlert is part of the EU climate change and health cluster ( https://climate-health.eu ). BA, AFG, JK, VL, AR, KHS, RV, SW, AY, DCL, AWS and ISF are staff members of, and CLD and CMRT are consultants to, the WHO. JA was supported by an appointment to the Research Participation Program at the Centers for Disease Control and Prevention, administered by the Oak Ridge Institute for Science and Education through an interagency agreement between the U.S. Department of Energy and the Centers for Disease Control and Prevention. SK, AMK, JSM, MRJ, TLL and GSGS were supported by funding from the Bill & Melinda Gates Foundation [INV-016807]. NMF was supported by funding from Wellcome, the Jameel Institute and the MRC Centre for Global Infectious Disease Analysis. JFM is supported by grant funding from the Bill & Melinda Gates Foundation and Gavi. RSN and KP are staff members of the Pan American Health Organization.
SD is and JC was an employee of The Fred Hollows Foundation; SJB is an employee of the Bill & Melinda Gates Foundation. Their participation in this work was in their personal capacity. All other authors declare no competing interests.
Not required.
All data relevant to the study are available in Supplementary Table 2 and Supplementary Table 3 . Code available upon request.
The authors alone are responsible for the views expressed in this article and they do not necessarily represent the views, decisions or policies of the institutions with which they are affiliated. The boundaries and names shown and the designations used on the maps in this article do not imply the expression of any opinion whatsoever on the part of the authors, or the institutions with which they are affiliated, concerning the legal status of any country, territory, city or area or of its authorities, or concerning the delimitation of its frontiers or boundaries.
Intergovernmental Panel on Climate Change . Summary for Policymakers . In: Masson-Delmotte V , Zhai P , Pirani A et al. (eds). Climate Change 2021: The Physical Science Basis. Contribution of Working Group I to the Sixth Assessment Report of the Intergovernmental Panel on Climate Change. Cambridge and New York : Cambridge University Press , 2021 .
Google Scholar
Google Preview
Romanello M , Napoli CD , Green C et al. The 2023 report of the Lancet Countdown on health and climate change: the imperative for a health-centred response in a world facing irreversible harms . Lancet . 2023 ; 402 ( 10419 ): 2346 – 94 .
Mora C , McKenzie T , Gaw IM et al. Over half of known human pathogenic diseases can be aggravated by climate change . Nat Clim Chang . 2022 ; 12 ( 9 ): 869 – 75 .
Zoleko-Manego R , Okwu DG , Handrich C et al. Effectiveness of antimalarial drug combinations in treating concomitant urogenital schistosomiasis in malaria patients in Lambaréné, Gabon: a non-randomised event-monitoring study . PLoS Negl Trop Dis . 2022 ; 16 ( 10 ): e0010899 .
Afolabi MO , Adebiyi A , Cano J et al. Prevalence and distribution pattern of malaria and soil-transmitted helminth co-endemicity in sub-Saharan Africa, 2000-2018: a geospatial analysis . PLoS Negl Trop Dis . 2022 ; 16 ( 9 ): e0010321 .
World malaria report 2023. Available at https://www.who.int/publications/i/item/9789240086173 (accessed January 26, 2024) . Geneva, Switzerland : World Health Organization , 2023 .
Global report on neglected tropical diseases 2024 . Geneva, Switzerland : World Health Organization , 2024 .
Clark J , Glasziou P , Del Mar C et al. A full systematic review was completed in 2 weeks using automation tools: a case study . J Clin Epidemiol . 2020 ; 121 : 81 – 90 .
Tricco AC , Lillie E , Zarin W et al. PRISMA Extension for Scoping Reviews (PRISMA-ScR): checklist and Explanation . Ann Intern Med . 2018 ; 169 ( 7 ): 467 – 73 .
Clark JM , Sanders S , Carter M et al. Improving the translation of search strategies using the Polyglot Search Translator: a randomized controlled trial . J Med Libr Assoc . 2020 ; 108 ( 2 ): 195 – 207 .
Haddaway NR , Grainger MJ , Gray CT. citationchaser: an R package and Shiny app for forward and backward citations chasing in academic searching. Available at https://zenodo.org/records/4543513 (accessed December 12, 2023 ). Zenodo . 2021 .
Tidman R , Abela-Ridder B , de Castañeda RR. The impact of climate change on neglected tropical diseases: a systematic review . Trans R Soc Trop Med Hyg . 2021 ; 115 ( 2 ): 147 – 68 .
Ending the neglect to attain the Sustainable Development Goals: a road map for neglected tropical diseases 2021–2030. Available at https://apps.who.int/iris/handle/10665/338565 (accessed January 28, 2021 ). Geneva, Switzerland : World Health Organization , 2020 .
GBD 2019 Diseases and Injuries Collaborators . Global burden of 369 diseases and injuries in 204 countries and territories, 1990-2019: a systematic analysis for the Global Burden of Disease Study 2019 . Lancet . 2020 ; 396 ( 10258 ): 1204 – 22 .
Haakenstad A , Yearwood JA , Fullman N et al. Assessing performance of the Healthcare Access and Quality Index, overall and by select age groups, for 204 countries and territories, 1990-2019: a systematic analysis from the Global Burden of Disease Study . 2019 . Lancet Glob Health . 2022 ; 10 ( 12 ): e1715 – e43 .
Ermert V , Fink AH , Morse AP et al. The impact of regional climate change on malaria risk due to greenhouse forcing and land-use changes in tropical Africa . Environ Health Perspect . 2012 ; 120 ( 1 ): 77 – 84 .
Colón-González FJ , Sewe MO , Tompkins AM et al. Projecting the risk of mosquito-borne diseases in a warmer and more populated world: a multi-model, multi-scenario intercomparison modelling study . Lancet Planet Health . 2021 ; 5 ( 7 ): e404 – e14 .
Caminade C , Kovats S , Rocklov J et al. Impact of climate change on global malaria distribution . Proc Natl Acad Sci USA . 2014 ; 111 ( 9 ): 3286 – 91 .
Feachem RGA , Chen I , Akbari O et al. Malaria eradication within a generation: ambitious, achievable, and necessary . Lancet . 2019 ; 394 ( 10203 ): 1056 – 112 .
Rodó X , Martinez PP , Siraj A et al. Malaria trends in Ethiopian highlands track the 2000 ‘slowdown’ in global warming . Nat Commun . 2021 ; 12 ( 1 ): 1555 .
Stern DI , Gething PW , Kabaria CW et al. Temperature and malaria trends in highland East Africa . PLoS One . 2011 ; 6 ( 9 ): e24524 .
Alonso D , Bouma MJ , Pascual M. Epidemic malaria and warmer temperatures in recent decades in an East African highland . Proc Biol Sci . 2011 ; 278 ( 1712 ): 1661 – 9 .
Edlund S , Davis M , Douglas JV et al. A global model of malaria climate sensitivity: comparing malaria response to historic climate data based on simulation and officially reported malaria incidence . Malar J . 2012 ; 11 : 331 .
Tabassum S , Kalsoom T , Zaheer Z et al. Reflections on the surge in malaria cases after unprecedented flooding in Pakistan-A commentary . Health Sci Rep . 2023 ; 6 ( 10 ): e1620 .
Georgiades P , Proestos Y , Lelieveld J et al. Machine Learning Modeling of Aedes albopictus Habitat Suitability in the 21st Century . Insects . 2023 ; 14 ( 5 ): 447 .
Campbell LP , Luther C , Moo-Llanes D et al. Climate change influences on global distributions of dengue and chikungunya virus vectors . Philos Trans R Soc Lond B Biol Sci . 2015 ; 370 ( 1665 ): 20140135 .
Liu H , Huang X , Guo X et al. Climate change and Aedes albopictus risks in China: current impact and future projection . Infect Dis Poverty . 2023 ; 12 ( 1 ): 26 .
Messina JP , Brady OJ , Golding N et al. The current and future global distribution and population at risk of dengue . Nat Microbiol . 2019 ; 4 ( 9 ): 1508 – 15 .
Schaffner F , Mathis A. Dengue and dengue vectors in the WHO European region: past, present, and scenarios for the future . Lancet Infect Dis . 2014 ; 14 ( 12 ): 1271 – 80 .
Colón-González FJ , Harris I , Osborn TJ et al. Limiting global-mean temperature increase to 1.5-2°C could reduce the incidence and spatial spread of dengue fever in Latin America . Proc Natl Acad Sci USA . 2018 ; 115 ( 24 ): 6243 – 8 .
Escobar LE , Romero-Alvarez D , Leon R et al. Declining Prevalence of Disease Vectors Under Climate Change . Sci Rep . 2016 ; 6 : 39150 .
Bouzid M , Colón-González FJ , Lung T et al. Climate change and the emergence of vector-borne diseases in Europe: case study of dengue fever . BMC Public Health . 2014 ; 14 : 781 .
Chalghaf B , Chemkhi J , Mayala B et al. Ecological niche modeling predicting the potential distribution of Leishmania vectors in the Mediterranean basin: impact of climate change . Parasit Vectors . 2018 ; 11 ( 1 ): 461 .
Moo-Llanes DA , Pech-May A , Ibarra-Cerdeña CN et al. Inferring distributional shifts of epidemiologically important North and Central American sandflies from Pleistocene to future scenarios . Med Vet Entomol . 2019 ; 33 ( 1 ): 31 – 43 .
Moo-Llanes D , Ibarra-Cerdeña CN , Rebollar-Téllez EA et al. Current and future niche of North and Central American sand flies (Diptera: psychodidae) in climate change scenarios . PLoS Negl Trop Dis . 2013 ; 7 ( 9 ): e2421 .
Peterson AT , Campbell LP , Moo-Llanes DA et al. Influences of climate change on the potential distribution of Lutzomyia longipalpis sensu lato (Psychodidae: phlebotominae) . Int J Parasitol . 2017 ; 47 ( 10-11 ): 667 – 74 .
González C , Wang O , Strutz SE et al. Climate change and risk of leishmaniasis in North America: predictions from ecological niche models of vector and reservoir species . PLoS Negl Trop Dis . 2010 ; 4 ( 1 ): e585 .
McCreesh N , Nikulin G , Booth M . Predicting the effects of climate change on Schistosoma mansoni transmission in eastern Africa . Parasit Vectors . 2015 ; 8 : 4 .
Cao B , Bai C , Wu K et al. Tracing the future of epidemics: coincident niche distribution of host animals and disease incidence revealed climate-correlated risk shifts of main zoonotic diseases in China . Glob Chang Biol . 2023 ; 29 ( 13 ): 3723 – 46 .
Zhu G , Fan J , Peterson AT . Schistosoma japonicum transmission risk maps at present and under climate change in mainland China . PLoS Negl Trop Dis . 2017 ; 11 ( 10 ): e0006021 .
Impact of the COVID-19 pandemic on seven neglected tropical diseases: a model-based analysis ( https://www.who.int/publications/i/item/9789240027671 , accessed 10 March 2024 ). Geneva, Switzerland : World Health Organization , 2021 .
Watts N , Amann M , Arnell N et al. The 2020 report of The Lancet Countdown on health and climate change: responding to converging crises . Lancet . 2021 ; 397 ( 10269 ): 129 – 70 .
Booth M. Climate Change and the Neglected Tropical Diseases . Adv Parasitol . 2018 ; 100 : 39 – 126 .
de Souza WM , Weaver SC. Effects of climate change and human activities on vector-borne diseases . Nat Rev Micro . 2024 . https://pubmed.ncbi.nlm.nih.gov/38486116/
Otto FEL , Zachariah M , Saeed F et al. Climate change increased extreme monsoon rainfall, flooding highly vulnerable communities in Pakistan . Environ Res Clim . 2023 ; 2 ( 2 ): 025001 .
Tompkins AM , Thomson MC. Uncertainty in malaria simulations in the highlands of Kenya: relative contributions of model parameter setting, driving climate and initial condition errors . PLoS One . 2018 ; 13 ( 9 ): e0200638 .
Mee PT , Buultjens AH , Oliver J et al. Mosquitoes provide a transmission route between possums and human s for Buruli ulcer in southeastern Australia . Nat Microbiol . 2024 ; 9 ( 2 ): 377–89 .
Callaway E. Dogs thwart effort to eradicate Guinea worm . Nature . 2016 ; 529 ( 7584 ): 10 – 1 .
G-FINDER 2023 . Neglected disease research and development: the higher cost of lower funding . Sydney : Policy Cures Research , 2024 .
Stamm LV . Pinta: Latin America's Forgotten Disease? Am J Trop Med Hyg . 2015 ; 93 ( 5 ): 901 – 3 .
Vighi da Rosa R , Damares Rodrigues de Souza D , Cartell A et al. Mal de Pinta, first autochthonous case from South of Brazil . Int J Dermatol . 2021 ; 60 ( 1 ): e19 – 21 .
Moore JL , Liang S , Akullian A et al. Cautioning the use of degree-day models for climate change projections in the presence of parametric uncertainty . Ecol Appl . 2012 ; 22 ( 8 ): 2237 – 47 .
McIver L , Beavon E , Malm A et al. Impacts of climate change on human health in humanitarian settings: Evidence gaps and future research needs . PLoS Climate . 2024 ; 3 ( 3 ): e0000243
Kulkarni MA , Duguay C , Ost K . Charting the evidence for climate change impacts on the global spread of malaria and dengue and adaptive responses: a scoping review of reviews . Global Health . 2022 ; 18 ( 1 ): 1 .
Clapham HE , Cummings DAT , Johansson MA . Immune status alters the probability of apparent illness due to dengue virus infection: evidence from a pooled analysis across multiple cohort and cluster studies . PLoS Negl Trop Dis . 2017 ; 11 ( 9 ): e0005926 .
Guerra CA , Kang SY , Citron DT et al. Human mobility patterns and malaria importation on Bioko Island . Nat Commun . 2019 ; 10 ( 1 ): 2332 .
Rugai D , Kassenga GR . Climate change impacts and institutional response capacity in Dar es Salaam, Tanzania . In: Macchi S , Ms Tiepolo (eds). Climate Change Vulnerability in Southern African Cities . Cham, Switzerland : Springer , 2014 , 39 – 55 .
Shirley H , Grifferty G , Yates EF et al. The connection between climate change, surgical care and neglected tropical diseases . Ann Glob Health . 2022 ; 88 ( 1 ): 68 .
Greischar MA , Beck-Johnson LM , Mideo N. Partitioning the influence of ecology across scales on parasite evolution . Evolution . 2019 ; 73 ( 11 ): 2175 – 88 .
Wimalasiri-Yapa B , Barrero RA , Stassen L et al. Temperature modulates immune gene expression in mosquitoes during arbovirus infection . Open Biol . 2021 ; 11 ( 1 ): 200246 .
WHO officially recognizes noma as a neglected tropical disease ( https://www.who.int/news/item/15-12-2023-who-officially-recognizes-noma-as-a-neglected-tropical-disease , accessed 25 January 2024 ). Geneva, Switzerland : World Health Organization , 2023 .
Chaves LF , Hashizume M , Satake A et al. Regime shifts and heterogeneous trends in malaria time series from Western Kenya Highlands . Parasitology . 2012 ; 139 ( 1 ): 14 – 25 .
Paaijmans KP , Wandago MO , Githeko AK et al. Unexpected high losses of Anopheles gambiae larvae due to rainfall . PLoS One . 2007 ; 2 ( 11 ): e1146 .
Chemison A , Ramstein G , Tompkins AM et al. Impact of an accelerated melting of Greenland on malaria distribution over Africa . Nat Commun . 2021 ; 12 ( 1 ): 3971 .
Global health community calls for urgent action on climate and health at COP28. Available at https://www.who.int/news/item/27-11-2023-global-health-community-calls-for-urgent-action-on-climate-and-health-at-cop28 (accessed February 21, 2024 ). Geneva, Switzerland : World Health Organization , 2023 .
Cooley GM , Feldstein LR , Bennett SD et al. No serological evidence of trachoma or yaws among residents of registered camps and makeshift settlements in Cox's Bazar, Bangladesh . Am J Trop Med Hyg . 2021 ; 104 ( 6 ): 2031 – 7 .
Ending the neglect to attain the sustainable development goals. One health: approach for action against neglected tropical diseases 2021-2030. Available at https://www.who.int/publications/i/item/9789240042414 (accessed March 21, 2024 ). Geneva, Switzerland : World Health Organization , 2022 .
Mabey D , Agler E , Amuasi JH et al. Towards a comprehensive research and development plan to support the control, elimination and eradication of neglected tropical diseases . Trans R Soc Trop Med Hyg . 2020 ; 115 ( 2 ): 196 – 9 .
Hay SI , Snow RW . The malaria Atlas Project: developing global maps of malaria risk . PLoS Med . 2006 ; 3 ( 12 ): e473 .
International Trachoma Initiative. Global Atlas of Trachoma. Available at www.trachomaatlas.org (accessed February 21, 2024 ). Decatur : Task Force for Global Health , 2024 .
Brooker S , Hotez PJ , Bundy DA. The global atlas of helminth infection: mapping the way forward in neglected tropical disease control . PLoS Negl Trop Dis . 2010 ; 4 ( 7 ): e779 .
Simarro PP , Cecchi G , Paone M et al. The Atlas of human African trypanosomiasis: a contribution to global mapping of neglected tropical diseases . Int J Health Geogr . 2010 ; 9 : 57 .
Pigott DM , Golding N , Messina JP et al. Global database of leishmaniasis occurrence locations, 1960-2012 . Sci Data . 2014 ; 1 : 140036 .
Clarke J , Lim A , Gupte PR et al. OpenDengue: data from the OpenDengue database. Version [1.2]. figshare. Dataset. Available at https://doi.org/10.6084/m9.figshare.24259573.v3 (accessed February 21, 2024 ). London, UK : London School of Hygiene & Tropical Medicine , 2023 .
Malaria threats map . Available at https://apps.who.int/malaria/maps/threats/ (accessed February 29, 2024 ). Geneva, Switzerland : World Health Organization , 2024 .
Lee H , Calvin K , Dasgupta D et al. Climate change 2023: synthesis report. Contribution of working groups I, II and III to the sixth assessment report of the intergovernmental panel on climate change . Available at https://www.ipcc.ch/report/ar6/syr/downloads/report/IPCC_AR6_SYR_LongerReport.pdf (accessed January 23, 2024 ). Geneva, Switzerland : Intergovernmental Panel on Climate Change , 2023 .
Venkatesan P. Millions of dollars pledged at COP28 to fight NTDs . Lancet Infec Dis . 2024 ; 24 ( 2 ): e88 .
Hougton JT , Callander BA , Varney SK. Climate change 1992: the Supplementary Report to the IPCC Scientific Assessment . Cambridge : Cambridge University Press , 1992 .
Intergovernmental Panel on Climate Change . Summary for Policymakers: Emissions Scenarios. A Special Report of IPCC Working Group III . Geneva : IPCC , 2000 .
Intergovernmental Panel on Climate Change . Summary for policymakers . In: Pörtner H-O , Roberts DCV , Masson-Delmotte V et al. (eds). IPCC Special Report on the Ocean and Cryosphere in a Changing Climate Geneva : IPCC , 2019 .
Rogelj J , Meinshausen M , Knutti R. Global warming under old and new scenarios using IPCC climate sensitivity range estimates . Nat Clim Change . 2012 ; 2 ( 4 ): 248 – 53 .
Intergovernmental Panel on Climate Change . Climate Change 2021: the Physical Science Basis. Contribution of Working Group I to the Sixth Assessment Report of the Intergovernmental Panel on Climate Change [ Masson-Delmotte V. , Zhai P. , Pirani A. , Connors S.L. , Péan C. , Berger S. , Caud N. , Chen Y. , Goldfarb L. , Gomis M.I. , Huang M. , Leitzell K. , Lonnoy E. , Matthews J.B.R. , Maycock T.K. , Waterfield T. , Yelekçi O. , Yu R. , Zhou B. (eds.)]. Cambridge and New York : Cambridge University Press , 2021 .
Intergovernmental Panel on Climate Change . Climate Change 2023: Synthesis Report. Contribution of Working Groups I, II and III to the Sixth Assessment Report of the Intergovernmental Panel on Climate Change [ Core Writing Team , Lee H. , Romero J. (eds.)]. Geneva : IPCC , 2023 .
- chikungunya fever
- dengue fever
- cost of illness
- leishmaniasis
- tropical disease
- climate change
Supplementary data
Email alerts, citing articles via.
- About Transactions of the Royal Society of Tropical Medicine and Hygiene
- Recommend to your Library
Affiliations
- Online ISSN 1878-3503
- Print ISSN 0035-9203
- Copyright © 2024 Royal Society of Tropical Medicine and Hygiene
- About Oxford Academic
- Publish journals with us
- University press partners
- What we publish
- New features
- Open access
- Institutional account management
- Rights and permissions
- Get help with access
- Accessibility
- Advertising
- Media enquiries
- Oxford University Press
- Oxford Languages
- University of Oxford
Oxford University Press is a department of the University of Oxford. It furthers the University's objective of excellence in research, scholarship, and education by publishing worldwide
- Copyright © 2024 Oxford University Press
- Cookie settings
- Cookie policy
- Privacy policy
- Legal notice
This Feature Is Available To Subscribers Only
Sign In or Create an Account
This PDF is available to Subscribers Only
For full access to this pdf, sign in to an existing account, or purchase an annual subscription.
Thank you for visiting nature.com. You are using a browser version with limited support for CSS. To obtain the best experience, we recommend you use a more up to date browser (or turn off compatibility mode in Internet Explorer). In the meantime, to ensure continued support, we are displaying the site without styles and JavaScript.
- View all journals
- Explore content
- About the journal
- Publish with us
- Sign up for alerts
- Published: 08 May 2024

A meta-analysis on global change drivers and the risk of infectious disease
- Michael B. Mahon ORCID: orcid.org/0000-0002-9436-2998 1 , 2 na1 ,
- Alexandra Sack 1 , 3 na1 ,
- O. Alejandro Aleuy 1 ,
- Carly Barbera 1 ,
- Ethan Brown ORCID: orcid.org/0000-0003-0827-4906 1 ,
- Heather Buelow ORCID: orcid.org/0000-0003-3535-4151 1 ,
- David J. Civitello 4 ,
- Jeremy M. Cohen ORCID: orcid.org/0000-0001-9611-9150 5 ,
- Luz A. de Wit ORCID: orcid.org/0000-0002-3045-4017 1 ,
- Meghan Forstchen 1 , 3 ,
- Fletcher W. Halliday 6 ,
- Patrick Heffernan 1 ,
- Sarah A. Knutie 7 ,
- Alexis Korotasz 1 ,
- Joanna G. Larson ORCID: orcid.org/0000-0002-1401-7837 1 ,
- Samantha L. Rumschlag ORCID: orcid.org/0000-0003-3125-8402 1 , 2 ,
- Emily Selland ORCID: orcid.org/0000-0002-4527-297X 1 , 3 ,
- Alexander Shepack 1 ,
- Nitin Vincent ORCID: orcid.org/0000-0002-8593-1116 1 &
- Jason R. Rohr ORCID: orcid.org/0000-0001-8285-4912 1 , 2 , 3 na1
Nature ( 2024 ) Cite this article
6499 Accesses
607 Altmetric
Metrics details
- Infectious diseases
Anthropogenic change is contributing to the rise in emerging infectious diseases, which are significantly correlated with socioeconomic, environmental and ecological factors 1 . Studies have shown that infectious disease risk is modified by changes to biodiversity 2 , 3 , 4 , 5 , 6 , climate change 7 , 8 , 9 , 10 , 11 , chemical pollution 12 , 13 , 14 , landscape transformations 15 , 16 , 17 , 18 , 19 , 20 and species introductions 21 . However, it remains unclear which global change drivers most increase disease and under what contexts. Here we amassed a dataset from the literature that contains 2,938 observations of infectious disease responses to global change drivers across 1,497 host–parasite combinations, including plant, animal and human hosts. We found that biodiversity loss, chemical pollution, climate change and introduced species are associated with increases in disease-related end points or harm, whereas urbanization is associated with decreases in disease end points. Natural biodiversity gradients, deforestation and forest fragmentation are comparatively unimportant or idiosyncratic as drivers of disease. Overall, these results are consistent across human and non-human diseases. Nevertheless, context-dependent effects of the global change drivers on disease were found to be common. The findings uncovered by this meta-analysis should help target disease management and surveillance efforts towards global change drivers that increase disease. Specifically, reducing greenhouse gas emissions, managing ecosystem health, and preventing biological invasions and biodiversity loss could help to reduce the burden of plant, animal and human diseases, especially when coupled with improvements to social and economic determinants of health.
This is a preview of subscription content, access via your institution
Access options
Access Nature and 54 other Nature Portfolio journals
Get Nature+, our best-value online-access subscription
$29.99 / 30 days
cancel any time
Subscribe to this journal
Receive 51 print issues and online access
$199.00 per year
only $3.90 per issue
Buy this article
- Purchase on Springer Link
- Instant access to full article PDF
Prices may be subject to local taxes which are calculated during checkout
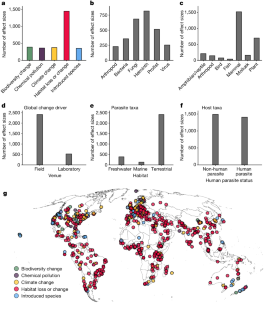
Similar content being viewed by others
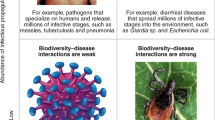
Towards common ground in the biodiversity–disease debate
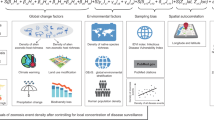
Biological invasions facilitate zoonotic disease emergences
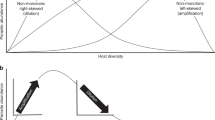
Measuring the shape of the biodiversity-disease relationship across systems reveals new findings and key gaps
Data availability.
All the data for this Article have been deposited at Zenodo ( https://doi.org/10.5281/zenodo.8169979 ) 52 and GitHub ( https://github.com/mahonmb/GCDofDisease ) 53 .
Code availability
All the code for this Article has been deposited at Zenodo ( https://doi.org/10.5281/zenodo.8169979 ) 52 and GitHub ( https://github.com/mahonmb/GCDofDisease ) 53 . R markdown is provided in Supplementary Data 1 .
Jones, K. E. et al. Global trends in emerging infectious diseases. Nature 451 , 990–994 (2008).
Article ADS CAS PubMed PubMed Central Google Scholar
Civitello, D. J. et al. Biodiversity inhibits parasites: broad evidence for the dilution effect. Proc. Natl Acad. Sci USA 112 , 8667–8671 (2015).
Halliday, F. W., Rohr, J. R. & Laine, A.-L. Biodiversity loss underlies the dilution effect of biodiversity. Ecol. Lett. 23 , 1611–1622 (2020).
Article PubMed PubMed Central Google Scholar
Rohr, J. R. et al. Towards common ground in the biodiversity–disease debate. Nat. Ecol. Evol. 4 , 24–33 (2020).
Article PubMed Google Scholar
Johnson, P. T. J., Ostfeld, R. S. & Keesing, F. Frontiers in research on biodiversity and disease. Ecol. Lett. 18 , 1119–1133 (2015).
Keesing, F. et al. Impacts of biodiversity on the emergence and transmission of infectious diseases. Nature 468 , 647–652 (2010).
Cohen, J. M., Sauer, E. L., Santiago, O., Spencer, S. & Rohr, J. R. Divergent impacts of warming weather on wildlife disease risk across climates. Science 370 , eabb1702 (2020).
Article CAS PubMed PubMed Central Google Scholar
Rohr, J. R. et al. Frontiers in climate change-disease research. Trends Ecol. Evol. 26 , 270–277 (2011).
Altizer, S., Ostfeld, R. S., Johnson, P. T. J., Kutz, S. & Harvell, C. D. Climate change and infectious diseases: from evidence to a predictive framework. Science 341 , 514–519 (2013).
Article ADS CAS PubMed Google Scholar
Rohr, J. R. & Cohen, J. M. Understanding how temperature shifts could impact infectious disease. PLoS Biol. 18 , e3000938 (2020).
Carlson, C. J. et al. Climate change increases cross-species viral transmission risk. Nature 607 , 555–562 (2022).
Halstead, N. T. et al. Agrochemicals increase risk of human schistosomiasis by supporting higher densities of intermediate hosts. Nat. Commun. 9 , 837 (2018).
Article ADS PubMed PubMed Central Google Scholar
Martin, L. B., Hopkins, W. A., Mydlarz, L. D. & Rohr, J. R. The effects of anthropogenic global changes on immune functions and disease resistance. Ann. N. Y. Acad. Sci. 1195 , 129–148 (2010).
Rumschlag, S. L. et al. Effects of pesticides on exposure and susceptibility to parasites can be generalised to pesticide class and type in aquatic communities. Ecol. Lett. 22 , 962–972 (2019).
Allan, B. F., Keesing, F. & Ostfeld, R. S. Effect of forest fragmentation on Lyme disease risk. Conserv. Biol. 17 , 267–272 (2003).
Article Google Scholar
Brearley, G. et al. Wildlife disease prevalence in human‐modified landscapes. Biol. Rev. 88 , 427–442 (2013).
Rohr, J. R. et al. Emerging human infectious diseases and the links to global food production. Nat. Sustain. 2 , 445–456 (2019).
Bradley, C. A. & Altizer, S. Urbanization and the ecology of wildlife diseases. Trends Ecol. Evol. 22 , 95–102 (2007).
Allen, T. et al. Global hotspots and correlates of emerging zoonotic diseases. Nat. Commun. 8 , 1124 (2017).
Sokolow, S. H. et al. Ecological and socioeconomic factors associated with the human burden of environmentally mediated pathogens: a global analysis. Lancet Planet. Health 6 , e870–e879 (2022).
Young, H. S., Parker, I. M., Gilbert, G. S., Guerra, A. S. & Nunn, C. L. Introduced species, disease ecology, and biodiversity–disease relationships. Trends Ecol. Evol. 32 , 41–54 (2017).
Barouki, R. et al. The COVID-19 pandemic and global environmental change: emerging research needs. Environ. Int. 146 , 106272 (2021).
Article CAS PubMed Google Scholar
Nova, N., Athni, T. S., Childs, M. L., Mandle, L. & Mordecai, E. A. Global change and emerging infectious diseases. Ann. Rev. Resour. Econ. 14 , 333–354 (2021).
Zhang, L. et al. Biological invasions facilitate zoonotic disease emergences. Nat. Commun. 13 , 1762 (2022).
Olival, K. J. et al. Host and viral traits predict zoonotic spillover from mammals. Nature 546 , 646–650 (2017).
Guth, S. et al. Bats host the most virulent—but not the most dangerous—zoonotic viruses. Proc. Natl Acad. Sci. USA 119 , e2113628119 (2022).
Nelson, G. C. et al. in Ecosystems and Human Well-Being (Millennium Ecosystem Assessment) Vol. 2 (eds Rola, A. et al) Ch. 7, 172–222 (Island Press, 2005).
Read, A. F., Graham, A. L. & Raberg, L. Animal defenses against infectious agents: is damage control more important than pathogen control? PLoS Biol. 6 , 2638–2641 (2008).
Article CAS Google Scholar
Medzhitov, R., Schneider, D. S. & Soares, M. P. Disease tolerance as a defense strategy. Science 335 , 936–941 (2012).
Torchin, M. E. & Mitchell, C. E. Parasites, pathogens, and invasions by plants and animals. Front. Ecol. Environ. 2 , 183–190 (2004).
Bellay, S., de Oliveira, E. F., Almeida-Neto, M. & Takemoto, R. M. Ectoparasites are more vulnerable to host extinction than co-occurring endoparasites: evidence from metazoan parasites of freshwater and marine fishes. Hydrobiologia 847 , 2873–2882 (2020).
Scheffer, M. Critical Transitions in Nature and Society Vol. 16 (Princeton Univ. Press, 2020).
Rohr, J. R. et al. A planetary health innovation for disease, food and water challenges in Africa. Nature 619 , 782–787 (2023).
Reaser, J. K., Witt, A., Tabor, G. M., Hudson, P. J. & Plowright, R. K. Ecological countermeasures for preventing zoonotic disease outbreaks: when ecological restoration is a human health imperative. Restor. Ecol. 29 , e13357 (2021).
Hopkins, S. R. et al. Evidence gaps and diversity among potential win–win solutions for conservation and human infectious disease control. Lancet Planet. Health 6 , e694–e705 (2022).
Mitchell, C. E. & Power, A. G. Release of invasive plants from fungal and viral pathogens. Nature 421 , 625–627 (2003).
Chamberlain, S. A. & Szöcs, E. taxize: taxonomic search and retrieval in R. F1000Research 2 , 191 (2013).
Newman, M. Fundamentals of Ecotoxicology (CRC Press/Taylor & Francis Group, 2010).
Rohatgi, A. WebPlotDigitizer v.4.5 (2021); automeris.io/WebPlotDigitizer .
Lüdecke, D. esc: effect size computation for meta analysis (version 0.5.1). Zenodo https://doi.org/10.5281/zenodo.1249218 (2019).
Lipsey, M. W. & Wilson, D. B. Practical Meta-Analysis (SAGE, 2001).
R Core Team. R: A Language and Environment for Statistical Computing Vol. 2022 (R Foundation for Statistical Computing, 2020); www.R-project.org/ .
Viechtbauer, W. Conducting meta-analyses in R with the metafor package. J. Stat. Softw. 36 , 1–48 (2010).
Pustejovsky, J. E. & Tipton, E. Meta-analysis with robust variance estimation: Expanding the range of working models. Prev. Sci. 23 , 425–438 (2022).
Lenth, R. emmeans: estimated marginal means, aka least-squares means. R package v.1.5.1 (2020).
Bartoń, K. MuMIn: multi-modal inference. Model selection and model averaging based on information criteria (AICc and alike) (2019).
Burnham, K. P. & Anderson, D. R. Multimodel inference: understanding AIC and BIC in model selection. Sociol. Methods Res. 33 , 261–304 (2004).
Article MathSciNet Google Scholar
Marks‐Anglin, A. & Chen, Y. A historical review of publication bias. Res. Synth. Methods 11 , 725–742 (2020).
Nakagawa, S. et al. Methods for testing publication bias in ecological and evolutionary meta‐analyses. Methods Ecol. Evol. 13 , 4–21 (2022).
Gurevitch, J., Koricheva, J., Nakagawa, S. & Stewart, G. Meta-analysis and the science of research synthesis. Nature 555 , 175–182 (2018).
Bates, D., Mächler, M., Bolker, B. & Walker, S. Fitting linear mixed-effects models using lme4. J. Stat. Softw. 67 , 1–48 (2015).
Mahon, M. B. et al. Data and code for ‘A meta-analysis on global change drivers and the risk of infectious disease’. Zenodo https://doi.org/10.5281/zenodo.8169979 (2024).
Mahon, M. B. et al. Data and code for ‘A meta-analysis on global change drivers and the risk of infectious disease’. GitHub github.com/mahonmb/GCDofDisease (2024).
Download references
Acknowledgements
We thank C. Mitchell for contributing data on enemy release; L. Albert and B. Shayhorn for assisting with data collection; J. Gurevitch, M. Lajeunesse and G. Stewart for providing comments on an earlier version of this manuscript; and C. Carlson and two anonymous reviewers for improving this paper. This research was supported by grants from the National Science Foundation (DEB-2109293, DEB-2017785, DEB-1518681, IOS-1754868), National Institutes of Health (R01TW010286) and US Department of Agriculture (2021-38420-34065) to J.R.R.; a US Geological Survey Powell grant to J.R.R. and S.L.R.; University of Connecticut Start-up funds to S.A.K.; grants from the National Science Foundation (IOS-1755002) and National Institutes of Health (R01 AI150774) to D.J.C.; and an Ambizione grant (PZ00P3_202027) from the Swiss National Science Foundation to F.W.H. The funders had no role in study design, data collection and analysis, decision to publish or preparation of the manuscript.
Author information
These authors contributed equally: Michael B. Mahon, Alexandra Sack, Jason R. Rohr
Authors and Affiliations
Department of Biological Sciences, University of Notre Dame, Notre Dame, IN, USA
Michael B. Mahon, Alexandra Sack, O. Alejandro Aleuy, Carly Barbera, Ethan Brown, Heather Buelow, Luz A. de Wit, Meghan Forstchen, Patrick Heffernan, Alexis Korotasz, Joanna G. Larson, Samantha L. Rumschlag, Emily Selland, Alexander Shepack, Nitin Vincent & Jason R. Rohr
Environmental Change Initiative, University of Notre Dame, Notre Dame, IN, USA
Michael B. Mahon, Samantha L. Rumschlag & Jason R. Rohr
Eck Institute of Global Health, University of Notre Dame, Notre Dame, IN, USA
Alexandra Sack, Meghan Forstchen, Emily Selland & Jason R. Rohr
Department of Biology, Emory University, Atlanta, GA, USA
David J. Civitello
Department of Ecology and Evolutionary Biology, Yale University, New Haven, CT, USA
Jeremy M. Cohen
Department of Botany and Plant Pathology, Oregon State University, Corvallis, OR, USA
Fletcher W. Halliday
Department of Ecology and Evolutionary Biology, Institute for Systems Genomics, University of Connecticut, Storrs, CT, USA
Sarah A. Knutie
You can also search for this author in PubMed Google Scholar
Contributions
J.R.R. conceptualized the study. All of the authors contributed to the methodology. All of the authors contributed to investigation. Visualization was performed by M.B.M. The initial study list and related information were compiled by D.J.C., J.M.C., F.W.H., S.A.K., S.L.R. and J.R.R. Data extraction was performed by M.B.M., A.S., O.A.A., C.B., E.B., H.B., L.A.d.W., M.F., P.H., A.K., J.G.L., E.S., A.S. and N.V. Data were checked for accuracy by M.B.M. and A.S. Analyses were performed by M.B.M. and J.R.R. Funding was acquired by D.J.C., J.R.R., S.A.K. and S.L.R. Project administration was done by J.R.R. J.R.R. supervised the study. J.R.R. and M.B.M. wrote the original draft. All of the authors reviewed and edited the manuscript. J.R.R. and M.B.M. responded to reviewers.
Corresponding author
Correspondence to Jason R. Rohr .
Ethics declarations
Competing interests.
The authors declare no competing interests.
Peer review
Peer review information.
Nature thanks Colin Carlson and the other, anonymous, reviewer(s) for their contribution to the peer review of this work. Peer reviewer reports are available.
Additional information
Publisher’s note Springer Nature remains neutral with regard to jurisdictional claims in published maps and institutional affiliations.
Extended data figures and tables
Extended data fig. 1 prisma flowchart..
The PRISMA flow diagram of the search and selection of studies included in this meta-analysis. Note that 77 studies came from the Halliday et al. 3 database on biodiversity change.
Extended Data Fig. 2 Summary of the number of studies (A-F) and parasite taxa (G-L) in the infectious disease database across ecological contexts.
The contexts are global change driver ( A , G ), parasite taxa ( B , H ), host taxa ( C , I ), experimental venue ( D , J ), study habitat ( E , K ), and human parasite status ( F , L ).
Extended Data Fig. 3 Summary of the number of effect sizes (A-I), studies (J-R), and parasite taxa (S-a) in the infectious disease database for various parasite and host contexts.
Shown are parasite type ( A , J , S ), host thermy ( B , K , T ), vector status ( C , L , U ), vector-borne status ( D , M , V ), parasite transmission ( E , N , W ), free living stages ( F , O , X ), host (e.g. disease, host growth, host survival) or parasite (e.g. parasite abundance, prevalence, fecundity) endpoint ( G , P , Y ), micro- vs macroparasite ( H , Q , Z ), and zoonotic status ( I , R , a ).
Extended Data Fig. 4 The effects of global change drivers and subsequent subcategories on disease responses with Log Response Ratio instead of Hedge’s g.
Here, Log Response Ratio shows similar trends to that of Hedge’s g presented in the main text. The displayed points represent the mean predicted values (with 95% confidence intervals) from a meta-analytical model with separate random intercepts for study. Points that do not share letters are significantly different from one another (p < 0.05) based on a two-sided Tukey’s posthoc multiple comparison test with adjustment for multiple comparisons. See Table S 3 for pairwise comparison results. Effects of the five common global change drivers ( A ) have the same directionality, similar magnitude, and significance as those presented in Fig. 2 . Global change driver effects are significant when confidence intervals do not overlap with zero and explicitly tested with two-tailed t-test (indicated by asterisks; t 80.62 = 2.16, p = 0.034 for CP; t 71.42 = 2.10, p = 0.039 for CC; t 131.79 = −3.52, p < 0.001 for HLC; t 61.9 = 2.10, p = 0.040 for IS). The subcategories ( B ) also show similar patterns as those presented in Fig. 3 . Subcategories are significant when confidence intervals do not overlap with zero and were explicitly tested with two-tailed one sample t-test (t 30.52 = 2.17, p = 0.038 for CO 2 ; t 40.03 = 4.64, p < 0.001 for Enemy Release; t 47.45 = 2.18, p = 0.034 for Mean Temperature; t 110.81 = −4.05, p < 0.001 for Urbanization); all other subcategories have p > 0.20. Note that effect size and study numbers are lower here than in Figs. 3 and 4 , because log response ratios cannot be calculated for studies that provide coefficients (e.g., odds ratio) rather than raw data; as such, all observations within BC did not have associated RR values. Despite strong differences in sample size, patterns are consistent across effect sizes, and therefore, we can be confident that the results presented in the main text are not biased because of effect size selection.
Extended Data Fig. 5 Average standard errors of the effect sizes (A) and sample sizes per effect size (B) for each of the five global change drivers.
The displayed points represent the mean predicted values (with 95% confidence intervals) from the generalized linear mixed effects models with separate random intercepts for study (Gaussian distribution for standard error model, A ; Poisson distribution for sample size model, B ). Points that do not share letters are significantly different from one another (p < 0.05) based on a two-sided Tukey’s posthoc multiple comparison test with adjustment for multiple comparisons. Sample sizes (number of studies, n, and effect sizes, k) for each driver are as follows: n = 77, k = 392 for BC; n = 124, k = 364 for CP; n = 202, k = 380 for CC; n = 517, k = 1449 for HLC; n = 96, k = 355 for IS.
Extended Data Fig. 6 Forest plots of effect sizes, associated variances, and relative weights (A), Funnel plots (B), and Egger’s Test plots (C) for each of the five global change drivers and leave-one-out publication bias analyses (D).
In panel A , points are the individual effect sizes (Hedge’s G), error bars are standard errors of the effect size, and size of the points is the relative weight of the observation in the model, with larger points representing observations with higher weight in the model. Sample sizes are provided for each effect size in the meta-analytic database. Effect sizes were plotted in a random order. Egger’s tests indicated significant asymmetries (p < 0.05) in Biodiversity Change (worst asymmetry – likely not bias, just real effect of positive relationship between diversity and disease), Climate Change – (weak asymmetry, again likely not bias, climate change generally increases disease), and Introduced Species (relatively weak asymmetry – unclear whether this is a bias, may be driven by some outliers). No significant asymmetries (p > 0.05) were found in Chemical Pollution and Habitat Loss/Change, suggesting negligible publication bias in reported disease responses across these global change drivers ( B , C ). Egger’s test included publication year as moderator but found no significant relationship between Hedge’s g and publication year (p > 0.05) implying no temporal bias in effect size magnitude or direction. In panel D , the horizontal red lines denote the grand mean and SE of Hedge’s g and (g = 0.1009, SE = 0.0338). Grey points and error bars indicate the Hedge’s g and SEs, respectively, using the leave-one-out method (grand mean is recalculated after a given study is removed from dataset). While the removal of certain studies resulted in values that differed from the grand mean, all estimated Hedge’s g values fell well within the standard error of the grand mean. This sensitivity analysis indicates that our results were robust to the iterative exclusion of individual studies.
Extended Data Fig. 7 The effects of habitat loss/change on disease depend on parasite taxa and land use conversion contexts.
A) Enemy type influences the magnitude of the effect of urbanization on disease: helminths, protists, and arthropods were all negatively associated with urbanization, whereas viruses were non-significantly positively associated with urbanization. B) Reference (control) land use type influences the magnitude of the effect of urbanization on disease: disease was reduced in urban settings compared to rural and peri-urban settings, whereas there were no differences in disease along urbanization gradients or between urban and natural settings. C) The effect of forest fragmentation depends on whether a large/continuous habitat patch is compared to a small patch or whether disease it is measured along an increasing fragmentation gradient (Z = −2.828, p = 0.005). Conversely, the effect of deforestation on disease does not depend on whether the habitat has been destroyed and allowed to regrow (e.g., clearcutting, second growth forests, etc.) or whether it has been replaced with agriculture (e.g., row crop, agroforestry, livestock grazing; Z = 1.809, p = 0.0705). The displayed points represent the mean predicted values (with 95% confidence intervals) from a metafor model where the response variable was a Hedge’s g (representing the effect on an infectious disease endpoint relative to control), study was treated as a random effect, and the independent variables included enemy type (A), reference land use type (B), or land use conversion type (C). Data for (A) and (B) were only those studies that were within the “urbanization” subcategory; data for (C) were only those studies that were within the “deforestation” and “forest fragmentation” subcategories. Sample sizes (number of studies, n, and effect sizes, k) in (A) for each enemy are n = 48, k = 98 for Virus; n = 193, k = 343 for Protist; n = 159, k = 490 for Helminth; n = 10, k = 24 for Fungi; n = 103, k = 223 for Bacteria; and n = 30, k = 73 for Arthropod. Sample sizes in (B) for each reference land use type are n = 391, k = 1073 for Rural; n = 29, k = 74 for Peri-urban; n = 33, k = 83 for Natural; and n = 24, k = 58 for Urban Gradient. Sample sizes in (C) for each land use conversion type are n = 7, k = 47 for Continuous Gradient; n = 16, k = 44 for High/Low Fragmentation; n = 11, k = 27 for Clearcut/Regrowth; and n = 21, k = 43 for Agriculture.
Extended Data Fig. 8 The effects of common global change drivers on mean infectious disease responses in the literature depends on whether the endpoint is the host or parasite; whether the parasite is a vector, is vector-borne, has a complex or direct life cycle, or is a macroparasite; whether the host is an ectotherm or endotherm; or the venue and habitat in which the study was conducted.
A ) Parasite endpoints. B ) Vector-borne status. C ) Parasite transmission route. D ) Parasite size. E ) Venue. F ) Habitat. G ) Host thermy. H ) Parasite type (ecto- or endoparasite). See Table S 2 for number of studies and effect sizes across ecological contexts and global change drivers. See Table S 3 for pairwise comparison results. The displayed points represent the mean predicted values (with 95% confidence intervals) from a metafor model where the response variable was a Hedge’s g (representing the effect on an infectious disease endpoint relative to control), study was treated as a random effect, and the independent variables included the main effects and an interaction between global change driver and the focal independent variable (whether the endpoint measured was a host or parasite, whether the parasite is vector-borne, has a complex or direct life cycle, is a macroparasite, whether the study was conducted in the field or lab, habitat, the host is ectothermic, or the parasite is an ectoparasite).
Extended Data Fig. 9 The effects of five common global change drivers on mean infectious disease responses in the literature only occasionally depend on location, host taxon, and parasite taxon.
A ) Continent in which the field study occurred. Lack of replication in chemical pollution precluded us from including South America, Australia, and Africa in this analysis. B ) Host taxa. C ) Enemy taxa. See Table S 2 for number of studies and effect sizes across ecological contexts and global change drivers. See Table S 3 for pairwise comparison results. The displayed points represent the mean predicted values (with 95% confidence intervals) from a metafor model where the response variable was a Hedge’s g (representing the effect on an infectious disease endpoint relative to control), study was treated as a random effect, and the independent variables included the main effects and an interaction between global change driver and continent, host taxon, and enemy taxon.
Extended Data Fig. 10 The effects of human vs. non-human endpoints for the zoonotic disease subset of database and wild vs. domesticated animal endpoints for the non-human animal subset of database are consistent across global change drivers.
(A) Zoonotic disease responses measured on human hosts responded less positively (closer to zero when positive, further from zero when negative) than those measured on non-human (animal) hosts (Z = 2.306, p = 0.021). Note, IS studies were removed because of missing cells. (B) Disease responses measured on domestic animal hosts responded less positively (closer to zero when positive, further from zero when negative) than those measured on wild animal hosts (Z = 2.636, p = 0.008). These results were consistent across global change drivers (i.e., no significant interaction between endpoint and global change driver). As many of the global change drivers increase zoonotic parasites in non-human animals and all parasites in wild animals, this may suggest that anthropogenic change might increase the occurrence of parasite spillover from animals to humans and thus also pandemic risk. The displayed points represent the mean predicted values (with 95% confidence intervals) from a metafor model where the response variable was a Hedge’s g (representing the effect on an infectious disease endpoint relative to control), study was treated as a random effect, and the independent variable of global change driver and human/non-human hosts. Data for (A) were only those diseases that are considered “zoonotic”; data for (B) were only those endpoints that were measured on non-human animals. Sample sizes in (A) for zoonotic disease measured on human endpoints across global change drivers are n = 3, k = 17 for BC; n = 2, k = 6 for CP; n = 25, k = 39 for CC; and n = 175, k = 331 for HLC. Sample sizes in (A) for zoonotic disease measured on non-human endpoints across global change drivers are n = 25, k = 52 for BC; n = 2, k = 3 for CP; n = 18, k = 29 for CC; n = 126, k = 289 for HLC. Sample sizes in (B) for wild animal endpoints across global change drivers are n = 28, k = 69 for BC; n = 21, k = 44 for CP; n = 50, k = 89 for CC; n = 121, k = 360 for HLC; and n = 29, k = 45 for IS. Sample sizes in (B) for domesticated animal endpoints across global change drivers are n = 2, k = 4 for BC; n = 4, k = 11 for CP; n = 7, k = 20 for CC; n = 78, k = 197 for HLC; and n = 1, k = 2 for IS.
Supplementary information
Supplementary information.
Supplementary Discussion, Supplementary References and Supplementary Tables 1–3.
Reporting Summary
Peer review file, supplementary data 1.
R markdown code and output associated with this paper.
Supplementary Table 4
EcoEvo PRISMA checklist.
Rights and permissions
Springer Nature or its licensor (e.g. a society or other partner) holds exclusive rights to this article under a publishing agreement with the author(s) or other rightsholder(s); author self-archiving of the accepted manuscript version of this article is solely governed by the terms of such publishing agreement and applicable law.
Reprints and permissions
About this article
Cite this article.
Mahon, M.B., Sack, A., Aleuy, O.A. et al. A meta-analysis on global change drivers and the risk of infectious disease. Nature (2024). https://doi.org/10.1038/s41586-024-07380-6
Download citation
Received : 02 August 2022
Accepted : 03 April 2024
Published : 08 May 2024
DOI : https://doi.org/10.1038/s41586-024-07380-6
Share this article
Anyone you share the following link with will be able to read this content:
Sorry, a shareable link is not currently available for this article.
Provided by the Springer Nature SharedIt content-sharing initiative
By submitting a comment you agree to abide by our Terms and Community Guidelines . If you find something abusive or that does not comply with our terms or guidelines please flag it as inappropriate.
Quick links
- Explore articles by subject
- Guide to authors
- Editorial policies
Sign up for the Nature Briefing: Anthropocene newsletter — what matters in anthropocene research, free to your inbox weekly.


A .gov website belongs to an official government organization in the United States.
A lock ( ) or https:// means you've safely connected to the .gov website. Share sensitive information only on official, secure websites.
- How It Spreads
- Where Malaria Occurs
- World Malaria Day 2024
- Clinical Guidance: Malaria Diagnosis & Treatment in the U.S.
- Clinical Features
- Clinical Testing and Diagnosis
- Malaria Risk Assessment for Travelers
- Choosing a Drug to Prevent Malaria
- How to Report a Case of Malaria
- Public Health Strategy
- Malaria's Impact Worldwide
- Communication Resources
Every April 25, CDC joins the global community to highlight malaria, a serious parasitic disease spread to humans by a certain type of mosquito, Anopheles . Malaria remains a leading cause of preventable illness and death in the world.
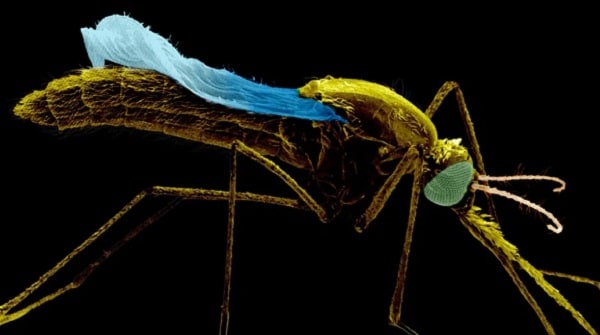
CDC’s World Malaria Day Materials
World malaria day 2024: dear colleague letter.

April 25, 2024
Dear Colleagues:
Today marks World Malaria Day, a day set aside to highlight the need for continued investment and sustained commitment to eliminate malaria. Malaria is a life-threatening disease spread to humans by Anopheles mosquitoes. It continues to be a cause of preventable illness and death in the world, resulting in nearly 249 million cases and 608,000 deaths across 85 countries in 2022. Africa bears the largest burden of malaria cases and deaths, with children and pregnant women being disproportionately affected. Children under five make up approximately 80% of malaria deaths in Africa, and malaria in pregnancy can lead to severe health consequences, including maternal and neonatal deaths.
Malaria also poses a threat domestically. There are nearly 2,000 cases of malaria reported each year, mostly due to international travel. However, last year, for the first time in 20 years, the United States experienced cases of locally acquired mosquito-transmitted malaria. Experts in the Centers for Disease Control and Prevention’s (CDC) Division of Parasitic Diseases and Malaria sprang into action, supporting early detection and a prompt response. With our state and local partners in the lead, CDC acted as a technical co-pilot, strengthening public health capacity. Public health teams working together was essential in limiting further local spread beyond the 10 cases across four states (Florida, Texas, Maryland, and Arkansas), serving as an important reminder that a global problem can quickly become a local one.
CDC’s priority is to protect health and improve lives, and since 1946, CDC has been a global leader in malaria prevention and control. We have led the development of many of the gold-standard malaria vector surveillance and control methods that are used around the world today. CDC co-implements the President’s Malaria Initiative, which is led by the United States Agency for International Development. CDC also provides technical recommendations to the U.S. Global Malaria Coordinator, as well as in-country expertise, driving progress toward malaria elimination. These efforts have contributed to preventing at least 2.1 billion malaria cases and 11.7 million malaria deaths since 2000, but threats remain.
Threats, including drug and insecticide resistance, invasive mosquito species, severe weather events, and limitations with rapid diagnostic tests, pose ongoing challenges to eliminating malaria. This year's World Malaria Day theme, "Accelerating the Fight Against Malaria for a More Equitable World,” highlights the importance of action in addressing malaria Thus, CDC and our global partners must work together to develop new interventions and support public health innovations to quickly address these threats. Recently, CDC developed a new rapid, cost-effective test for detecting an invasive malaria vector, Anopheles stephensi. This mosquito, unlike other malaria vectors, is resistant to many key insecticides, thrives in urban areas, and is starting to spread across parts of Africa. This novel tool can be used in resource-limited settings, allowing us to detect this emerging threat early, closely monitor its spread, and support rapid response. Developing such innovative tools supports global efforts to combat malaria and demonstrates CDC’s expertise and unwavering commitment to staying ahead of emerging threats.
As we reflect on these accomplishments and the progress made, let us recommit ourselves to the goal of eliminating malaria and working together to overcome these challenges and end this preventable disease. Together, we can make a tangible difference in protecting the health and improving the lives of millions affected by malaria and contribute to a healthier, more resilient world.
Thank you for your ongoing dedication and partnership in this vital work.
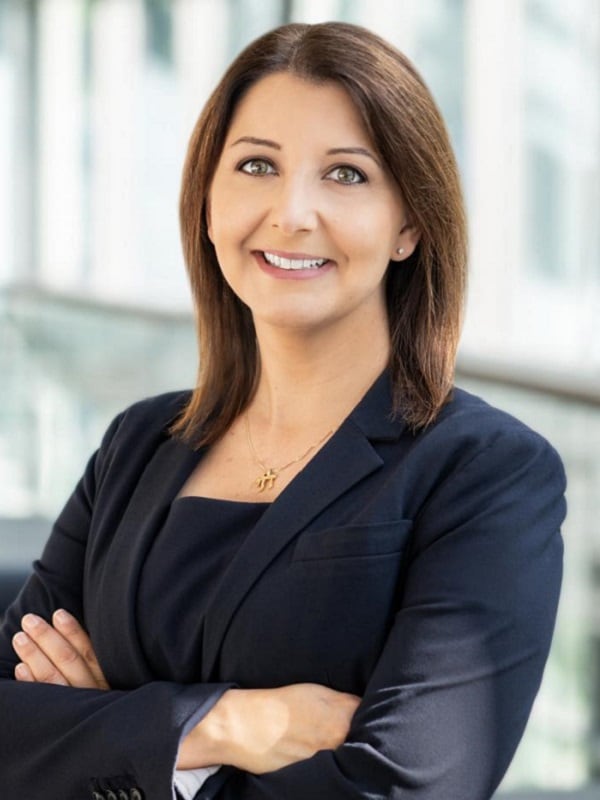
PDF version
World Malaria Day Feature 2024: Leading with Malaria Control Innovation in Mind
Every April 25, CDC joins the global community to highlight malaria, a serious parasitic disease spread to humans by a certain type of mosquito, Anopheles . Malaria remains a leading cause of preventable illness and death in the world. In 2022 , there were approximately 249 million malaria cases and 608,000 malaria deaths in 85 countries. Malaria disproportionately impacts women and young families, with children under five making up approximately 80% of malaria deaths and pregnant women facing severe health outcomes. Africa carries the largest burden, accounting for approximately 94% (233 million) malaria cases and 95% (580,000) malaria deaths.
CDC continues to be a global leader in malaria work, with our state-of-the-art insectary and entomology labs providing a platform for innovative research to address malaria's threat. This past summer, a team from CDC's Division of Parasitic Diseases and Malaria (DPDM)'s Entomology Branch created an innovative, simple, and accessible loop-mediated isothermal amplification (LAMP) assay for molecular identification of the invasive malaria vector Anopheles stephensi.
The team, led by molecular biologist Cristina Rafferty, included two interns from Summer 2023 through the Public Health Entomology for All (PHEFA) program :
- JeNiyah Scaife
- Gloria Raise
Understanding Anopheles stephensi (The threat)
Anopheles stephensi ( An. stephensi ) is a malaria vector native to South Asia but has since spread to Africa and poses a huge threat to the malaria landscape. In Africa, An. stephensi was first detected in Djibouti, a country that was on the cusp of malaria pre-elimination status in 2012 with only 27 presumed or confirmed cases. In 2020, malaria cases had reached more than 73,000, with many believing it was due to An. stephensi . On the African continent, the vector has also been detected in Sudan, Somalia, Eritrea, Ethiopia, Kenya, and more recently Nigeria and Ghana. If the spread continues, estimates predict it could put an additional 126 million people per year at risk of malaria, mostly in peri-urban/urban areas.
An. stephensi is also different from typical African malaria vectors; it's both urban and peri-urban as opposed to typical rural vectors, has different feeding behaviors, can breed in man-made containers, and is not seasonal, thus having the potential to transmit malaria year-round. It is also resistant to common insecticides. These attributes make the most common malaria vector interventions (e.g., indoor residual sprays and insecticide-treated nets) less effective against An. stephensi.
Access to fast and accurate molecular tools is integral in confirming the presence of An. stephensi in new locations. This is because it can be difficult to distinguish from other vectors morphologically. Up until now, there were no easily accessible molecular tools to rapidly detect invasions of An. stephensi, leading to significant challenges to early detection as it continues to spread across Africa.
Creating an accessible assay (The tech)
This past summer, CDC's team created a rapid colorimetric loop-mediated isothermal amplification assay for An. stephensi species identification, referred to as the CLASS assay. The team worked tirelessly rearing mosquitoes, conducting DNA extraction, analyzing PCRs and gels, and testing with different reagent concentrations at different times and temperatures. The team kept accessibility and field-friendliness at the forefront when designing the assay. Teams utilizing this assay do not need a full molecular laboratory nor highly trained molecular biologists to interpret the assays, which addresses major barriers that currently exist in the field.
Creating innovative tools to detect malaria vectors such as An. stephensi is key to global malaria control and elimination. Utilizing the CLASS assay will not only help with early detection and accurate identification of An. stephensi, but also further informs researchers on the changing malaria landscape and how to best prepare and rapidly respond.
A pre-print describing the CLASS assay is available now. Malaria programs have started to pilot the CLASS assay in countries, including Kenya, Ghana, Ethiopia, and Tanzania. The countries are using the assay for screening in the field, with additional follow up as necessary. The CDC team will gather valuable feedback from countries, further informing researchers about its use.
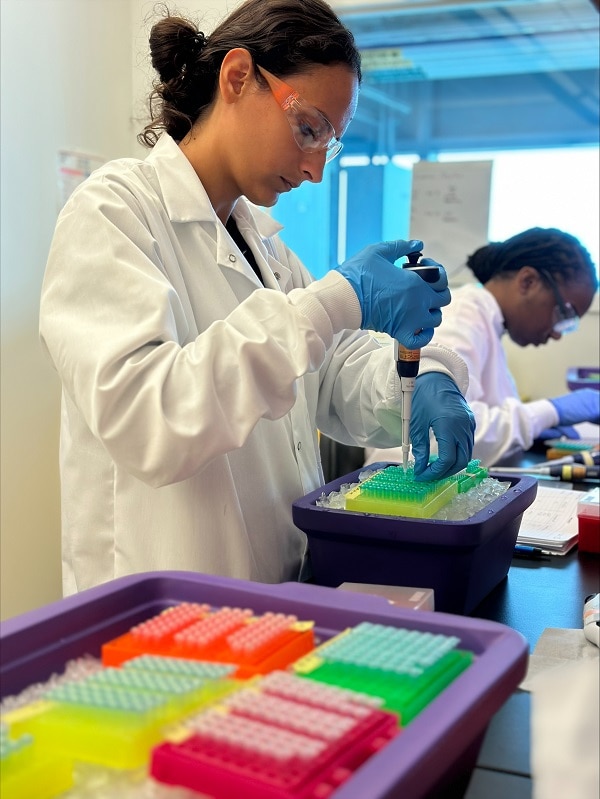
Investing in a diverse entomology workforce (The team)
Two PHEFA interns, JeNiyah Scaife and Gloria Raise, under the mentorship of molecular biologist Cristina Rafferty, conducted much of the lab work that supported optimizing the CLASS assay. PHEFA, a joint program between the Entomological Society of America (ESA) and CDC, aims to expand and diversify the pool of scholars interested in and entering the public health entomology field. Scaife and Raise attend undergraduate institutions that take deliberate steps to recruit, enroll, and retain students from underrepresented populations. They both completed a rigorous application and interview process before being selected by DPDM. Though the students were rising seniors majoring in forensic science, they each wanted to learn more about entomology.
"[...] a lot of people don't even know what entomology is; there's very few schools that have an entomology program, so I guess the aim of the program is to show that there is entomology connected with public health [...] it kind of shows what the power of entomology can have, and the impact it can have on people's health and lives," says Raise.
In addition to learning more about the field, the direct experience was invaluable. "I learned more in 10 weeks than I've learned in an entire semester at school," says Scaife. "As far as using some of the instruments in the lab, I feel more confidently when it comes to running PCRs, or using the thermal cycler, or running a gel; I feel like I know it like the back of my hand. I looked forward to going to the lab every morning to see what we could get done throughout the day or something new I was going to learn." Exuding pride, Rafferty, who mentored these students, said, "not only did they help to change the course of how the world can detect An. stephensi, but they also gave me hope in there being a more diverse and ready public health entomology workforce."
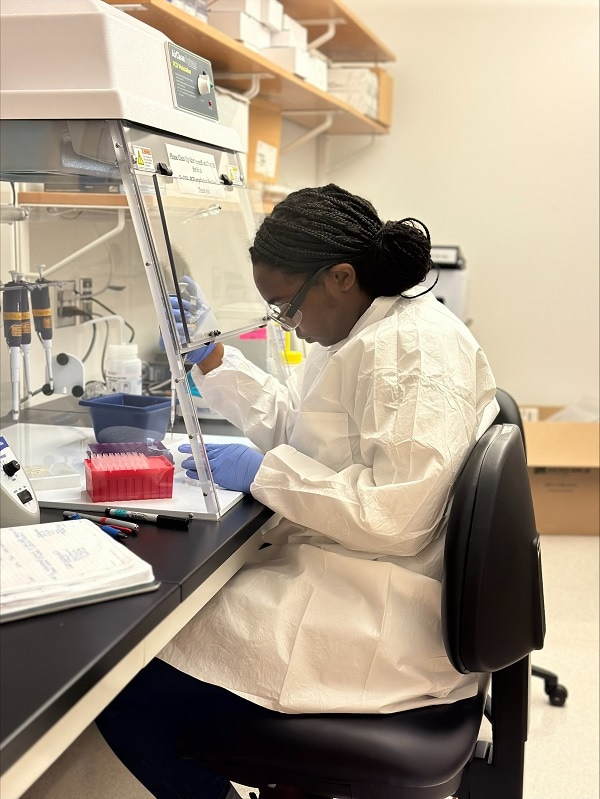
As malaria continues to evolve as a global threat to human health, we must continue to invest in innovative approaches to detect, prevent, control, and one day, eliminate it. These approaches require diverse and inclusive thinking. Efforts like PHEFA interns working to optimize and develop the CLASS assay is a keystone example of why such methods are paramount in public health. DPDM is proud to have talented individuals with diverse backgrounds and experiences contributing to innovative work that improves how CDC can address emerging public health threats, ultimately protecting the lives and livelihoods of the most vulnerable.
Malaria Fact Sheets and Infographics
Malaria social media cards.
Malaria is a serious disease caused by a parasite that infects the Anopheles mosquito. You get malaria when bitten by an infective mosquito.
For Everyone
Health care providers, public health.

An official website of the United States government
The .gov means it’s official. Federal government websites often end in .gov or .mil. Before sharing sensitive information, make sure you’re on a federal government site.
The site is secure. The https:// ensures that you are connecting to the official website and that any information you provide is encrypted and transmitted securely.
- Publications
- Account settings
Preview improvements coming to the PMC website in October 2024. Learn More or Try it out now .
- Advanced Search
- Journal List
- Glasgow Med J
- v.45(2); 1896 Feb

An Essay on Malaria and Its Consequences
Full text is available as a scanned copy of the original print version. Get a printable copy (PDF file) of the complete article (1.0M), or click on a page image below to browse page by page.
- Share full article
Advertisement
Supported by
Environmental Changes Are Fueling Human, Animal and Plant Diseases, Study Finds
Biodiversity loss, global warming, pollution and the spread of invasive species are making infectious diseases more dangerous to organisms around the world.

By Emily Anthes
Several large-scale, human-driven changes to the planet — including climate change, the loss of biodiversity and the spread of invasive species — are making infectious diseases more dangerous to people, animals and plants, according to a new study.
Scientists have documented these effects before in more targeted studies that have focused on specific diseases and ecosystems. For instance, they have found that a warming climate may be helping malaria expand in Africa and that a decline in wildlife diversity may be boosting Lyme disease cases in North America.
But the new research, a meta-analysis of nearly 1,000 previous studies, suggests that these patterns are relatively consistent around the globe and across the tree of life.
“It’s a big step forward in the science,” said Colin Carlson, a biologist at Georgetown University, who was not an author of the new analysis. “This paper is one of the strongest pieces of evidence that I think has been published that shows how important it is health systems start getting ready to exist in a world with climate change, with biodiversity loss.”
In what is likely to come as a more surprising finding, the researchers also found that urbanization decreased the risk of infectious disease.
The new analysis, which was published in Nature on Wednesday, focused on five “global change drivers” that are altering ecosystems across the planet: biodiversity change, climate change, chemical pollution, the introduction of nonnative species and habitat loss or change.
The researchers compiled data from scientific papers that examined how at least one of these factors affected various infectious-disease outcomes, such as severity or prevalence. The final data set included nearly 3,000 observations on disease risks for humans, animals and plants on every continent except for Antarctica.
The researchers found that, across the board, four of the five trends they studied — biodiversity change, the introduction of new species, climate change and chemical pollution — tended to increase disease risk.
“It means that we’re likely picking up general biological patterns,” said Jason Rohr, an infectious disease ecologist at the University of Notre Dame and senior author of the study. “It suggests that there are similar sorts of mechanisms and processes that are likely occurring in plants, animals and humans.”
The loss of biodiversity played an especially large role in driving up disease risk, the researchers found. Many scientists have posited that biodiversity can protect against disease through a phenomenon known as the dilution effect.
The theory holds that parasites and pathogens, which rely on having abundant hosts in order to survive, will evolve to favor species that are common, rather than those that are rare, Dr. Rohr said. And as biodiversity declines, rare species tend to disappear first. “That means that the species that remain are the competent ones, the ones that are really good at transmitting disease,” he said.
Lyme disease is one oft-cited example. White-footed mice, which are the primary reservoir for the disease, have become more dominant on the landscape, as other rarer mammals have disappeared, Dr. Rohr said. That shift may partly explain why Lyme disease rates have risen in the United States. (The extent to which the dilution effect contributes to Lyme disease risk has been the subject of debate, and other factors, including climate change, are likely to be at play as well.)
Other environmental changes could amplify disease risks in a wide variety of ways. For instance, introduced species can bring new pathogens with them, and chemical pollution can stress organisms’ immune systems. Climate change can alter animal movements and habitats, bringing new species into contact and allowing them to swap pathogens .
Notably, the fifth global environmental change that the researchers studied — habitat loss or change — appeared to reduce disease risk. At first glance, the findings might appear to be at odds with previous studies, which have shown that deforestation can increase the risk of diseases ranging from malaria to Ebola. But the overall trend toward reduced risk was driven by one specific type of habitat change: increasing urbanization.
The reason may be that urban areas often have better sanitation and public health infrastructure than rural ones — or simply because there are fewer plants and animals to serve as disease hosts in urban areas. The lack of plant and animal life is “not a good thing,” Dr. Carlson said. “And it also doesn’t mean that the animals that are in the cities are healthier.”
And the new study does not negate the idea that forest loss can fuel disease; instead, deforestation increases risk in some circumstances and reduces it in others, Dr. Rohr said.
Indeed, although this kind of meta-analysis is valuable for revealing broad patterns, it can obscure some of the nuances and exceptions that are important for managing specific diseases and ecosystems, Dr. Carlson noted.
Moreover, most of the studies included in the analysis examined just a single global change drive. But, in the real world, organisms are contending with many of these stressors simultaneously. “The next step is to better understand the connections among them,” Dr. Rohr said.
Emily Anthes is a science reporter, writing primarily about animal health and science. She also covered the coronavirus pandemic. More about Emily Anthes
Explore the Animal Kingdom
A selection of quirky, intriguing and surprising discoveries about animal life..
Scientists say they have found an “alphabet” in the songs of sperm whales , raising the possibility that the animals are communicating in a complex language.
Indigenous rangers in Australia’s Western Desert got a rare close-up with the northern marsupial mole , which is tiny, light-colored and blind, and almost never comes to the surface.
For the first time, scientists observed a primate in the wild treating a wound with a plant that has medicinal properties.
A new study resets the timing for the emergence of bioluminescence back to millions of years earlier than previously thought.
Scientists are making computer models to better understand how cicadas emerge collectively after more than a decade underground .

COMMENTS
Malaria is caused by the parasites, not by a virus or by a type of bacterium. If it isn't treated, malaria can cause severe health problems such as seizures, brain damage, trouble breathing, organ failure and death. The disease is rare in the U.S., with about 2,000 cases per year.
Malaria is a life-threatening disease spread to humans by some types of mosquitoes. It is mostly found in tropical countries. It is preventable and curable. The infection is caused by a parasite and does not spread from person to person. Symptoms can be mild or life-threatening.
In the early 21st century the incidence of malaria, and the number of deaths caused by the disease, appeared to be declining. For example, the World Health Organization (WHO) estimated that in 2000 there were 233 million cases of malaria worldwide, which resulted in roughly 985,000 deaths, mostly of young children in Africa. In 2010 there were ...
1. Introduction. Malaria affected an estimated 219 million people causing 435,000 deaths in 2017 globally. This burden of morbidity and mortality is a result of more than a century of global effort and research aimed at improving the prevention, diagnosis, and treatment of malaria [].Malaria is the most common disease in Africa and some countries in Asia with the highest number of indigenous ...
Worldwide estimates tell us that over a billion people are currently at high risk of malaria, and around 600,000 people die of this disease every year. 1 Many people who are exposed to malaria live in regions where poverty, lack of knowledge, and a shortage of healthcare are barriers to life-saving treatment.
Malaria is a treatable disease. Artemisinin-based combination therapies (ACTs) are the most effective antimalarial medicines available today and the mainstay of recommended treatment for Plasmodium falciparum malaria, the deadliest malaria parasite globally. ACTs combine 2 active pharmaceuticals with different mechanisms of action, including derivates of artemisinin extracted from the plant ...
Malaria is a preventable and treatable disease. Early diagnosis and treatment of malaria reduces disease and prevents deaths, and also contributes to reducing transmission. The best available treatment, particularly for Plasmodium falciparum malaria, is artemisinin-based combination therapy (ACT). Antimalarial medicines can also be used to ...
Malaria is a climate-sensitive disease, influenced by changes in temperature, rainfall, and humidity. There is growing concern that such changes might alter malaria's geographical distribution and transmission, expanding it into areas in which the disease has been controlled or in non-endemic areas. Patterns of distribution of vector-borne ...
This documentary video discusses the epidemiology of malaria; strategies for prevention, including vector control and vaccines; and the pipeline of promising new drugs for the fight to eliminate ma...
Malaria has had a profound effect on human lives for thousands of years and remains one of the most serious, life-threatening infectious diseases 1-3.The disease is caused by protozoan pathogens ...
Sub-Saharan Africa carried the brunt of the disease, where a child probably died of malaria every 45 seconds and efforts to control the disease were very limited. Yet this desperate situation had ...
Malaria disease can be categorized as uncomplicated or severe (complicated). In general, malaria is a curable disease if diagnosed and treated promptly and correctly. All the clinical symptoms associated with malaria are caused by the asexual erythrocytic or blood stage parasites. When the parasite develops in the erythrocyte, numerous known ...
Malaria. Malaria is a mosquito-borne disease caused by a parasite. People with malaria often experience fever, chills, and flu-like illness. Left untreated, they may develop severe complications and die. In 2020 an estimated 241 million cases of malaria occurred worldwide and 627,000 people died, mostly children in sub-Saharan Africa.
Malaria is a disease caused by a parasite. The parasite is spread to humans through the bites of infected mosquitoes. People who have malaria usually feel very sick with a high fever and shaking chills. While the disease is uncommon in temperate climates, malaria is still common in tropical and subtropical countries. Each year nearly 290 ...
The outlook for malaria control is grim. The disease, caused by mosquito-borne parasites, is present in 102 countries and is responsible for over 100 million clinical cases and 1 to 2 million deaths each year. Over the past two decades, efforts to control malaria have met with less and less success. In many regions where malaria transmission had been almost eliminated, the disease has made a ...
Malaria is a mosquito-borne disease that is caused by Plasmodium parasites. Patients with malaria experience flu-like symptoms and, in severe cases, the disease can progress to neurological ...
Factors associated with the risk of malaria among children: analysis of 2021 Nigeria Malaria Indicator Survey. Malaria remains a burden globally, with the African region accounting for 94% of the overall disease burden and deaths in 2019. It is the major cause of morbidity and mortality among children in Nigeria.
Malaria is a difficult disease to control largely due to the highly adaptable nature of the vector and parasites involved. While effective tools have been and will continue to be developed to combat malaria, inevitably, over time the parasites and mosquitoes will evolve means to circumvent those tools if used in isolation or used ineffectively.
Aims and Scope. Malaria Journal is aimed at the scientific community interested in malaria in its broadest sense. It is the only journal that publishes exclusively articles on malaria and, as such, it aims to bring together knowledge from the different specialties involved in this very broad discipline, from the bench to the bedside and to the ...
Malaria is an infectious disease in tropical countries and is spread by mosquitoes. ... Please use one of the following formats to cite this article in your essay, paper or report: APA. Mandal ...
There was wide variation in the geographical coverage of papers by disease (Figure 4). Apart from malaria, dengue and chikungunya, and schistosomiasis, there were <10 papers per disease per country for every country, with many endemic countries not featured in any papers for several NTDs.
As a result of the interplay between many factors, the control of this disease can be challenging. However, few studies have demonstrated malaria's complexity, control, and modeling although this perspective could lead to effective policy recommendations. This paper aims to be a didactic material providing the reader with an overview of malaria.
Early malaria control work consisted primarily of insecticide spraying and draining water from mosquito breeding sites. In 1951, malaria was declared eliminated from the United States. Now, 70 years later, our name may have changed, but our mission to stamp out malaria—both internationally and domestically—remains very much intact.
Malaria is a climate-sensitive, vector-borne disease that caused 619,000 deaths among 247 million cases in 2021 ( 1 ). This burden is acutely focused on Africa, where 95% of global cases are reported ( 1 ). Reductions in cases in Africa have slowed or even reversed in recent years, attributed in part to a stall in investments in global ...
The database resulting from our literature search includes 972 studies and 2,938 observations of global change drivers on disease or parasitism from 1,006 parasite taxa, 480 host taxa and 1,497 ...
Malaria is a life-threatening disease spread to humans by Anopheles mosquitoes. It continues to be a cause of preventable illness and death in the world, resulting in nearly 249 million cases and 608,000 deaths across 85 countries in 2022. Africa bears the largest burden of malaria cases and deaths, with children and pregnant women being ...
An Essay on Malaria and Its Consequences - PMC. Journal List. Glasgow Med J. v.45 (2); 1896 Feb. PMC5950432. As a library, NLM provides access to scientific literature. Inclusion in an NLM database does not imply endorsement of, or agreement with, the contents by NLM or the National Institutes of Health.
The researchers compiled data from scientific papers that examined how at least one of these factors affected various infectious-disease outcomes, such as severity or prevalence.
Photo Essay. Information for Researchers. Food Allergy. Why Food Allergy is a Priority for NIAID. ... NIH Study Shows Chronic Wasting Disease Unlikely to Move from Animals to People May 17, 2024 ... April 27, 2024. Experimental NIH Malaria Monoclonal Antibody Protective in Malian Children April 26, 2024. About NIAID Toggle submenu. Who We Are.