DigitalCommons@UNMC
Home > Eppley Institute > Theses & Dissertations

Theses & Dissertations: Cancer Research
Theses/dissertations from 2024 2024.
Novel Spirocyclic Dimer (SpiD3) Displays Potent Preclinical Effects in Hematological Malignancies , Alexandria Eiken
Therapeutic Effects of BET Protein Inhibition in B-cell Malignancies and Beyond , Audrey L. Smith
Identifying the Molecular Determinants of Lung Metastatic Adaptation in Prostate Cancer , Grace M. Waldron
Identification of Mitotic Phosphatases and Cyclin K as Novel Molecular Targets in Pancreatic Cancer , Yi Xiao
Theses/Dissertations from 2023 2023
Development of Combination Therapy Strategies to Treat Cancer Using Dihydroorotate Dehydrogenase Inhibitors , Nicholas Mullen
Overcoming Resistance Mechanisms to CDK4/6 Inhibitor Treatment Using CDK6-Selective PROTAC , Sarah Truong
Theses/Dissertations from 2022 2022
Omics Analysis in Cancer and Development , Emalie J. Clement
Investigating the Role of Splenic Macrophages in Pancreatic Cancer , Daisy V. Gonzalez
Polymeric Chloroquine in Metastatic Pancreatic Cancer Therapy , Rubayat Islam Khan
Evaluating Targets and Therapeutics for the Treatment of Pancreatic Cancer , Shelby M. Knoche
Characterization of 1,1-Diarylethylene FOXM1 Inhibitors Against High-Grade Serous Ovarian Carcinoma Cells , Cassie Liu
Novel Mechanisms of Protein Kinase C α Regulation and Function , Xinyue Li
SOX2 Dosage Governs Tumor Cell Identity and Proliferation , Ethan P. Metz
Post-Transcriptional Control of the Epithelial-to-Mesenchymal Transition (EMT) in Ras-Driven Colorectal Cancers , Chaitra Rao
Use of Machine Learning Algorithms and Highly Multiplexed Immunohistochemistry to Perform In-Depth Characterization of Primary Pancreatic Tumors and Metastatic Sites , Krysten Vance
Characterization of Metastatic Cutaneous Squamous Cell Carcinoma in the Immunosuppressed Patient , Megan E. Wackel
Visceral adipose tissue remodeling in pancreatic ductal adenocarcinoma cachexia: the role of activin A signaling , Pauline Xu
Phos-Tag-Based Screens Identify Novel Therapeutic Targets in Ovarian Cancer and Pancreatic Cancer , Renya Zeng
Theses/Dissertations from 2021 2021
Functional Characterization of Cancer-Associated DNA Polymerase ε Variants , Stephanie R. Barbari
Pancreatic Cancer: Novel Therapy, Research Tools, and Educational Outreach , Ayrianne J. Crawford
Apixaban to Prevent Thrombosis in Adult Patients Treated With Asparaginase , Krishna Gundabolu
Molecular Investigation into the Biologic and Prognostic Elements of Peripheral T-cell Lymphoma with Regulators of Tumor Microenvironment Signaling Explored in Model Systems , Tyler Herek
Utilizing Proteolysis-Targeting Chimeras to Target the Transcriptional Cyclin-Dependent Kinases 9 and 12 , Hannah King
Insights into Cutaneous Squamous Cell Carcinoma Pathogenesis and Metastasis Using a Bedside-to-Bench Approach , Marissa Lobl
Development of a MUC16-Targeted Near-Infrared Antibody Probe for Fluorescence-Guided Surgery of Pancreatic Cancer , Madeline T. Olson
FGFR4 glycosylation and processing in cholangiocarcinoma promote cancer signaling , Andrew J. Phillips
Theses/Dissertations from 2020 2020
Cooperativity of CCNE1 and FOXM1 in High-Grade Serous Ovarian Cancer , Lucy Elge
Characterizing the critical role of metabolic and redox homeostasis in colorectal cancer , Danielle Frodyma
Genomic and Transcriptomic Alterations in Metabolic Regulators and Implications for Anti-tumoral Immune Response , Ryan J. King
Dimers of Isatin Derived Spirocyclic NF-κB Inhibitor Exhibit Potent Anticancer Activity by Inducing UPR Mediated Apoptosis , Smit Kour
From Development to Therapy: A Panoramic Approach to Further Our Understanding of Cancer , Brittany Poelaert
The Cellular Origin and Molecular Drivers of Claudin-Low Mammary Cancer , Patrick D. Raedler
Mitochondrial Metabolism as a Therapeutic Target for Pancreatic Cancer , Simon Shin
Development of Fluorescent Hyaluronic Acid Nanoparticles for Intraoperative Tumor Detection , Nicholas E. Wojtynek
Theses/Dissertations from 2019 2019
The role of E3 ubiquitin ligase FBXO9 in normal and malignant hematopoiesis , R. Willow Hynes-Smith
BRCA1 & CTDP1 BRCT Domainomics in the DNA Damage Response , Kimiko L. Krieger
Targeted Inhibition of Histone Deacetyltransferases for Pancreatic Cancer Therapy , Richard Laschanzky
Human Leukocyte Antigen (HLA) Class I Molecule Components and Amyloid Precursor-Like Protein 2 (APLP2): Roles in Pancreatic Cancer Cell Migration , Bailee Sliker
Theses/Dissertations from 2018 2018
FOXM1 Expression and Contribution to Genomic Instability and Chemoresistance in High-Grade Serous Ovarian Cancer , Carter J. Barger
Overcoming TCF4-Driven BCR Signaling in Diffuse Large B-Cell Lymphoma , Keenan Hartert
Functional Role of Protein Kinase C Alpha in Endometrial Carcinogenesis , Alice Hsu
Functional Signature Ontology-Based Identification and Validation of Novel Therapeutic Targets and Natural Products for the Treatment of Cancer , Beth Neilsen
Elucidating the Roles of Lunatic Fringe in Pancreatic Ductal Adenocarcinoma , Prathamesh Patil
Theses/Dissertations from 2017 2017
Metabolic Reprogramming of Pancreatic Ductal Adenocarcinoma Cells in Response to Chronic Low pH Stress , Jaime Abrego
Understanding the Relationship between TGF-Beta and IGF-1R Signaling in Colorectal Cancer , Katie L. Bailey
The Role of EHD2 in Triple-Negative Breast Cancer Tumorigenesis and Progression , Timothy A. Bielecki
Perturbing anti-apoptotic proteins to develop novel cancer therapies , Jacob Contreras
Role of Ezrin in Colorectal Cancer Cell Survival Regulation , Premila Leiphrakpam
Evaluation of Aminopyrazole Analogs as Cyclin-Dependent Kinase Inhibitors for Colorectal Cancer Therapy , Caroline Robb
Identifying the Role of Janus Kinase 1 in Mammary Gland Development and Breast Cancer , Barbara Swenson
DNMT3A Haploinsufficiency Provokes Hematologic Malignancy of B-Lymphoid, T-Lymphoid, and Myeloid Lineage in Mice , Garland Michael Upchurch
Theses/Dissertations from 2016 2016
EHD1 As a Positive Regulator of Macrophage Colony-Stimulating Factor-1 Receptor , Luke R. Cypher
Inflammation- and Cancer-Associated Neurolymphatic Remodeling and Cachexia in Pancreatic Ductal Adenocarcinoma , Darci M. Fink
Role of CBL-family Ubiquitin Ligases as Critical Negative Regulators of T Cell Activation and Functions , Benjamin Goetz
Exploration into the Functional Impact of MUC1 on the Formation and Regulation of Transcriptional Complexes Containing AP-1 and p53 , Ryan L. Hanson
DNA Polymerase Zeta-Dependent Mutagenesis: Molecular Specificity, Extent of Error-Prone Synthesis, and the Role of dNTP Pools , Olga V. Kochenova
Defining the Role of Phosphorylation and Dephosphorylation in the Regulation of Gap Junction Proteins , Hanjun Li
Molecular Mechanisms Regulating MYC and PGC1β Expression in Colon Cancer , Jamie L. McCall
Pancreatic Cancer Invasion of the Lymphatic Vasculature and Contributions of the Tumor Microenvironment: Roles for E-selectin and CXCR4 , Maria M. Steele
Altered Levels of SOX2, and Its Associated Protein Musashi2, Disrupt Critical Cell Functions in Cancer and Embryonic Stem Cells , Erin L. Wuebben
Theses/Dissertations from 2015 2015
Characterization and target identification of non-toxic IKKβ inhibitors for anticancer therapy , Elizabeth Blowers
Effectors of Ras and KSR1 dependent colon tumorigenesis , Binita Das
Characterization of cancer-associated DNA polymerase delta variants , Tony M. Mertz
A Role for EHD Family Endocytic Regulators in Endothelial Biology , Alexandra E. J. Moffitt
Biochemical pathways regulating mammary epithelial cell homeostasis and differentiation , Chandrani Mukhopadhyay
EPACs: epigenetic regulators that affect cell survival in cancer. , Catherine Murari
Role of the C-terminus of the Catalytic Subunit of Translesion Synthesis Polymerase ζ (Zeta) in UV-induced Mutagensis , Hollie M. Siebler
LGR5 Activates TGFbeta Signaling and Suppresses Metastasis in Colon Cancer , Xiaolin Zhou
LGR5 Activates TGFβ Signaling and Suppresses Metastasis in Colon Cancer , Xiaolin Zhou
Theses/Dissertations from 2014 2014
Genetic dissection of the role of CBL-family ubiquitin ligases and their associated adapters in epidermal growth factor receptor endocytosis , Gulzar Ahmad
Strategies for the identification of chemical probes to study signaling pathways , Jamie Leigh Arnst
Defining the mechanism of signaling through the C-terminus of MUC1 , Roger B. Brown
Targeting telomerase in human pancreatic cancer cells , Katrina Burchett
The identification of KSR1-like molecules in ras-addicted colorectal cancer cells , Drew Gehring
Mechanisms of regulation of AID APOBEC deaminases activity and protection of the genome from promiscuous deamination , Artem Georgievich Lada
Characterization of the DNA-biding properties of human telomeric proteins , Amanda Lakamp-Hawley
Studies on MUC1, p120-catenin, Kaiso: coordinate role of mucins, cell adhesion molecules and cell cycle players in pancreatic cancer , Xiang Liu
Epac interaction with the TGFbeta PKA pathway to regulate cell survival in colon cancer , Meghan Lynn Mendick
Theses/Dissertations from 2013 2013
Deconvolution of the phosphorylation patterns of replication protein A by the DNA damage response to breaks , Kerry D. Brader
Modeling malignant breast cancer occurrence and survival in black and white women , Michael Gleason
The role of dna methyltransferases in myc-induced lymphomagenesis , Ryan A. Hlady
Design and development of inhibitors of CBL (TKB)-protein interactions , Eric A. Kumar
Pancreatic cancer-associated miRNAs : expression, regulation and function , Ashley M. Mohr
Mechanistic studies of mitochondrial outer membrane permeabilization (MOMP) , Xiaming Pang
Novel roles for JAK2/STAT5 signaling in mammary gland development, cancer, and immune dysregulation , Jeffrey Wayne Schmidt
Optimization of therapeutics against lethal pancreatic cancer , Joshua J. Souchek
Theses/Dissertations from 2012 2012
Immune-based novel diagnostic mechanisms for pancreatic cancer , Michael J. Baine
Sox2 associated proteins are essential for cell fate , Jesse Lee Cox
KSR2 regulates cellular proliferation, transformation, and metabolism , Mario R. Fernandez
Discovery of a novel signaling cross-talk between TPX2 and the aurora kinases during mitosis , Jyoti Iyer
Regulation of metabolism by KSR proteins , Paula Jean Klutho
The role of ERK 1/2 signaling in the dna damage-induced G2 , Ryan Kolb
Regulation of the Bcl-2 family network during apoptosis induced by different stimuli , Hernando Lopez
Studies on the role of cullin3 in mitosis , Saili Moghe
Characteristics of amyloid precursor-like protein 2 (APLP2) in pancreatic cancer and Ewing's sarcoma , Haley Louise Capek Peters
Structural and biophysical analysis of a human inosine triphosphate pyrophosphatase polymorphism , Peter David Simone
Functions and regulation of Ron receptor tyrosine kinase in human pancreatic cancer and its therapeutic applications , Yi Zou
Theses/Dissertations from 2011 2011
Coordinate detection of new targets and small molecules for cancer therapy , Kurt Fisher
The role of c-Myc in pancreatic cancer initiation and progression , Wan-Chi Lin
The role of inosine triphosphate pyrophosphatase (ITPA) in maintanence [sic] of genomic stability in human cells , Miriam-Rose Menezes
- Eppley Institute Website
- McGoogan Library
Advanced Search
- Notify me via email or RSS
- Collections
- Disciplines
Author Corner
Home | About | FAQ | My Account | Accessibility Statement
Privacy Copyright
- Harvard Medical School
- HMS Theses and Dissertations
- Communities & Collections
- By Issue Date
- FAS Department
- Quick submit
- Waiver Generator
- DASH Stories
- Accessibility
- COVID-related Research
Terms of Use
- Privacy Policy
- By Collections
- By Departments
Targeted Therapies for the Treatment of Metastatic Breast Cancer
Citable link to this page
Collections.
- HMS Theses and Dissertations [520]
Contact administrator regarding this item (to report mistakes or request changes)
Advertisement
Nanoparticle-mediated cancer cell therapy: basic science to clinical applications
- Open access
- Published: 24 February 2023
- Volume 42 , pages 601–627, ( 2023 )
Cite this article
You have full access to this open access article
- Jaya Verma 1 ,
- Caaisha Warsame 1 ,
- Rajkumar Kottayasamy Seenivasagam 2 ,
- Nirmal Kumar Katiyar 1 ,
- Eiman Aleem 3 &
- Saurav Goel ORCID: orcid.org/0000-0002-8694-332X 1 , 4
6506 Accesses
15 Citations
1 Altmetric
Explore all metrics
Every sixth person in the world dies due to cancer, making it the second leading severe cause of death after cardiovascular diseases. According to WHO, cancer claimed nearly 10 million deaths in 2020. The most common types of cancers reported have been breast (lung, colon and rectum, prostate cases), skin (non-melanoma) and stomach. In addition to surgery, the most widely used traditional types of anti-cancer treatment are radio- and chemotherapy. However, these do not distinguish between normal and malignant cells. Additional treatment methods have evolved over time for early detection and targeted therapy of cancer. However, each method has its limitations and the associated treatment costs are quite high with adverse effects on the quality of life of patients. Use of individual atoms or a cluster of atoms (nanoparticles) can cause a paradigm shift by virtue of providing point of sight sensing and diagnosis of cancer. Nanoparticles (1–100 nm in size) are 1000 times smaller in size than the human cell and endowed with safer relocation capability to attack mechanically and chemically at a precise location which is one avenue that can be used to destroy cancer cells precisely. This review summarises the extant understanding and the work done in this area to pave the way for physicians to accelerate the use of hybrid mode of treatments by leveraging the use of various nanoparticles.
Similar content being viewed by others

Understanding the Novel Approach of Nanoferroptosis for Cancer Therapy
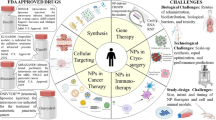
Nanoparticles for Cancer Therapy: Current Progress and Challenges
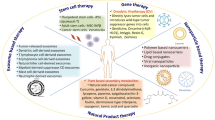
Cancer treatment therapies: traditional to modern approaches to combat cancers
Avoid common mistakes on your manuscript.
1 Introduction
In 2020, 19.3 million new cases of cancer were reported and nearly 50% of this succumbed to death. According to the American cancer society, it is expected that the number will grow to about 28.4 million new cancer cases and 16.3 million cancer deaths by 2040 which will be a 47% rise from 2020 [ 1 ]. It is noteworthy to mention that in 1927, cancer was named one of the top three causes of death in America by the US Census Bureau ( AACR: Landmarks in cancer research. 1907–2007 ). Over these 100 years, although therapeutic advances have increased the overall survival (OS) rates, there is still no cure for cancer. Some of the earliest evidence of cancer suggestive of osteosarcoma was found in human mummies in ancient Egypt. The oldest description of cancer from the so called the ‘Edwin Smith Papyrus’ dates to about 3000 BC in Egypt. The papyrus, which is from an ancient trauma surgery textbook, describes 8 cases of breast tumours or ulcers that were removed by cauterisation with a tool called fire drill. Hippocrates (460‐360 BC) is attributed with giving tumours the name Karkinoma ‘carcinoma’, and thus ‘cancer’ from the finger-like extensions (veins) stemming from the main body of a breast lesion that resembled a crab [ 2 ]. Over the last 2000 years, progress in our knowledge about cancer was slow until the twentieth century when the discovery of DNA double helix in 1953 by Watson and Crick, and later the human genome project revolutionised our knowledge of genomes, and cancer biology.
The cancer cell has a set of eight hallmarks or functional capabilities characteristic of malignancy, which include uncontrolled cell proliferation, and evading apoptosis [ 3 ]. The process of normal and abnormal cell growths can better be understood from Fig. 1 , which shows how a normal tissue transforms into a tumour causing death of individuals.
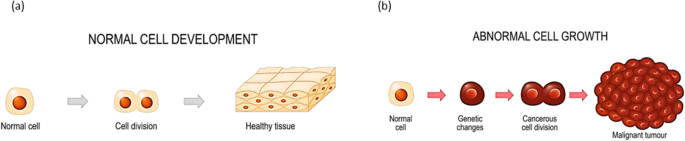
Image illustrating the cell development showing a normal cell growth and b abnormal cell growth [ 4 ]
There are two types of tumours (i) benign and (ii) malignant (cancerous). Currently, more than 100 different types of cancer exist and are named after either the tissue or organ they originate from.
Cancers that starts in specific kinds of cells fall into the following categories: carcinoma is the most frequent cancer that arises in epithelial tissue and affects the breast, kidney, liver, skin, lungs gland pancreas, neck and head [ 5 ]. Sarcoma affects connective tissues including muscles, bones, blood vessels and cartilage. Leukaemia arises mainly from the bone marrow. Myeloma and lymphoma are malignancies of the immune system [ 6 ]. Other types of tumours include Germ cell tumours and neuroendocrine tumours and carcinoid tumours, which are generally detected in the gastrointestinal system [ 6 ] [ 7 ]. Additionally, one vital feature of cancer cell is the unusual growth from a single cell, usually harbouring driver mutation (s) that result in clonal expansion. Such development was observed by the analysis of X chromosome inactivation which occurs randomly during embryonic proliferation and are the single cell origin of various tumours [ 8 ]. The multistep process of cancer development, at the cellular level, involves genetic and epigenetic modifications that drive cellular transformation and the acquirement of sustained proliferation survival, invasion and metastasis [ 7 , 8 ]. Treatment of benign tumour involves surgical removal if it compresses nearby structures or becomes gradually malignant. Treatment of malignant tumours consists of multi-modality treatment involving combinations of radiotherapy, immunotherapy, surgery, chemotherapy or targeted therapy.
R&D labs across the globe have focussed their research on early detection and cost effective treatment of various cancers [ 9 ]. Over the last decade, the treatment of cancer is moving towards precision medicine and less invasive strategies. Targeted therapy of cancer involves molecular level manipulation of proliferation, angiogenesis, cell death, invasion or immunosuppression. The rapid advances in nanotechnology enables the integration of inorganic nanomaterials with biomatter and drugs, which can be used for early detection, and treatment of cancer [ 10 ]. Nanoscience has opened new vistas to treat chemoresistant cancer cells through targeted delivery [ 11 , 12 ]. Several nanoscience integrated therapeutics have evolved from basic research to clinical discoveries [ 12 ]. Different kinds of nanoparticle technologies like low frequency mechanical vibrations by magnetic nanoparticles are being used to accelerate cell death [ 13 ].
The treatment of cancer cells using a materials science approach presents a great opportunity for multidisciplinary research. The targeted use of nanoparticles can enable new treatment methods. Nanoparticles are 1–100 nm size atomic clusters of matter. Research into inorganic nanoparticles and their interactions with the biological systems are in developing stages to establish their response and tune them as per desired properties by functionalisation [ 14 ] as well as nanocarriers [ 12 ]. Due to their high surface-to-volume ratio, high density of binding sites can be accommodated and triggered for their function such as binding to specific sites, release the drug at certain time/temperature/pH, in controlled manner, etc.
2 Historical perspective of cancer treatment
There are several approaches towards cancer treatment including surgery, radio- and chemotherapy, and most recently targeted immunotherapy.
2.1 Surgery
Historically, Maimonides in AD 1190 appears to be the first to document surgery as a method to remove tumours [ 15 ]. It was not until the nineteenth and early twentieth centuries that major advances were made in cancer surgery, especially after anaesthesia became available in 1846. Halsted developed the radical mastectomy during the last decade of the nineteenth century [ 16 ]. Most women with breast cancer nowadays have the primary tumour removed followed by adjuvant therapy that may include radiation, chemotherapy, targeted, or hormonal therapy. Progress in cellular, molecular and imaging techniques in the twentieth century was instrumental in the advancement of surgical techniques. For example, ultrasound (sonography), computed tomography (CT scans), magnetic resonance imaging (MRI scans) and positron emission tomography (PET scans) have replaced the exploratory surgeries used previously to diagnose cancer. Advances in surgical techniques also include laparoscopic, thoracoscopic surgeries, endoscopy, lasers, cryoablation and radiofrequency ablation [ 17 ].
The discovery and understanding of metastasis placed limitations on the use of surgery to treat invasive cancer, and other therapeutic interventions have been introduced.
2.2 Radiotherapy
Shortly after the discovery of X-rays by Wilhelm Rontgen in 1895, radiotherapy as a treatment option for cancer started to emerge. Currently, almost half of all patients with cancer are treated with radiation. Ionising radiation induces DNA damage leading to cancer cell death, but it has toxic effects on normal tissue [ 18 ]. Few years after its discovery, radiation therapy was found to cause cancer. Radiation carcinogenesis was established in human populations, and the dose–response relationship was described in radiation leukaemia [ 19 ]. Advances in radiation physics and computer technology in the twentieth century made it possible to aim radiation more precisely at tumours. For example, conformal radiation therapy (CRT) allows three-dimensional anatomical information of the tumour and surrounding healthy tissues, thus facilitating the establishment of three-dimensional conformal radiotherapy (3D-CRT) and radiation beams are delivered to the tumour from several directions. Intensity-modulated radiation therapy (IMRT) allows the intensity of the beams to be adjusted, thus delivering high dose to the cancer while decreasing the dose reaching the surrounding normal tissue [ 20 ]. Several technologies have been developed, the goal of which is to protect the surrounding normal tissue from the DNA damaging effects of radiation.
2.3 Chemotherapy
The discovery of chemotherapy was a result of observations that soldiers in World War II exposed to nitrogen mustard had low white blood cells count. This led to the discovery that intravenous nitrogen mustard slowed the growth of lymphomas and leukaemia in patients refractory to radiotherapy [ 21 ]. Nitrogen mustard was approved for cancer treatment in 1949. Later in 1948, and in 1950, the first successful chemotherapy for childhood leukaemia, and the first rationally conceived nucleotide analogue chemotherapeutic agents were developed, respectively [ 22 , 23 ]. Aminopterin discovered by Farber was the predecessor of methotrexate, which is commonly used today. Since then, hundreds of chemotherapeutic agents were developed and proved successful in inducing long-term remission. The use of combination chemotherapy proved advantageous over single agents. Some types of very fast-growing leukaemia and lymphoma respond very well to combination chemotherapy. For example, the remission induction therapy for acute myeloid leukaemia (AML) consists of 4–5 cycles of intensive chemotherapy, which typically includes cytarabine (Ara-C), the backbone for many therapeutic regimens, combined with etoposide and anthracycline [ 24 ]. Standard treatment with intensive induction chemotherapy for AML induces complete remission (CR) in 60–80% of patients aged 60 years and under. In paediatric AML, the overall remission-induction rates are approximately 85 to 95% and event-free survival (EFS) rates range from 50 to 65% [ 25 ].
Cytotoxic chemotherapy has many limitations such as drugs cannot distinguish between normal and cancer cells, they cause significant side effects that affect the quality of life of patients, and they usually target fast proliferating cancer cells, not cancer stem cells. For example, following 5-day cultivation with gemcitabine in vivo , pancreatic cancer stem cells were enriched up to 47.2% compared to 1.47% in a primary cancer cell line [ 26 ]. One of the major challenges that affects patients’ response to chemotherapy is drug resistance. For example, acquired resistance to Ara-C is a major obstacle in the clinical management of AML. Increasing the intensity of the current chemotherapy regimens does not improve outcomes because of the high percentage of treatment-related deaths (5–10%), and of long-term side effects [ 27 ]. Repurposing drugs such as disulfiram has been useful in overcoming Ara-C and bortezomib resistance in Down syndrome–associated AML cell lines [ 28 ].
2.4 Targeted therapy
The advances in our understanding of cancer biology and the human genome revolutionised therapeutics which block specific molecular pathways essential for cancer cell survival, cancer growth, progression and metastasis. Targeted cancer therapies are developed to interrupt a specific component of the complex network of altered signalling pathways that ultimately results in uncontrolled cell proliferation [ 29 ]. Molecular targeted therapies have shown remarkable success in the treatment of several cancer types including breast, leukaemia, colorectal, lung and ovarian cancers [ 30 ]. The first targeted cancer therapy was tamoxifen approved in 1977 for the treatment of breast cancer. Tamoxifen binds to the oestrogen receptor (ER), and therefore modulates ER activity, thus providing an effective treatment option for patients with ER-positive breast cancer [ 31 ]. Several mechanisms lead to aberrant functions by protein tyrosine kinases encoded by oncogenes, and subsequent cellular transformation. These include genomic rearrangements resulting in oncogenic fusion proteins (examples include BCR-ABL in chronic myelogenous leukaemia, PAX3-FOXO1 in alveolar rhabdomyosarcoma). Additionally, gain-of-function mutations, overexpression, gene amplification, and loss of the normal regulatory constraints of kinase activation [ 31 ]. Growth factor antagonists and growth factor receptor inhibitors are used as effective targeted therapeutic approaches to suppress progression and metastasis of cancer cells and sensitise the cells to killing by cytotoxic anticancer agents. Examples include Her2 antibodies targeting the Her2 receptor in breast cancer, and small molecule inhibitors targeting EGFR, IGF-1R, VEGFR and PDGFR [ 32 ].
One limitation of targeted therapy is drug resistance. There is a significant cross-talk between receptor tyrosine kinase pathways, and the same pathway is usually activated by multiple receptors. Therefore, if the function of one protein located upstream the signaling pathway is inhibited, another protein will most likely compensate the interrupted function. The outcome will still be uncontrolled proliferation [ 29 ].
Targeted immunotherapy has proven successful in many types of cancer. It harnesses the immune system to attack cancer cells. Cancer immunotherapy includes monoclonal antibodies, cancer vaccines, immune checkpoint inhibitors, CAR T cell therapy and immune system modulators. Limitations of cancer immunotherapy include resistance, escape of cancer cells from the immune response and issues related to delivery methods. Some of these issues could be resolved by using nanocarriers as vehicles because of their increased surface areas, targeted delivery, controlled surface and release chemistry, enhanced permeation and retention effect [ 33 ].
3 Nanoparticle advantages in cancer therapy
Nanoparticles (1–100 nm) can be used to treat cancer because of their unique characteristics like biocompatibility, reduced toxicity, increased permeability, improved stability, precision targeting and retention effect. Magnetic nanoparticles in particular offer several appealing features for biomedical applications [ 34 , 35 ]. They have so far been extensively researched and used for magnetic resonance imaging (MRI) as contrasting agents [ 36 , 37 ] particularly for drug delivery [ 38 , 39 , 40 ] and occasionally in conjunction with MRI [ 41 ], as part of the theragnostic concept, cell sorting, regenerative medicine [ 42 ], tissue engineering [ 43 , 44 ] for hyperthermia [ 45 , 46 , 47 ] and for protein purification [ 48 ]. An external magnetic field can be used to remotely control magnetic nanoparticles, which are nanometric-sized compounds with unique magnetic characteristics. Due to their high surface-to-volume ratio, it is possible to graft many molecules onto their surface. Additionally, it encourages their contact with living cells, proteins, viruses and DNA [ 13 , 49 ].
3.1 Magneto-mechanical effect
A newly developing area of research is the therapy of cancer using the magneto-mechanical effect of particles (TMMEP) [ 34 ]. The basic idea behind this method is to exert mechanical pressure on cancer cells in order to cause magnetic particle vibrations, which will ultimately cause the cell death [ 13 , 50 ]. For this, a low-frequency alternating magnetic field is used as shown in Fig. 2 and magnetic particles are injected into the tumour or exposed to cancer cells. The average magnetic moment M of the particle, which depends on the amplitude and direction of the applied field B , is subjected to the magnetic torque M × B and tends to align with the direction of the field in an applied magnetic field B that is assumed to be uniform over the entire volume of the particle. Meanwhile, if the magnetic anisotropy of the particle is high enough (exhibiting strong particle-composition, size and shape dependence), the direction of the magnetic moment M remains almost blocked within the particle, parallel—or making a small angle—with the axis known as the easy axis of magnetisation, or maintained in the easy plane of magnetisation. The action of the magnetic torque becomes magneto-mechanical on particles separated from fluidic solutions or that are only partially attached. Similar to how Earth’s magnetic field affects a compass needle, it tends to reorient the particle until its easy axis or easy plane align with the applied magnetic field direction. Thus, the particles in TMMEP are continuously rotated or vibrated using rotating, or more generally changing, spatially homogeneous magnetic fields. Highly anisotropic particles, such as magnetic discs with ‘magnetic shape anisotropy’ or with ‘perpendicular magnetic anisotropy’, are usually recommended in this method demanding effective magnetic torque [ 51 ].
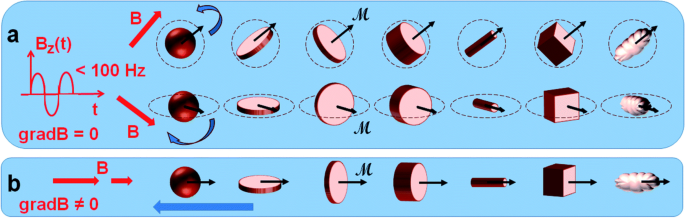
Schematic of numerous types of magnetic particles exposed to variable magnetic fields, thus subjected to magneto-mechanical torques, tending to rotate the particles [ 13 ]
3.2 Organic–inorganic interaction (hybridisation/functionalisation)
The primary determinants of the biocompatibility and uptake effectiveness of the nanoparticles used for cancer therapy are thought to be the physicochemical characteristics of NPs, including surface composition, size, superficial charge and shape. Through the functionalisation of the NP surface, it is feasible to increase the biocompatibility and uptake effectiveness of NPs by altering their physicochemical properties. Theragnostic properties of functionalised nanoparticles have completely transformed the way in which cancer is treated. The method of surface functionalising NPs attempts to enhance and/or add features beneficial for using NPs in medicinal applications. Different kinds of nanomaterials have distinctive chemical characteristics and functional groups that can be exploited in the initial stages of functionalisation since they are accessible on their surfaces [ 52 ]. Typically, the initial stage of surface modification involves adding an organic functional group (R-NH 2 , R-COOH, etc.) that can be used to bind biological molecules using homo- or hetero-bifunctional cross linkers. The most popular linkers for silica NPs are aminosilanes, which adds an amino group to the NPs’ surface in preparation for the upcoming bioconjugation. Crosslinkers containing -SH or -NH 2 groups can be used to functionalise noble metals, such as gold, by reacting with the metal and creating a covalent bond [ 53 ].
These bifunctional linkers, like thio-carboxylic acids have functional groups at the other end that can be used to bind ligands [ 54 ]. By replacing the original surfaces of metal oxides with functional groups like diol, amine, carboxylic acid and thiol useful for the following processes, metal oxides can be easily modified [ 55 ]. The sp 2 hybridised carbon atoms present in considerable amounts in the carbon-based nanomaterials can be used to produce functional groups. It is possible to produce -COOH, -OH and -C = O on the surface of NPs through oxidation that can be further modified, for instance by reaction with the amine group and cycloaddition can introduce various types of functional groups. Table 1 lists various types of nanomaterials, their chemical compositions and/or groups, and the appropriate substances or methods that can be employed for surface modification utilising crosslinkers [ 52 ].
Non-covalent conjugation and covalent conjugation are two alternative methods that can be used to modify the surface of NPs.
The non-covalent approach, which is specifically utilised with metallic and silica NPs is based on a variety of weak interactions, including electrostatic, ionic, van der Walls and hydrophobic contacts, absorption and hydrogen bonding [ 56 ]. Non-covalent bonds have the benefit of being quite straightforward and unaffected by the structure of the molecules being utilised or how they interact with biological targets. However, various factors like pH and ionic strength can quickly affect non-covalent transformations. Depending on the nature of the NPs, various other methods can be used to create the covalent bonding mechanisms [ 57 ]. Additionally, utilising successive functionalisation, this approach enables alterations at many levels [ 58 ]. In order to execute the theragnostic approach, this methodology can be used to create structures with various purposes, such as diagnostic and therapy. Usually, different linker molecules can be used to covalently attach ligands to the surface of NPs. PEG is a prime example. It can be synthesised with certain functional groups at the ends and utilised as homo-bifunctional or hetero-bifunctional linkers to carry out a variety of functionalisation activities.
The large number of scholarly works devoted to this subject attest to the effectiveness of NP surface modification in enhancing uptake and biocompatibility. Due to the modification of surface charge and the inactivation of reactive chemical groups that may impact cellular membrane stability, these findings show that conjugating molecules on the surface of NPs can effectively improve biocompatibility both in vivo and in vitro (Fig. 3 ). Additionally, the inclusion of certain molecules can improve the passive and active uptake of NPs, minimising in vivo systemic toxicity and enabling very precise therapy and/or diagnosis. Both covalent and non-covalent methods can be used to bind compounds to the NP surface. In order to improve uptake and perform active targeting, the former is usually applied to bind proteins, aptamers, antibodies, and peptides, while non-covalent interactions are typically used for drug loading and for all molecules that must be released in cells [ 52 ].
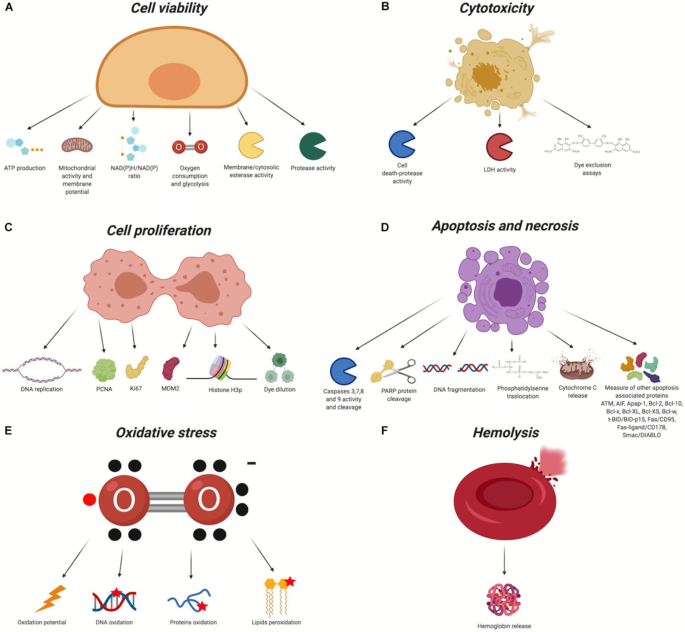
Biocompatibility evaluation assays. In red, the chemical groups that react with the nanomaterial. X, organic/inorganic free chemical groups used to bind the ligands. A Cell viability. B Cytotoxicity. C Cell proliferation. D Apoptosis and necrosis. E Oxidative stress. F Haemolysis [ 52 ]
3.3 Clinical relevance
The benefits of functionalised nanocarriers, such as their enhanced permeation and retention, passive targeting capacity, capacity to load drugs for targeting modification, and high surface-to-volume ratio, made it possible to conduct a number of clinical research studies focusing on combined therapy [ 59 ]. For instance, Katragadda et al. [ 60 ] showed a safe and effective nanosized formulation for the delivery of paclitaxel and 17-AAG combination therapy, which has only yielded modest results in phase 1 clinical studies. Novel nanoparticles based on polymeric microspheres loaded with two anticancer medications were created by Liu et al. [ 61 ] for pulmonary transport. Studies on in vivo pharmacokinetics and biodistribution of the microspheres revealed that they had a long circulation time and might develop in the lung.
Araujo et al. [ 62 ] (Table 2 ) described tyrosine kinase inhibitors used in clinical practice for treating solid tumours. SRC is a tyrosine kinase that plays a crucial role in the oncogenic and bone-metastatic processes, making it a viable therapeutic agent for the treatment of solid tumours. One of the SRC inhibitors now under development and the current findings are helpful in determining if targeting SRC is an effective therapeutic approach. Experiments conducted in vivo and in vitro revealed that the NPs exhibit superior antitumour effect and lower toxicity [ 63 , 64 ].
3.4 Modelling and simulation studies
It is known that NPs have potential to ameliorate cancer treatment through to their highly developed functionalisation and their ability to accumulate in specific tumours. Yet, one common issue is the gap of knowledge on understanding the impact of NP designs (surface functionalisation, shape and size) to reduce the complication on transport barriers in the body [ 65 ]. Increased computational modelling methods, along with advanced multiscale simulations of NPs, tumours, and the biological transport barriers that influence them, permits us to explore the influence of a variety of designs in biologically related scenarios [ 66 ]. The development of effective NP cancer therapies can be accelerated with the use of in silico models [ 66 ] discussed further.
3.4.1 In silico models of tumours
Continuum, discrete and hybrid are the current mathematical methods used to model tumours. However, continuum models (which uses ordinary and partial differential equations [ 67 ] and are best suited to describe global changes to a tumour) are restricted in their ability to re-create cellular interactions, heterogeneity and other features which discrete modelling method perform best. With the discrete method, it can model realistic heterogenous development of tumour, it can provide critical insight on angiogenic vessel growth and/or the emergence of cellular resistance [ 66 ], by following the simple rules leading their lifespan and interaction with local environment and other agents. Both methods provide crucial information but due to their limitations, researchers have proposed to combine them to obtain the advantages of both, the hybrid methods, which develops on discrete models but merge them with gradients of variables, modelled using continuum equations [ 68 ].
Various reviews are made on these topics which shows recent developments in hybrid models [ 69 ], discrete models [ 70 ] and multiscale modelling [ 71 , 72 ] of cancer (Fig. 4 ).
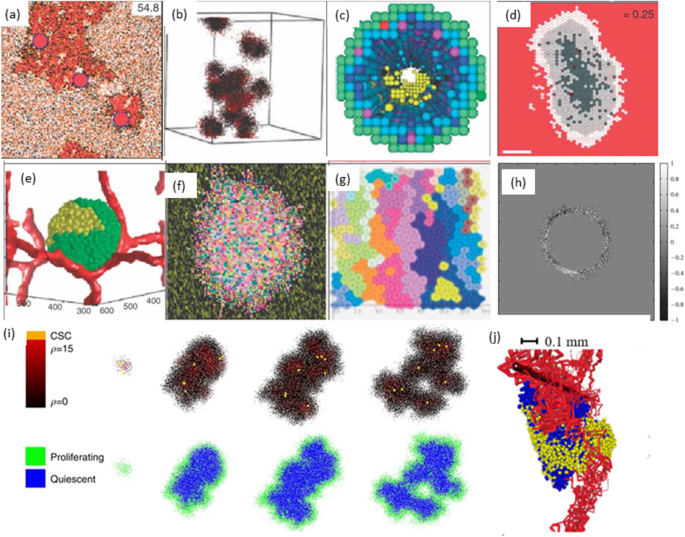
Schematic illustration of hybrid models of tumour growth developed by various researchers [ 73 ]. a In prostate ducts simulation of hybrid method of tumour invasion [ 74 ]. b Hybrid cellular method of 3D tumour self-metastatic [ 75 ]. c Square-grid cellular, in situ simulation of 3D model of ductal carcinoma [ 76 ]. d Hexagonal cellular mechanism of 2D spheroids tumour [ 77 ]. e Potts model simulation of 3D vascularised spheroid tumour [ 78 ]. f Potts model of a 2D spheroid tumour in a heterogeneous environment [ 79 ]. g Particle model with Voronoi triangulation simulation of 2D tumour [ 80 ]. h Simulation of the extracellular matrix in models of tumour growth [ 81 ]. i Simulation of cancer growth with multiscale agent-based modelling [ 82 ]. j Vascular tumour growth (blue: proliferating tumour cells, yellow: quiescent tumour cells) [ 83 ]
3.4.2 Modelling of transport barriers for NP delivery
To use NPs as a drug delivery pathway requires the particles to pass through from point of entry to their designated biological target in the body [ 66 ]. To overcome transport barriers, NPs require to travel through the vasculature [ 84 , 85 ], extravasation [ 86 ], avoiding uptake by the reticulo-endothelial system [ 87 ], progression through the tumour tissue [ 88 ], endocytosis [ 89 ] and delivery to the relevant part of the cell [ 90 ], as shown in Fig. 5 . It is known that when NPs are injected into the blood stream, there is a high probability for escaping into the tumour site through various arrival ports if the circulation time is prolonged [ 66 ]. These could be influenced by the design of the NPs including the charge, shape and size. When NPs are of size less than 5 nm, the kidneys rapidly clear them [ 91 ] and NPs greater than 100 nm have a high chance to be discovered and cleared by macrophages [ 92 ]. However, NPs between the two size will be transported by macrophage uptake [ 93 ].
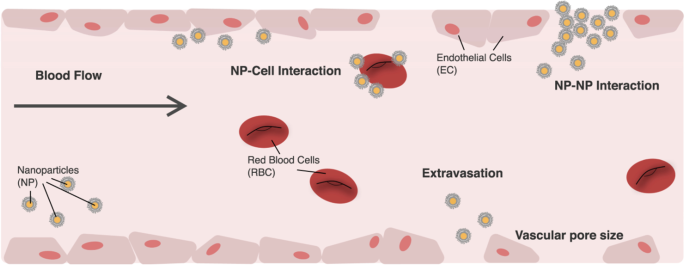
Image illustrating NP transportation across the vascular chamber. Consideration when modelling NPs [ 66 ]
In the past, molecular dynamics (MD) simulations have been used to investigate the effect of NPs charge, shape, size, pH-stability and the protein corona formation influencing the NP transportation. For instance, Lopez et al. [ 94 ] used a coarse-grained model method to examine the adsorption effect of blood plasma proteins onto NPs. Shao et al. [ 95 ] used a coarse-grained approach to model the protein adsorption on NPs and simulated it using discontinuous molecular dynamics simulations. Maleki et al. [ 96 ] used single-walled carbon nanotube and multi-walled carbon nanotubes to study the pH-sensitive loading/releasing of doxorubicin. Additionally, other factors influencing the effect of NP transportation across the vascular are the margination, as the NPs can easily evade from the porous tumour vessel to the blood cells by preventing their interactions, as shown in Fig. 5 . This was demonstrated in the study by Müller et al. [ 97 ] by combining the use of computational fluid dynamics and dissipative particle dynamics (DPD) to observe the margination effect. It was examined that in the core region within the red blood cells, NPs smaller than 200 nm are entrapped, but when NPs are larger than 500 nm the margination effect can be exploited as shown in Fig. 6 [ 65 ].
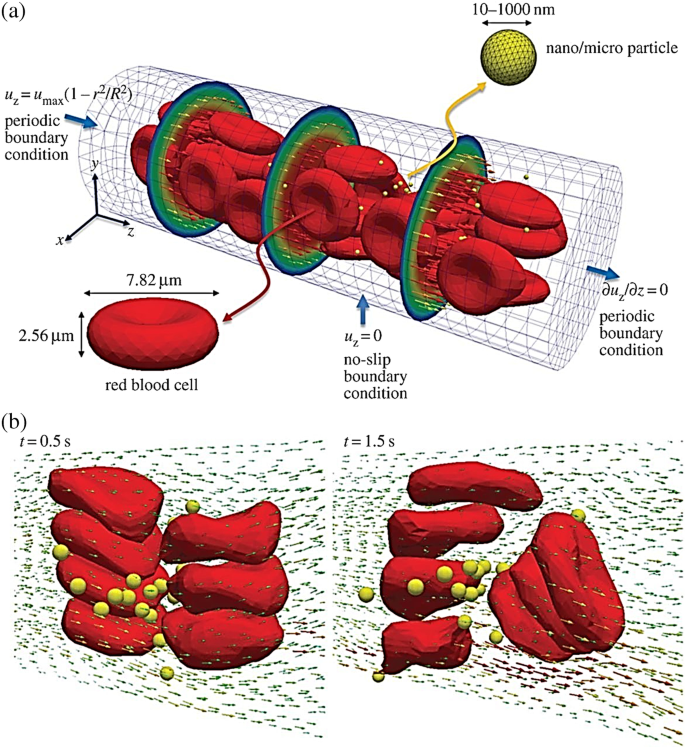
Image illustrating the microcirculation behaviour of the effect of NP shape and size. a Beginning structure of deformable red blood cells and spherical rigid particles scattered and b formation of complex flow field across the particles [ 65 ]
3.4.3 Tissue penetration and NP internalisation
One significant challenge for NP-based therapies is the prediction of NPs depth, their ability to penetrate into the tumour and the location of their accumulation [ 66 ]. With the use of in silico method such impediment can be better understood as it provides a mean of understanding the tumour penetration. For instance, Wu et al. [ 98 ] used a combination of agent-based modelling and fluid dynamics to investigate the influence of the interstitial fluid pressure on the lymphatic systems, the blood and their effect on drug delivery. This study showed the impact of increasing the drug distribution which resulted on a decrease respond on the lymphatic, see Fig. 7 . For further investigation on the heterogeneous distribution of NPs, Wijeratne et al. [ 99 ] focused on investigating the conditions of the tumour for an improved NP drug delivery using a three-dimensional model continuum approach.
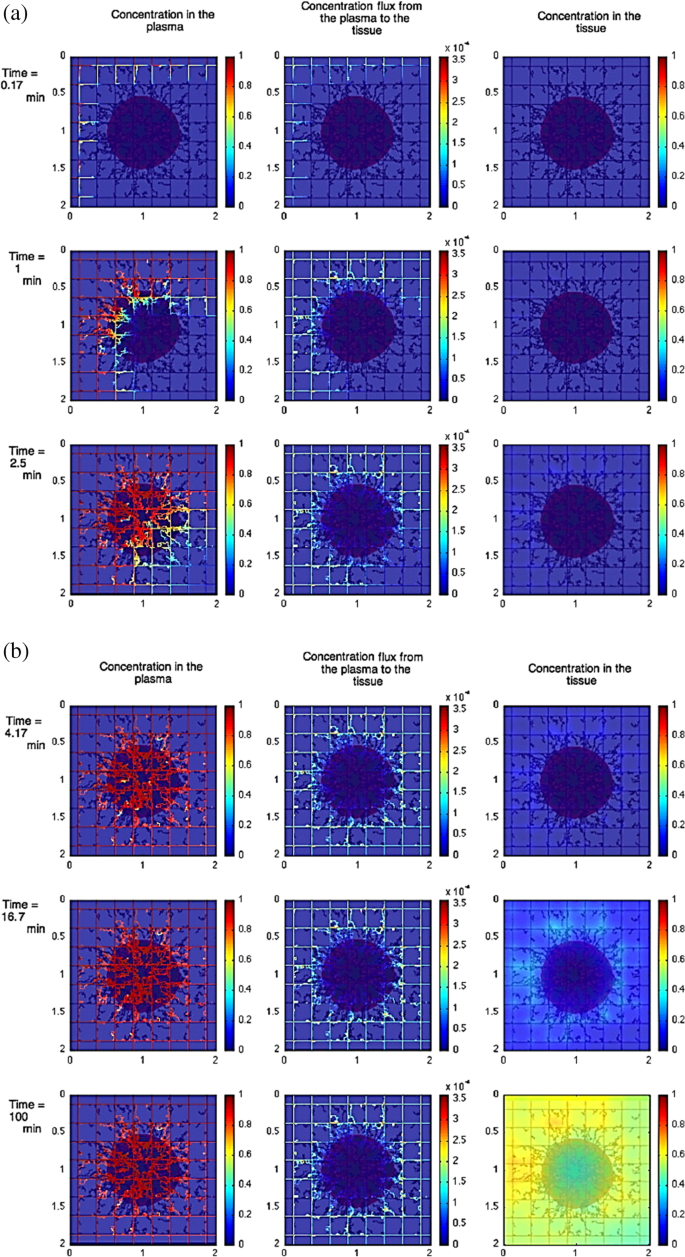
Image illustrating the effect of constant tumour tissue injection at the beginning vs later times. a The beginning time where red colour shows tumour with viable tissue, blue shows the hypoxic and brown shows the necrotic. b Later times, where left column shows the concentration in the plasma, the middle shows the concentration flux from plasma to the tissue, and the right column shows the concentration in the tissue [ 98 ]
In terms of NP internalisation, Li et al. [ 65 ] presented early stages of receptor-driven endocytosis of NPs by introducing a novel dissipative particle dynamics technique. They examined the usage of multiscale modelling techniques to clarify how NP shape, surface functionalisation and size has an influence on their distribution by targeted cell in the microvasculature and following their internalisation. Quantitatively distinct behaviours of these PEGylated NPs were observed where the size of the PEGylated NPs with spherical core had a diameter of around 8 nm and a tethered chain with a molecular weight of around 838 Da. As presented in Fig. 8a , the PEGylated NP was initially absorbed on the surface of the cell membrane when the grafting density was 0.2 chains nm −2 . After more than 2000 ns, the PEGylated NP was unable to penetrate the cell’s interior as it was still on the membrane’s surface. In contrast, as shown in Fig. 8b , when the grafting density was raised to 1.6 chains nm −2 , the cell membrane began enfolding the PEGylated NP, followed by the membrane—extruding phase where NP was eventually completely encircled by the membrane and formed a complex of NP-membrane interactions. As such, to deliver the targeted drug, the PEGylated NP can be transported into the unhealthy cell.
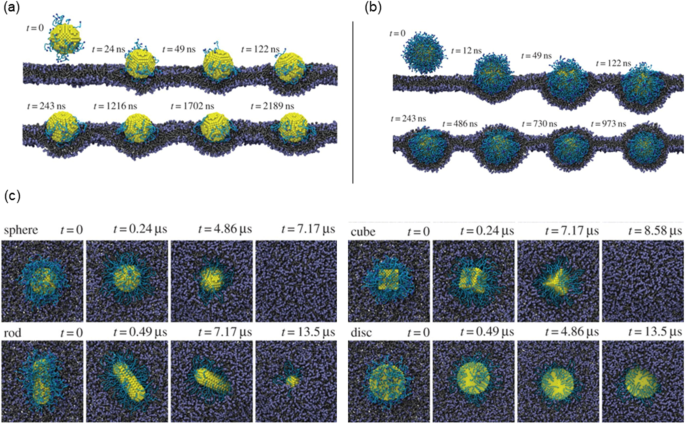
Image illustrating the NPs shape and surface functionality on the internalisation behaviour. a Different effects of PEGylated NP shape with grafting densities of 0.2. b Grafting densities of 1.6 chains nm. −2 . c The effect of different shaped PEGylated NP internalisation impact process [ 65 ]
As demonstrated in Fig. 8c , the NP models presented are spherical, rod-like, cubic and disc-like. Figure 8c shows that the shape of NPs plays a crucial role in designing the parameter for internalisation. The study conducted by Li et al. [ 65 ] compared different NP shape designs (first and second generation) that has similar surface areas for their cores and discovered that under a constant grafting density of 0.6 chains nm −2 , the most efficient internalisation by the cell was observed to be as follows: the spherical, the cubic and lastly the rod-like NPs. However, it was observed that the disc-like NP was only detected on the cell membrane’s surface after being simulated at the same duration as the rod and resulted that it could not be ‘internalised’ as others. This emphasised that surface functionalisation plays another important role. Despite the fact that distinct NP shapes have various surface area to volume ratios, to distinguish the effect of NP size from the surface is challenging, particularly when little NP shapes are investigated. However, with accurate defined condition, there is a potential for an unambiguous investigation of these shapes that influences NPs during endocytosis, that is only possible through computer simulations [ 65 , 100 ].
3.5 Identification of the best NPs for targeted delivery
To maximise the mechanical reaction of the particles with the biological tissue, TMMEP characteristics such as structure, dimension and magnetic properties of the particles, frequency and amplitude of the applied magnetic field must be determined. For the particles to be utilised in vivo and subsequently for therapeutic purposes, the biocompatibility of the components that constitute the particles remains a key factor in their composition. Due to their biocompatibility, iron oxides like magnetite (Fe 3 O 4 ) and maghemite (g-Fe 2 O 3 ) are excellent choices, even though they often display non-negligible cytotoxicity [ 50 , 101 , 102 ]. According to the research by Ling [ 101 ] and Goiriena-Goikoetxea [ 50 ], bare iron oxide nanoparticles in particular are known to cause reactive oxygen species (ROS), which is thought to be one of the primary causes of nanotoxicity. Moreover, when iron oxide nanoparticles are covered in a biocompatible layer, based either on inorganic shells, like gold, silica, or tantalum coatings, or on a wide range of biocompatible organic shells, depending on the nanoparticle core type and the intended applications, their toxicity is significantly reduced. Alternatives that works well include magnetic materials made of nickel cobalt or NiFe alloys [ 13 ]. A gold coating or polyelectrolytes, for example, or limited dissolution should be ensured to maintain the biocompatibility of particles made of these hazardous metals. Surface functionalisation of particles are being required to precisely target a cell type or to improve particle dispersion in fluids [ 103 , 104 ].
To achieve this, a layer of gold is deposited on the surface of the NPs, enabling the grafting of organic molecules via thiolates’ self-assembly on the gold surface. These thiolates frequently have functional terminal groups and a polyethylene glycol (PEG) spacer. The functional group enables the attachment of biomolecules for precise targeting while ensuring biocompatibility, while the PEG spacer promotes particle stability [ 105 , 106 , 107 ].
Magnetic particles are typically injected directly into the proposed area in vivo research [ 34 , 37 ], preventing bloodstream absorption. Organising an injection of the particles via the venous route for prospective therapeutic applications is still challenging as long as the functionalisation strategies for delivering to the zone of interest are not efficient. The size and structure of the particles with the intervention of suitable functionalisation could be crucial for their circulation in the blood flow, at least close to the tumour site, even though venous injection remains difficult for targeting the tumour [ 39 , 108 ]. Due to the phenomenon of flow margination, anisotropic shapes like nanodiscs, nanorods, and nanowires should be more advantageous than spherical ones. Additionally, if magnetically triggered by a different magnetic field, anisotropic particles enter the tumour site more effectively due to the EPR effect [ 13 ]. Some of the effective materials for these applications are discussed in the following sections:
Iron oxide nanoparticles (IONPs)
Latest reports have highlighted new IONP therapeutic potentials [ 109 ]. IONPs produce mechanical stresses and torques in response to low-frequency magnetic fields, which are transferred to the materials with which they come into contact. These torques can be utilised to modify molecules, improve gene transfection or tissue engineering, control calcium entry within cells, trigger protein degradation, activate enzymes or kill cancer cells (Fig. 9 ) [ 13 , 110 , 111 , 112 , 113 , 114 ]. Sara et al. [ 115 ] demonstrated that pancreatic cancer-associated fibroblasts used as a model may be killed by a superparamagnetic iron oxide nanoparticle as small as 6 nm. An extensive analysis of magnetic field amplitude, frequency and type (rotating vs. alternating) revealed that the rotating low-amplitude low-frequency magnetic field (1 Hz and 40 mT) had the best effectiveness, reaching a 34% ratio in inducing cell death. Interestingly, cell death does not occur at the largest amplitudes of the magnetic field.
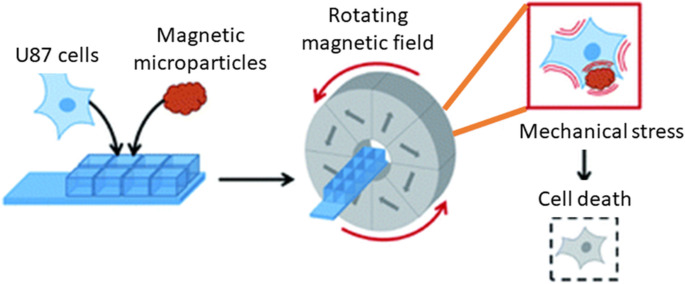
IONPs for anti-cancer therapy [ 116 ]
Modern kinetic Monte-Carlo calculations that could determine the torque experienced by magnetic nanoparticle assemblies explained these characteristics and agreed with cell killing investigations. Simulations revealed that the force the nanoparticles produced once they were ingested into the lysosome was about 3 pN, which is, in theory, insufficient to cause a direct membrane breach. Additional biological explanations for cell death have been investigated. Lysosome membrane permeabilisation and the release of lysosome content were caused by the mechanical activation of magnetic nanoparticles, and cell death was mediated by a lysosomal pathway that depended on cathepsin-B activity. By providing proof-of-concept that ultra-small nanoparticles can disrupt the tumour microenvironment through mechanical forces produced by the mechanical activation of magnetic nanoparticles upon exposure to a low-frequency rotating magnetic field, this study opened up new therapeutic possibilities for cancer (Fig. 9 ) [ 115 ].
Fe/Ni alloy
Kim et al. [ 117 ] used comparatively large nanoparticles with a 20/80% Fe/Ni alloy coated with gold in a disc geometry (60 nm thick and 1 m in diameter) in another study on cancer therapy. Human glioblastoma cell membranes were the target of functionalised anti-human-IL132R antibodies on the gold surfaces of the discs. They exposed the cells and particles to homogeneous AC magnetic fields with frequencies (10–20 Hz) and relatively low intensities (8 kA/m). The glioblastoma cells underwent apoptosis, and it was proposed that the discs aligned in the field and then slightly misaligned when the field was altered, harming the cell membranes to which they were linked and further resulting in an ionic signal that led to cell apoptosis [ 118 ].
Graphene quantum dots (GQDs) core–shell composite
In a study published by Fangjie et al. [ 119 ], a multimodal therapeutic system was demonstrated to be significantly more lethal in the destruction of cancer cells than a single dimension of nanotherapy, whether it be photothermal or photodynamic. Hollow magnetic nanospheres (HMNSs) were created to combine the benefits of photothermal and magnetomechanical cancer therapies. The cancer cells were structurally and physically eliminated by these combined stimuli, and their parameters were noticeably different from those caused by other therapies. As a core–shell composite, HMNS/SiO 2 /GQDs, the silica shells were also applied to HMNSs and coupled with carboxylated graphene quantum dots (GQDs). The composite was additionally stabilised using liposomes and loaded with the anticancer drug doxorubicin (DOX).
In a cooperative and multilateral manner, the multimodal system was able to destroy cancer cells via four distinct therapeutic mechanisms namely, magnetic field-mediated mechanical stimulation, photothermal damage, photodynamic toxicity, and chemotherapy. The innovative nanocomposites with combined mechanical, chemo and physical properties provide a different method for significantly enhancing the effectiveness of cancer therapy (see Fig. 10 ) [ 120 , 121 ].
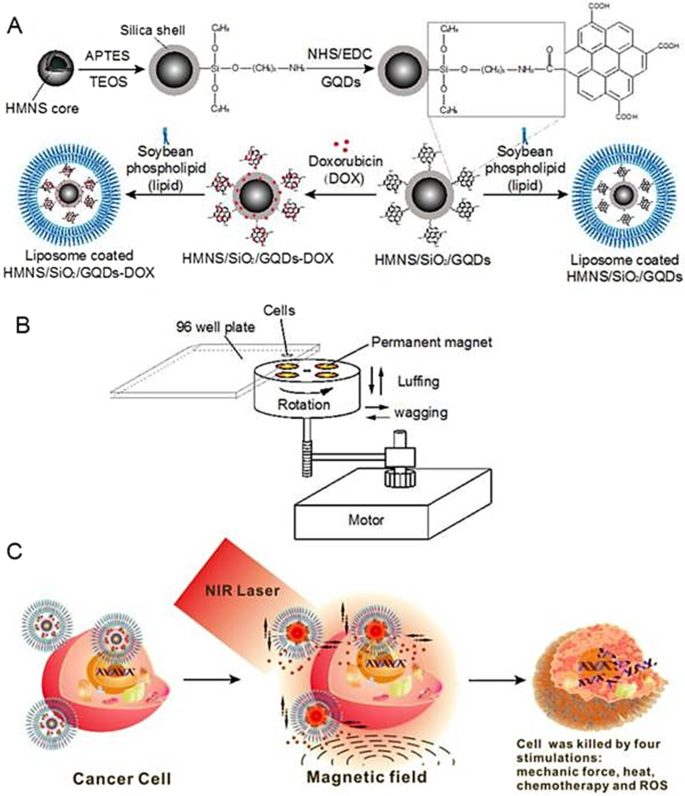
Schematic diagram of doxorubicin hydrochloride (DOX)-loaded nanocomposites [hollow magnetic nanosphere (HMNS)/SiO 2 /graphene quantum dots (GQDs)-DOX] that kill a cancer cell upon exposure to a dynamic magnetic field and near-infrared (NIR) laser irradiation. A The formation of liposome-coated HMNS/SiO 2 /GQDs-DOX nanocomposites. B The experimental setup of the dynamic magnetic field. Cells (96-well plate) are placed 1.4 cm above the magnets possessing a magnetic strength of 45.3 ± 0.5 mT and a rotation and swing of 2000 r/min. C The nanocomposites obtained exhibit multimodal therapy (mechanical force + heat + chemotherapy + reactive oxygen species) in cancer treatment when treated with an external magnetic field and NIR laser irradiation [ 119 ]
Polymeric nanoparticles
Polymeric nanoparticles (PNPs), mAb nanoparticles, extracellular vesicles (EVs) and metallic nanoparticles are broadly researched nanoparticles (NPs) for targeted delivery (Table 3 ). Colloidal macromolecules with submicron sizes of 10–1000 nm is referred to as PNPs. PNPs act as drug transporters for chemical medications, enabling their prolonged release to the intended malignant areas [ 122 , 123 ]. A nanocapsule or nanosphere is created when drugs are insulated within or adhered to the surface of nanoparticles. Over time, nanoparticle components have undergone changes. Nanoparticles were first created using nonbiodegradable polymers such as polymethyl methacrylate (PMMA), polyacrylamide, polystyrene and polyacrylates [ 124 , 125 ]. Polymeric nanoparticles produced by these materials need to be cleared up in a suitable manner to prevent toxicity and chronic inflammation. It is now possible to get these polymer-based nanoparticles destroyed, expelled, or physically removed from tissues without accumulating them to toxic levels. Biodegradable polymers have been created to optimise medication release kinetics, minimise toxicity, and boost biocompatibility. These polymers include chitosan, alginate, gelatin, albumin, poly(lactic acid), poly(lactic-co-glycolic acid), poly(amino acids) and poly(-caprolactone). These enhanced polymeric nanoparticles offer distinct benefits as a result of their characteristics and topologies. PNPs contribute to greater stability for unstable pharmacological compounds. In contrast to free drugs, PNPs have a higher loading capacity for chemical pharmaceuticals and offer optional distribution routes like oral and intravenous. Drugs’ capacity to resist degradation aids in reducing unintended toxicity to healthy tissues. For example, chemotherapy has used PNPs loaded with cisplatin such as dexamethasone or tocopheryl succinate, which inhibits cisplatin-induced ototoxicity [ 126 ].
4 Nanoparticles delivery for cancer treatment
4.1 targeting cancer cells.
The foremost aim of chemotherapeutic drugs for targeting cancer cells is to kill the cancer cells and to minimise the side effects [ 131 ]. For specific targeting of cancer cells using nanoparticles and medication delivery in cancer therapy, there are primarily two approaches used. Researchers are constantly working to improve various medications using nanocarriers to target cancer cells in a specific way.
In order to accumulate nanoparticle delivery systems including liposomes, polymeric-drug conjugates, micellar systems and polymeric NPs, passive targeting mostly relies on the physiological properties of the tumour. Rapidly developing tumours with enhanced vascular permeability and compromised lymphatic drainage frequently cause cancer and increase the permeability and retention (EPR) effect of nanosystems in that disease. Active cancer-targeting uses adding certain moieties to improve the delivery of nanoparticle systems to the tumour site [ 132 ]. Active targeting makes use of the highly expressed surface receptors on cancer cells by sustaining their engagement with the targeting ligands. In the earlier investigation on the active targeting of nanoparticles, several ligands composed of proteins (antibodies), nucleic acids, peptides or carbohydrates were employed [ 133 ]. These ligands can quickly attach to receptors that are expressed on cancer cells, mediating the binding and accumulation of NPs at the tumour site by receptor-mediated endocytosis and enabling the delivery of drugs for therapeutic action (Fig. 11 ). The two key criteria that determine the efficiency of active targeting are targeted specificity and deliverability. The nature and makeup of NPs directly affect the deliverability of nanoparticles [ 134 ]. The requirement that the desired NPs interact with the target antigen in close proximity makes developing active NP targeting a difficult task. Active targeting of nanoparticles for drug delivery enables effective encapsulation of NPs by target cells, and ongoing research is being done to demonstrate the effectiveness of drug delivery (Fig. 11 ) [ 135 ].
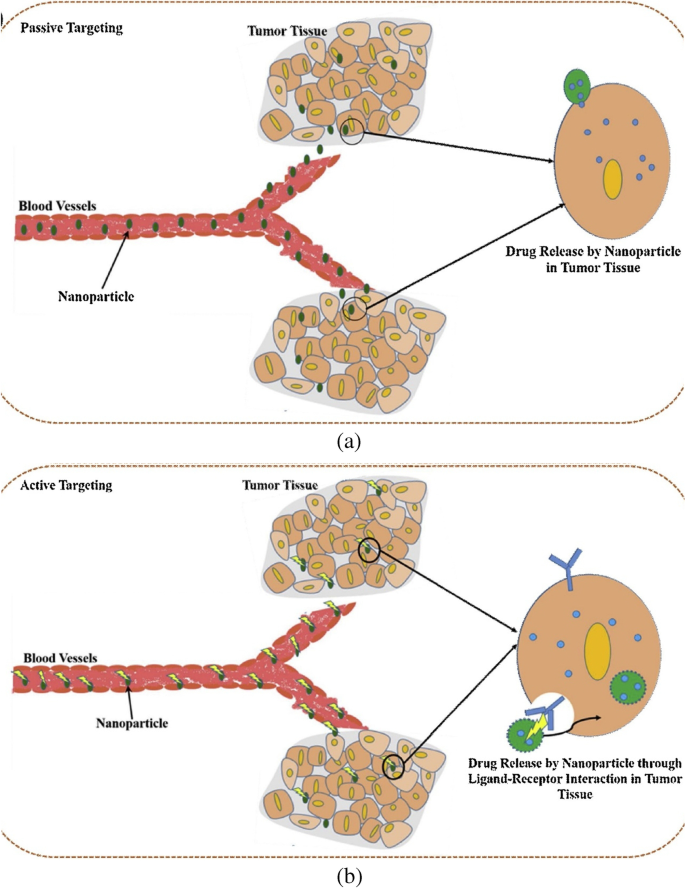
Mechanisms of tumour targeting by nanoparticles. a Passive targeting. b Active targeting [ 135 ]
4.2 Targeting the microenvironment
Tumour microenvironment has been implicated in cancer growth and metastasis. With a better understanding of tumours, it is now known that they develop in a microenvironment that is very heterogeneous, complex, and made up of TAMs, CAFs, immune cells, and ECM components. Recent research shows that one key tactic in preventing cancer growth, invasion, and metastasis is altering the tumour microenvironment and its aberrant composition. With the development of nanotechnology in the drug delivery field, creative strategies to combat the cancer threat have emerged [ 136 , 137 ]. However, it has emerged that the complexity of the tumour microenvironment has a significant, though debatable, impact on the control of nanochemotherapeutics’ higher tumoural penetration and, consequently, their biological effects [ 138 ]. To address this challenge, techniques have been developed employing nanotechnology that either target the tumour vasculature, change the stromal characteristics, or make use of the chemical microenvironment of the tumour to overcome acquired resistance caused by the tumour milieu. Therefore, by causing perturbations in the tumour microenvironment, nano-chemotherapeutics can change the way that drugs are delivered to tumours. Therefore, nanotechnology offers a flexible tool by permitting the delivery of either a solitary or combinations of chemotherapeutics together with numerous targeting ligands to specifically target overexpressed receptors or enzymes or a reductive environment, a characteristic of the tumour microenvironment [ 139 , 140 ]. This strategy offers target specificity, resulting in effective therapy with little unintended negative side effects. Additionally, the development of a combination therapeutic and diagnostic method known as nanotheranostics is made possible by our improving understanding of how to target the tumour microenvironment utilising nanotools [ 141 , 142 ]. Various approaches combining nano-chemotherapeutics with radiation and other related therapies will transform into a viable strategy for combating drug resistance since there are an increasing number of ongoing clinical trials on nanotherapy. Overall, it can be said that nano-chemotherapeutics do show promise in the early phases of cancer since these highly multifunctionalised nanocarriers enable delivery of chemotherapeutics either by utilising the tumour microenvironment or improved permeability and retention (EPR effect) as shown in Fig. 12 [ 143 ].
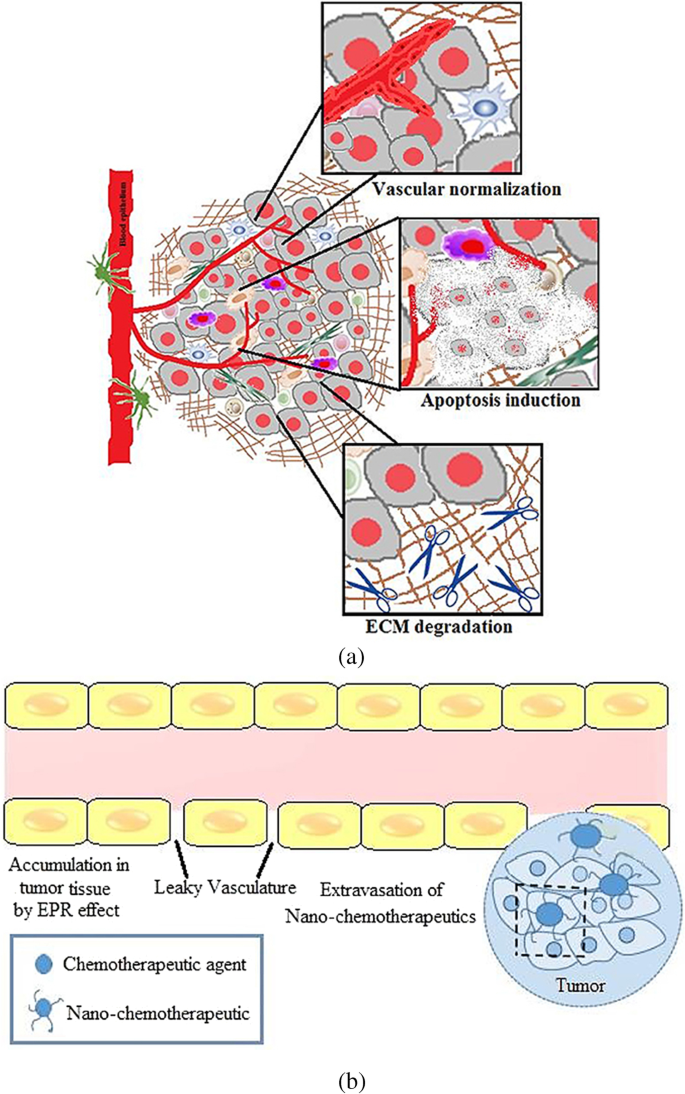
a Tumour microenvironment priming. b EPR effect in a tumour microenvironment [ 143 ]
4.3 NP and immunotherapy
Cancer immunotherapy, which activates body’s own immune system has become a viable method for treating a variety of cancers. By triggering a significant immune reaction against the tumour, this treatment not only destroys tumour cells but also stops them from getting back. High immune-mediated toxicity, ineffective and untargeted delivery of cancer antigens to immune cells, and off-target side effects are only a few of the daunting obstacles that therapeutic cancer immunotherapy must overcome. However, nanoparticle-mediated proposed system various ways to get beyond those restrictions and can thereby increase the effectiveness of immunotherapy. The primary problem in cancer immunotherapy is to deliver antigens for the subsequent development of an immune response [ 144 ]. To cause naive T-cell differentiation and activation as well as antigen presentation by the APCs for later CD8 + and CD4 + T cell activation, sufficient antigen and pretreatment are needed [ 145 , 146 ]. APCs are immune cells that deliver antigens to the class I and class II MHC molecules on the surface of killer cells so that they can connect with T cell receptors. There are four main types of APCs in our immune system: DCs, B cells, macrophages and monocytes. The vascular endothelial cells, thymic epithelial cells, fibroblast, pancreatic cells and glial cells are further amateur APCs that work in certain circumstances [ 147 ]. In recent years, scientists have focused increasingly on developing immunostimulatory NPs that can be efficiently internalised by APCs. By doing so, they can transport cancer antigens and adjuvants to target cells selectively and stimuli-responsively, triggering an antigen-specific immune response [ 148 , 149 , 150 ]. These NPs enable the required immunostimulatory effects by allowing serum proteins to bind to their surface and form a corona that interacts with a variety of receptors [ 151 ]. In addition to targeted delivery, NPs could shield the cargo molecules from bioactivity loss during circulation and prevent adverse effects that are not intended [ 152 ].
Different types of cancer have responded well to cancer immunotherapy; nevertheless, a small percentage of individuals with particular tumour types show negative responses to immunotherapy, which restricts its widespread clinical use. According to the data available, hot tumours or tumours in the immune clearance stage, are virtually always cancers with several mutations, including melanoma, kidney cancer, NSCLC and hereditary rectal cancer. These tumours respond exceptionally well to immunotherapies, including PD1 inhibitors, which can greatly increase the time that cancer patients survive after diagnosis. Additionally, solid tumours like late-stage gastrointestinal tumours, triple-negative prostate cancer, malignant lymphoma, and some haematological cancers can easily induce tumour dormancy that encourages cancer spread and recurrence. These tumour types respond poorly to conventional treatment procedures; however, immunotherapy can eradicate these latent cancer cells by inducing an immune response. Other cancers, such as glioblastoma, pancreatic, prostate, and ovarian cancers, are mostly cold tumours at the stage of immune escape. The therapeutic impact of immunotherapy is diminished by this tumour immune escape effect. It should be emphasised that immunotherapy does not immediately reduce tumour size in people with advanced cancer. Immunotherapy as a post-operative adjuvant therapy can prevent recurrence and successfully prolong patients’ lives while maintaining their quality of life (Table 4 ) [ 153 ].
4.4 NP and drug resistance
The most frequent reasons for chemotherapy failure, innate or acquired drug resistance, severely restricts the therapeutic results of chemotherapy. Recent developments in nanotechnology have offered substitute methods for addressing tumour medication resistance. Drug-loaded nanoparticles (NPs) are superior to free drug forms in a number of ways, including decreased cytotoxicity, prolonged blood circulation and greater tumour accumulation. However, due to the multiple pathophysiological hurdles present in the tumour microenvironment, such as intertumoral dispersion, penetration, intracellular trafficking, etc., nanoparticulate medicines have currently only minimally increased the overall survival rate in clinical studies. To increase the therapeutic effectiveness of nanomedicine, smart NPs with stimulus-adaptable physical and chemical characteristics have been developed in considerable detail. At the level of the tumour tissue, the drug resistance mechanism is highly intricate. Commonly regarded as the main drug resistance factors (Fig. 13 ), tumour heterogeneity, tumour microenvironment (TME), drug transporter and multidrug resistance, cancer stem cells (CSCs), epithelial-mesenchymal transition (EMT) and tumour metastasis all contributes to the off-target effect in the use of chemotherapy. Additionally, drug efflux caused by drug transporters compromises the delivery of cellular chemotherapeutics, resulting in low therapeutic doses [ 154 , 155 , 156 ]. Furthermore, the survival compensation effect may be strengthened by low pH, a hypoxic tumour microenvironment, and other anti-apoptotic chemicals [ 157 ]. Other resistant factors include gene mutations and genomic instability, epigenetic alterations including DNA methylation and protein acetylation, suppression of apoptotic signalling, and overexpression of anti-apoptotic molecules, in addition to the five medication resistance factors described [ 158 ].
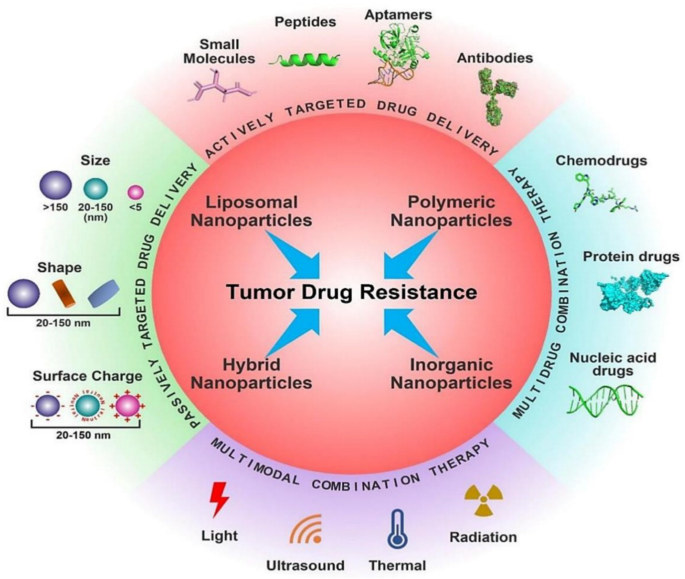
Different nanotherapeutic approaches for overcoming cancer drug resistance [ 158 ]
The emergence of MDR has grown to be a significant issue in oncology and reduces the efficacy of chemotherapy in the management of many metastatic tumours. Resistance to numerous medications that are structurally and functionally different from the initial drug is highlighted by multidrug resistance. Increasing evidence suggests that resistance to cancer treatments is a difficult and complex process that needs serious attention right away and a thorough knowledge of the molecular principles [ 120 ]. According to the data currently available, medication resistance can be classified as intrinsic or extrinsic depending on the variables involved. Drug resistance can either be inherited or acquired, depending on the type of cancer. The doctors have a huge treatment challenge as a result of these two types of pharmaceutical resistance (inherited vs. acquired and extrinsic vs. intrinsic). Although cancer cells and their surroundings include resistance-mediating components which leads to resistance development. On the other hand, extrinsic or acquired drug resistance may appear while treating cancers that were previously sensitive to cytotoxic medications. Extrinsic resistance could arise through a variety of adaptive responses, such as the modification of signaling pathways, activation of alternative signaling pathways, and increased expression of the therapeutic target, and it would contribute to offset the therapeutic effects of previously used medications [ 159 ]. Additionally, the regulation and reprogramming of many metabolic and cellular physiological pathways, the tumour microenvironment, stemness, and cancer resistance are all impacted by the manipulation of signaling pathways.
Proinflammatory cytokines, chemokines and reactive oxygen species (ROS), among other elements, are crucial in the control of many signaling pathways. Overall, a variety of factors, causes, and mechanisms that are linked to drug resistance in various cancers (incorporating extrinsic and intrinsic resistance) include altering the tumour microenvironment, tumour heterogeneity brought on by cellular changes, reduced drug uptake, drug inactivation, altered drug targets, drug efflux, inhibiting cell death, changing the DNA repair process, epigenetics, inhibiting apoptotic pathways and autophagy and epithelium [ 160 , 161 ]. Due to this, it is important to comprehend the phenomenon of cancer resistance as well as the fundamental signaling processes resulting from a variety of exogenous and endogenous elements in order to create future therapeutic interventions or combination therapies for diverse cancers. Table 5 shows a list of various factors that contribute to treatment resistance in cancers are shown.
5 Future direction(s)
Artificial intelligence or AI refers to the simulation of human intelligence processes by machines, especially computer systems. In the last ten years, the field of AI has made much progress in vision, image/speech recognition and generation, planning, and decision-making. This has increased the role and importance of AI in all areas of healthcare most particularly in diagnosis, drug discovery and basic life science research.
However, the use of AI in healthcare is associated with challenges like lack of quality medical data and the gaps between the technical accuracy of AI tests and clinical usefulness. This has led to unreliable results many a times. Despite these challenges, AI is being increasingly used and trusted by healthcare professionals and industry. In the past years, AI has made unique contributions in anticancer drug development and treatment [ 171 , 172 , 173 ]. For instance, when it comes to formulating the most suited treatment for a patient, there is a possibility for doctors to choose unsuitable treatment where the patient is likely to miss on vital treatments opportunities which could result in delaying patient’s condition [ 174 ]. It is evident that AI has further capabilities of analysing, detecting, and processing information that humans are limited to, due to their level of knowledge.
AI has been integrated in various cancer fields including chemotherapy, radiotherapy and immunotherapy. The interaction between the drugs and the patients is the main focus of implementing AI in chemotherapy. The primary applications include chemotherapy drug use management, chemotherapy drug tolerance prediction, and chemotherapy programme optimisation [ 175 , 176 , 177 , 178 ]. AI can also be used in earlier detection of cancers. For example, the use of AI has enabled review and translation of mammograms faster with increased accuracy, reducing the need for unnecessary biopsies to detect breast cancers.
Additionally, the implementation of AI in radiotherapy assist radiologists to map out target areas or automatically plan radiation regimens for treatments as can be seen from the work from elsewhere [ 179 , 180 , 181 ]. Also, in the immunotherapy treatment, physicians uses AI to evaluate the treatment effect by adjusting the treatment plan for cancer patients [ 182 , 183 , 184 , 185 ].
AI can be used to add precision during surgery in determining cancer margins. Clear and adequate margins is a major determinant of completeness of surgery and cancer survival. AI and robotic surgery can help in accurate surgery which will preserve the function of organs without compromising on the oncological safety. Virtual reality simulation and AI can also help train future oncologists and surgeons. It is well recognised that AI can deliver critical information and insights that cannot be detected by human identification, thus personalising each cancer patient with suitable treatment [ 186 , 187 , 188 ]. Additionally, it is believed that AI can be a significant driver in human cancer research and treatment, by paving the way for the development of anticancer medication that could substantially accelerate the discover of new materials. It is also believed that AI will have a significant impact on the medical technologies in the upcoming years [ 174 ].
While AI has the limits of finding out things from the data which is used to train the AI system, the radical innovation from AI cannot be expected. This type of innovation in cancer research will require fundamental basic sciences to develop and propose new ambitious measures. The authors of this review paper allude to one such possibility wherein the use of a new type of alloy nanoparticles can play a vital role in cancer treatment. We bring in the concept of high entropy alloy nanoparticles (HEAs) [ 189 ] which is a material system or an alloy containing five or more type of chemical elements arranged in a crystalline structure. These nanoparticles are known for their chemical homogeneity and could be promising for different kind of functionalisation because all metallic elements can reside over the surface of nanoparticles (Fig. 14 ), while pure element metallic nanoparticles have just one single element formulating the surface. Recently, HEA nanoparticles have shown prominence in the catalytic activity due to multiple binding sites over the surface [ 190 , 191 ]. For example, the HEA nanoparticle surface atoms have different surroundings compared to their neighbour, which provide wide energy catalytic sites for analytes. As the paper alluded to the positive effects of nanoparticles (NPs), those benefits can best be realised by forcing a chemical reaction between an element contained in the NP and the cancer cell. However, not all cancer cell with initiate chemical reaction with an element. In that aspect, HEAs containing multi-elements offers higher probability for a reaction to initiate, and further accelerated by the presence of other surrounding (less chemically affinitive) particles (see Fig. 14 ). Additionally, the unique properties of NP obtained from their surface functionalisation can offer new abilities to study HEA nanoparticle interaction with biomatter for biosensing. HEAs gives wide space to manipulate or allow to bind with varying kinds of hetero-receptors. A major challenge with HEA nanoparticles is to fabricate free standing nanoparticles. In most cases, nanoparticle requires free standing (either powder form or suspended in solvent). Free standing nanoparticles are easy to be to be functionalised. Only few techniques such as carbothermal shocks method are available, where nanoparticles are supported over carbon nanofibre [ 192 ] and could not be separated from carbon nanofibre. Another method is cryomilling which is capable to prepare free standing nanoparticles in bulk quantity [ 193 ]. The best advantage of the HEA nanoparticles are the wide compositional space from the periodic table which means it is possible to mix different metallic elements to fabricate as many as 10 8 different types of nanoparticle in equi-atomic or quasi-equi-atomic proportions [ 194 ]. However, only a few metallic nanoparticles have been used at the pre-clinical stage and it has been quite challenging to get FDA approval for advancement in this area. Consequently, the idea being proposed here has just only remained a hypothesis. As such, it is our mere perspective at this stage, that HEA NPs can contribute significantly to cancer sensing/treatment and further research in this area will help to validate this hypothesis.
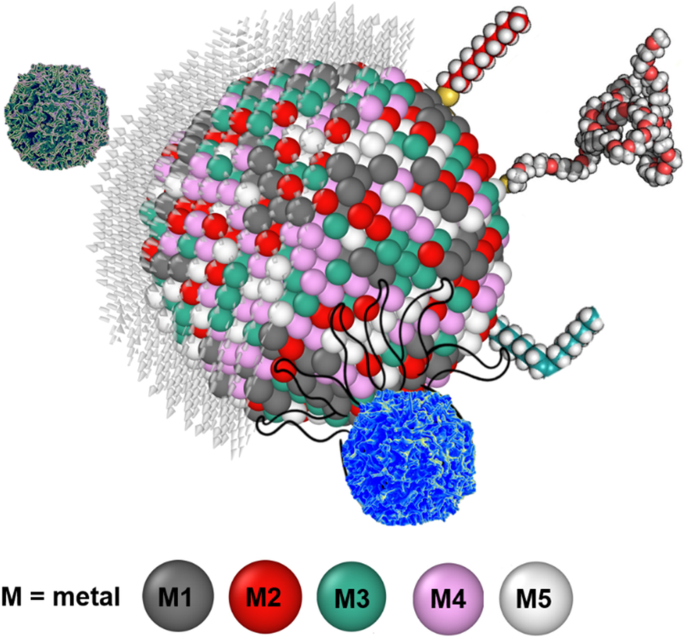
Interaction between HEA nanoparticle with a cancer cell. Distinctly different from simple metals, an HEA contain at least up to 5 chemical elements shown by M1, M2, M3, M4 and M5 and one out of these five elements is likely to be chemically affinitive to cancer cells making them vulnerable to succumb to HEA faster than single elemental NPs
6 Concluding remarks
The field of magneto-mechanical anti-cancer therapies has exponentially grown for the last 5 years, paving the foundation for new therapies in oncology. The main efficacy demonstration was done in vitro , using highly heterogeneous magnetic-responsive nanoparticles and magnetic stimulation. Beside the inaugural thermal effect, the demonstration that mechanical stimulation can modulate the cell biology of cancer is further comforted by the recent demonstration of mechano-transduction pathways. These pathways as well as the connected physico-mechanic properties of the tissues are probably as important for the physiological tissue homeostasis than the classical molecular pathways governing the initiation and promotion of cancer. Only a few in vivo investigations have been done, contrasting with the number of in vitro studies. In vivo strategies are confronted by the bottleneck of tissue delivery that is very poor after intravenous injection. Intratumor delivery is a growing alternative in nanomedicine that has potential of becoming the first strategy, increasing the efficacy of local nanoparticle delivery as well as it decreases the potential systemic side effects. Anticipating from the beginning of these investigations, the biocompatibility of nanoparticle is mandatory. Moreover, it will also be mandatory to decipher the physical properties of the targeted tissue, such as stiffness and intra-tissular pressure that could modulate the efficacy of magneto-mechanical therapy. Several imaging methodologies have been developed for that such as ultrasound stiffness imaging. A mechanical dosimetry mapping of the tissue should provide the opportunity of a real mechanical therapy personalisation. Both in vitro and in vivo studies in the field of magneto-mechanical therapies of cancer pave the way for a real renewing of cancer therapies, responding to the therapeutical resistances observed in the field of chemotherapy and targeted molecular and cellular therapies. Translating physics and nanomagnetism will need a strong interdisciplinarity associating synergistically physician, biologists and physicists.
Through this review, we tried to demonstrate that the transport of NPs within the tumour microvasculature can be greatly influenced by various parameters such as size, shape, surface functionality, etc. The microcirculation of NPs and their subsequent internalisation by disease cells can be understood through computer simulation methods such as IMFEM and DPD. The important roles played by above parameters can be elucidated through these simulations. Thus, by combing the IMFEM with DPD simulations, the life journey of the NP-based drug carriers can be predicted through multiscale modelling approach. Through these multiscale simulations, fundamental mechanisms underpinning the NP-mediated drug delivery can be elucidated. These detailed physical insights can provide useful guidelines in the design of NPs. For instance, larger sized NPs were found to be able to migrate into the ‘cell-free layer’ whereas smaller sized NPs could be more efficiently taken up by the diseased cells. Based on these observations, a multistage delivery platform was designed by Ferrari and co-worker [ 195 ]. In the design of this platform, biodegradable and biocompatible mesoporous silicon particles were used to carry nanosized quantum dots or carbon nanotubes. During the microcirculation process, these mesoporous silicon particles can be more easily accumulated at the tumour sites due to the EPR and margination effects. Then, the NPs were gradually released, and they diffuse into the tumour cells. Through receptor mediated endocytosis and other pathways, these NPs can be internalised by tumour cells. Comparing with traditional design of NPs, this multistage platform has considered different physical mechanisms during the NP-mediated drug delivery process. Note that the traditional design of NPs relies on the slow and inefficient ‘Edisonian’ approaches. Such a process is very time-consuming and cost inefficient. According to the multiscale modelling approach, the design of NPs can be more easily achieved through the computer simulations. Soon, we hope that simulation-based design paradigms can guide experimental design of next-generation NPs, with enhanced active targeting, low toxicity and limited side effects.
Sung, H., et al. (2021). Global cancer statistics 2020: GLOBOCAN estimates of incidence and mortality worldwide for 36 cancers in 185 countries. 71 (3), 209–249.
Castiglioni, A. (1947). A history of medicine . Book. https://doi.org/10.4324/9780429019883
Hanahan, D. (2022). Hallmarks of cancer: New dimensions. Cancer Discovery, 12 (1), 31–46.
Article CAS PubMed Google Scholar
Pedmedical. Healthy cell. 2022; Available from: https://www.shutterstock.com/discover/stock-assets-uk-0220?c3apidt=p11180842815&gclid=CjwKCAjwhNWZBhB_EiwAPzlhNkuEWQgNIi53MLZ7pPjOlHI2burmAnSGE3NyvROPpvHk2zrj9nG3cRoCxwUQAvD_BwE&gclsrc=aw.ds&kw=shutterstock .
Clinic, C. Carcinoma. 2019; Available from: https://my.clevelandclinic.org/health/diseases/23180-carcinoma#:~:text=Carcinoma%20is%20cancer%20that%20forms,head%20and%20neck%20are%20carcinomas .
Selchick, F. Cancer: Types, causes, prevention, and more. 2022; Available from: https://www.healthline.com/health/cancer#:~:text=Types%20of%20cancer&text=Carcinoma%20is%20a%20cancer%20that,cancers%20of%20the%20immune%20system .
Institute, N.C. What Is Cancer? 2021; Available from: https://www.cancer.gov/about-cancer/understanding/what-is-cancer#:~:text=Cancer%20is%20a%20disease%20caused,are%20also%20called%20genetic%20changes .
Cooper, J. L., & Robinson, P. (2000). The argument for making large classes seem small. New Directions for Teaching and Learning, 2000 (81), 5–16.
Article Google Scholar
Editorial. (2021). Advancing cancer therapy. Nature Cancer, 2 (3), 245–246. https://doi.org/10.1038/s43018-021-00192-x
Jain, R. K., & Stylianopoulos, T. (2010). Delivering nanomedicine to solid tumors. Nature Reviews Clinical Oncology, 7 (11), 653–664.
Article CAS PubMed PubMed Central Google Scholar
Hu, J.C.-M., & Zhang, L. (2009). Therapeutic nanoparticles to combat cancer drug resistance. Current Drug Metabolism, 10 (8), 836–841.
Peer, D., et al. (2007). Nanocarriers as an emerging platform for cancer therapy. Nature Nanotechnology, 2 (12), 751–760.
Naud, C., et al. (2020). Cancer treatment by magneto-mechanical effect of particles, a review. Nanoscale Advances, 2 (9), 3632–3655.
Murphy, C. J., et al. (2015). Biological responses to engineered nanomaterials: Needs for the next decade. ACS Central Science, 1 (3), 117–123.
Morton, J. (1997). Reviews. Thesis Eleven, 51 (1), 131–132.
Ghossain, A., & Ghossain, M. A. (2009). History of mastectomy before and after Halsted. Le Journal medical libanais. The Lebanese medical journal, 57 (2), 65–71.
PubMed Google Scholar
Sankaranarayanan, R., et al. (2013). Long term effect of visual screening on oral cancer incidence and mortality in a randomized trial in Kerala India. Oral Oncology, 49 (4), 314–321.
Article PubMed Google Scholar
Wang, K., & Tepper, J. E. (2021). Radiation therapy-associated toxicity: Etiology, management, and prevention. CA: A Cancer Journal for Clinicians, 71 (5), 437–454.
Bird, S. M. (2015). The 1959 meeting in Vienna on controlled clinical trials–A methodological landmark. Journal of the Royal Society of Medicine, 108 (9), 372–375.
Article PubMed PubMed Central Google Scholar
Cho, B. (2018). Intensity-modulated radiation therapy: A review with a physics perspective. Radiation Oncology Journal, 36 (1), 1–10.
Goodman, L. S., et al. (1946). Nitrogen mustard therapy: Use of methyl-bis (beta-chloroethyl) amine hydrochloride and tris (beta-chloroethyl) amine hydrochloride for hodgkin’s disease, lymphosarcoma, leukemia and certain allied and miscellaneous disorders. Journal of the American Medical Association, 132 (3), 126–132.
Farber, S., et al. (1948). Temporary remissions in acute leukemia in children produced by folic acid antagonist, 4-aminopteroyl-glutamic acid (aminopterin). New England Journal of Medicine, 238 (23), 787–793.
Hitchings, G. H., et al. (1950). Antagonists of nucleic acid derivatives: I The Lactobacillus casei model. Journal of Biological Chemistry, 183 (1), 1–9.
Article CAS Google Scholar
De Rooij, J. D., Zwaan, C. M., & van den Heuvel-Eibrink, M. (2015). Pediatric AML: From biology to clinical management. Journal of clinical medicine, 4 (1), 127–149.
Kim, H. (2020). Treatments for children and adolescents with AML. Blood research, 55 (S1), S5–S13.
Hermann, P. C., et al. (2007). Distinct populations of cancer stem cells determine tumor growth and metastatic activity in human pancreatic cancer. Cell Stem Cell, 1 (3), 313–323.
Slats, A., et al. (2005). Causes of death–other than progressive leukemia–in childhood acute lymphoblastic (ALL) and myeloid leukemia (AML): The Dutch Childhood Oncology Group experience. Leukemia, 19 (4), 537–544.
Bista, R., et al. (2017). Disulfiram overcomes bortezomib and cytarabine resistance in Down-syndrome-associated acute myeloid leukemia cells. Journal of Experimental & Clinical Cancer Research, 36 (1), 1–14.
Aleem, E., & Arceci, R. J. (2015). Targeting cell cycle regulators in hematologic malignancies. Frontiers in cell and developmental biology, 3 , 16.
Lee, Y. T., Tan, Y. J., & Oon, C. E. (2018). Molecular targeted therapy: Treating cancer with specificity. European journal of pharmacology, 834 , 188–196.
Yan, L., Rosen, N., & Arteaga, C. (2011). Targeted cancer therapies. Chinese journal of cancer, 30 (1), 1.
Mansour, M. A., Caputo, V. S., & Aleem, E. (2021). Highlights on selected growth factors and their receptors as promising anticancer drug targets. The International Journal of Biochemistry & Cell Biology, 140 , 106087.
Nadukkandy, A. S., et al. (2022). Tracing new landscapes in the arena of nanoparticle-based cancer immunotherapy. Frontiers in Nanotechnology, 4 , 911063.
Pankhurst, Q. A., et al. (2003). Applications of magnetic nanoparticles in biomedicine. Journal of physics D: Applied physics, 36 (13), R167.
Cheng, Y., et al. (2014). Multifunctional nanoparticles for brain tumor imaging and therapy. Advanced drug delivery reviews, 66 , 42–57.
Stephen, Z. R., Kievit, F. M., & Zhang, M. (2011). Magnetite nanoparticles for medical MR imaging. Materials Today, 14 (7–8), 330–338.
Jin, R., et al. (2014). Superparamagnetic iron oxide nanoparticles for MR imaging and therapy: Design considerations and clinical applications. Current opinion in pharmacology, 18 , 18–27.
Arruebo, M., et al. (2007). Magnetic nanoparticles for drug delivery. Nano Today, 2 (3), 22–32.
Mody, V. V., et al. (2014). Magnetic nanoparticle drug delivery systems for targeting tumor. Applied Nanoscience, 4 (4), 385–392.
Sun, C., Lee, J. S., & Zhang, M. (2008). Magnetic nanoparticles in MR imaging and drug delivery. Advanced drug delivery reviews, 60 (11), 1252–1265.
Gobbo, O. L., et al. (2015). Magnetic nanoparticles in cancer theranostics. Theranostics, 5 (11), 1249.
Gao, Y., et al. (2015). Emerging translational research on magnetic nanoparticles for regenerative medicine. Chemical Society Reviews, 44 (17), 6306–6329.
Zhang, Q., et al. (2017). Large-scale immuno-magnetic cell sorting of T cells based on a self-designed high-throughput system for potential clinical application. Nanoscale, 9 (36), 13592–13599.
Cartmell, S., et al. (2005). Use of magnetic particles to apply mechanical forces for bone tissue engineering purposes. in Journal of Physics: Conference Series. IOP Publishing.
Sanchez, C., et al. (2014). Targeting a G-protein-coupled receptor overexpressed in endocrine tumors by magnetic nanoparticles to induce cell death. ACS Nano, 8 (2), 1350–1363.
Kafrouni, L., & Savadogo, O. (2016). Recent progress on magnetic nanoparticles for magnetic hyperthermia. Progress in Biomaterials, 5 (3), 147–160.
Dutz, S., & Hergt, R. (2014). Magnetic particle hyperthermia—A promising tumour therapy? Nanotechnology, 25 (45), 452001.
Corchero, J. L., & Villaverde, A. (2009). Biomedical applications of distally controlled magnetic nanoparticles. Trends in biotechnology, 27 (8), 468–476.
Vitol, E. A., Novosad, V., & Rozhkova, E. A. (2012). Microfabricated magnetic structures for future medicine: From sensors to cell actuators. Nanomedicine, 7 (10), 1611–1624.
Goiriena-Goikoetxea, M., et al. (2020). Disk-shaped magnetic particles for cancer therapy. Applied Physics Reviews, 7 (1), 011306.
Mansell, R., et al. (2017). Magnetic particles with perpendicular anisotropy for mechanical cancer cell destruction. Scientific reports, 7 (1), 1–7.
Sanità, G., Carrese, B., & Lamberti, A. (2020). Nanoparticle surface functionalization: How to improve biocompatibility and cellular internalization. Frontiers in Molecular Biosciences, 7 , 587012.
Rea, I., et al. (1840). 2014 Diatomite biosilica nanocarriers for siRNA transport inside cancer cells. Biochimica et Biophysica Acta (BBA)-General Subjects, 12 , 3393–3403.
Google Scholar
Banihashem, S., Nezhati, M. N., & Panahia, H. A. (2020). Synthesis of chitosan-grafted-poly (N-vinylcaprolactam) coated on the thiolated gold nanoparticles surface for controlled release of cisplatin. Carbohydrate polymers, 227 , 115333.
Korpany, K. V., et al. (2016). One-step ligand exchange and switching from hydrophobic to water-stable hydrophilic superparamagnetic iron oxide nanoparticles by mechanochemical milling. Chemical Communications, 52 (14), 3054–3057.
Yue, L., et al. (2019). Gold nanorods with a noncovalently tailorable surface for multi-modality image-guided chemo-photothermal cancer therapy. Chemical Communications, 55 (90), 13506–13509.
Sakaguchi, M., et al. (2019). Surface modification of Fe3O4 nanoparticles with dextran via a coupling reaction between naked Fe3O4 mechano-cation and naked dextran mechano-anion: A new mechanism of covalent bond formation. Advanced Powder Technology, 30 (4), 795–806.
Świętek, M., et al. (2019). Scavenging of reactive oxygen species by phenolic compound-modified maghemite nanoparticles. Beilstein journal of nanotechnology, 10 (1), 1073–1088.
Zhao, C.-Y., et al. (2018). Nanotechnology for cancer therapy based on chemotherapy. Molecules, 23 (4), 826.
Katragadda, U., et al. (2013). Combined delivery of paclitaxel and tanespimycin via micellar nanocarriers: Pharmacokinetics, efficacy and metabolomic analysis. PLoS One, 8 (3), e58619.
Liu, K., et al. (2017). Paclitaxel and quercetin nanoparticles co-loaded in microspheres to prolong retention time for pulmonary drug delivery. International journal of nanomedicine, 12 , 8239.
Araujo, J., & Logothetis, C. (2010). Dasatinib: A potent SRC inhibitor in clinical development for the treatment of solid tumors. Cancer treatment reviews, 36 (6), 492–500.
Yang, L., et al. (2021). Carrier-free prodrug nanoparticles based on dasatinib and cisplatin for efficient antitumor in vivo. Asian journal of pharmaceutical sciences, 16 (6), 762–771.
Siddique, S., & Chow, J. C. (2022). Recent advances in functionalized nanoparticles in cancer theranostics. Nanomaterials, 12 (16), 2826.
Li, Y., et al. (2016). Cell and nanoparticle transport in tumour microvasculature: The role of size, shape and surface functionality of nanoparticles. Interface focus, 6 (1), 20150086.
Stillman, N. R., et al. (2020). In silico modelling of cancer nanomedicine, across scales and transport barriers. NPJ Computational Materials, 6 (1), 1–10.
Chauviere, A. H., et al. (2010). Mathematical oncology: How are the mathematical and physical sciences contributing to the war on breast cancer? Current breast cancer reports, 2 (3), 121–129.
Deisboeck, T. S., et al. (2009). In silico cancer modeling: Is it ready for prime time? Nature Clinical Practice Oncology, 6 (1), 34–42.
Rejniak, K. A., & Anderson, A. R. (2011). Hybrid models of tumor growth. Wiley Interdisciplinary Reviews: Systems Biology and Medicine, 3 (1), 115–125.
CAS PubMed Google Scholar
An, G., et al. (2009). Agent-based models in translational systems biology. Wiley Interdisciplinary Reviews: Systems Biology and Medicine, 1 (2), 159–171.
Konukoglu, E., et al. (2010). Mathematical and computational biology. In: T. S. Deisboeck, G. Stamatakos (Eds.), Multiscale cancer modeling . CRC Press.
Norton, K.-A., et al. (2019). Multiscale agent-based and hybrid modeling of the tumor immune microenvironment. Processes, 7 (1), 37.
Rejniak, K. A., & Anderson, A. R. A. (2011). Hybrid models of tumor growth. Wire Mechanism of Diseae, 3 (1), 115–125. https://doi.org/10.1002/wsbm.102
Basanta, D., et al. (2009). The role of transforming growth factor-β–mediated tumor-stroma interactions in prostate cancer progression: An integrative approach. Cancer research, 69 (17), 7111–7120.
Enderling, H., Hlatky, L., & Hahnfeldt, P. (2009). Migration rules: Tumours are conglomerates of self-metastases. British journal of cancer, 100 (12), 1917–1925.
Bankhead, A., III., Magnuson, N. S., & Heckendorn, R. B. (2007). Cellular automaton simulation examining progenitor hierarchy structure effects on mammary ductal carcinoma in situ. Journal of theoretical biology, 246 (3), 491–498.
Engelberg, J. A., Ropella, G. E., & Hunt, C. A. (2008). Essential operating principles for tumor spheroid growth. BMC systems biology, 2 (1), 1–19.
Shirinifard, A., et al. (2009). 3D multi-cell simulation of tumor growth and angiogenesis. PLoS One, 4 (10), e7190.
Rubenstein, B. M., & Kaufman, L. J. (2008). The role of extracellular matrix in glioma invasion: A cellular Potts model approach. Biophysical journal, 95 (12), 5661–5680.
Van Leeuwen, I. M., et al. (2009). An integrative computational model for intestinal tissue renewal. Cell proliferation, 42 (5), 617–636.
Toma, A., et al. (2012). A novel method for simulating the extracellular matrix in models of tumour growth. Computational and Mathematical Methods in Medicine, 2012 , 1–11. https://doi.org/10.1155/2012/109019
Wang, Z., et al. (2015). Simulating cancer growth with multiscale agent-based modeling. in Seminars in cancer biology. Elsevier.
Lapin, A., et al. (2022). Integrating a dynamic central metabolism model of cancer cells with a hybrid 3D multiscale model for vascular hepatocellular carcinoma growth. Scientific Reports, 12 (1), 1–13.
Zhu, X., et al. (2011). Cancer stem cell, niche and EGFR decide tumor development and treatment response: A bio-computational simulation study. Journal of theoretical biology, 269 (1), 138–149.
Sefidgar, M., et al. (2015). Numerical modeling of drug delivery in a dynamic solid tumor microvasculature. Microvascular research, 99 , 43–56.
Shah, P. N., et al. (2018). Extravasation of Brownian spheroidal nanoparticles through vascular pores. Biophysical journal, 115 (6), 1103–1115.
Nehoff, H., et al. (2014). Nanomedicine for drug targeting: Strategies beyond the enhanced permeability and retention effect. International journal of nanomedicine, 9 (1), 2539–2555.
PubMed PubMed Central Google Scholar
Hamis, S., Nithiarasu, P., & Powathil, G. G. (2018). What does not kill a tumour may make it stronger: In silico insights into chemotherapeutic drug resistance. Journal of theoretical biology, 454 , 253–267.
Angioletti-Uberti, S. (2017). Theory, simulations and the design of functionalized nanoparticles for biomedical applications: A soft matter perspective. npj Computational Materials, 3 (1), 1–15.
Finley, S. D., et al. (2015). Pharmacokinetics of anti-VEGF agent aflibercept in cancer predicted by data-driven molecular-detailed model. CPT: Pharmacometrics & systems pharmacology, 4 (11), 641–649.
CAS Google Scholar
Longmire, M., Choyke, P. L., & Kobayashi, H. (2008). Clearance properties of nano-sized particles and molecules as imaging agents: considerations and caveats. Nanomedicines, 3 (5), 703–717.
Gustafson, H. H., et al. (2015). Nanoparticle uptake: The phagocyte problem. Nano Today, 10 (4), 487–510.
Fedosov, D. A., Noguchi, H., & Gompper, G. (2014). Multiscale modeling of blood flow: From single cells to blood rheology. Biomechanics and modeling in mechanobiology, 13 (2), 239–258.
Lopez, H., & Lobaskin, V. (2015). Coarse-grained model of adsorption of blood plasma proteins onto nanoparticles. The Journal of chemical physics, 143 (24), 12B620_1.
Shao, Q., & Hall, C. K. (2016). Protein adsorption on nanoparticles: Model development using computer simulation. Journal of Physics: Condensed Matter, 28 (41), 414019.
Maleki, R., et al. (2020). pH-sensitive loading/releasing of doxorubicin using single-walled carbon nanotube and multi-walled carbon nanotube: A molecular dynamics study. Computer methods and programs in biomedicine, 186 , 105210.
Müller, K., Fedosov, D. A., & Gompper, G. (2014). Margination of micro-and nano-particles in blood flow and its effect on drug delivery. Scientific reports, 4 (1), 1–8.
Wu, M., et al. (2014). The effect of interstitial pressure on therapeutic agent transport: Coupling with the tumor blood and lymphatic vascular systems. Journal of theoretical biology, 355 , 194–207.
Wijeratne, P. A., & Vavourakis, V. (2019). A quantitative in silico platform for simulating cytotoxic and nanoparticle drug delivery to solid tumours. Interface Focus, 9 (3), 20180063.
Zhang, X., Ma, G., & Wei, W. (2021). Simulation of nanoparticles interacting with a cell membrane: Probing the structural basis and potential biomedical application. NPG Asia Materials, 13 (1), 1–18.
Ling, D., & Hyeon, T. (2013). Chemical design of biocompatible iron oxide nanoparticles for medical applications. Small (Weinheim an der Bergstrasse, Germany), 9 (9–10), 1450–1466.
Samadishadlou, M., et al. (2018). Magnetic carbon nanotubes: Preparation, physical properties, and applications in biomedicine. Artificial cells, nanomedicine, and biotechnology, 46 (7), 1314–1330.
Bodhinayake, I., Ottenhausen, M., & Boockvar, J. A. (2014). Targeting a heterogeneous tumor: The promise of the interleukin-13 receptor α2. Neurosurgery, 75 (2), N18–N19.
Hadjipanayis, C. G., et al. (2010). EGFRvIII antibody–conjugated iron oxide nanoparticles for magnetic resonance imaging–guided convection-enhanced delivery and targeted therapy of glioblastomaEGFRvIII-targeted therapy of GBM by IONPs after CED. Cancer research, 70 (15), 6303–6312.
Prats-Alfonso, E., & Albericio, F. (2011). Functionalization of gold surfaces: Recent developments and applications. Journal of materials science, 46 (24), 7643–7648.
Verma, J., & Bhattacharya, A. (2018). Development of coating formulation with silica–titania core–shell nanoparticles against pathogenic fungus. Royal Society Open Science, 5 (8), 180633.
Verma, J., et al. (2020). Super protective anti-bacterial coating development with silica–titania nano core–shells. Nanoscale Advances, 2 (9), 4093–4105.
Tietze, R., et al. (2015). Magnetic nanoparticle-based drug delivery for cancer therapy. Biochemical and biophysical research communications, 468 (3), 463–470.
Golovin, Y. I., et al. (2015). Towards nanomedicines of the future: Remote magneto-mechanical actuation of nanomedicines by alternating magnetic fields. Journal of Controlled Release, 219 , 43–60.
Mannix, R. J., et al. (2008). Nanomagnetic actuation of receptor-mediated signal transduction. Nature nanotechnology, 3 (1), 36–40.
Tay, A., et al. (2016). Induction of calcium influx in cortical neural networks by nanomagnetic forces. ACS Nano, 10 (2), 2331–2341.
Kilinc, D., Dennis, C. L., & Lee, G. U. (2016). Bio-nano-magnetic materials for localized mechanochemical stimulation of cell growth and death. Advanced Materials, 28 (27), 5672–5680.
Forbes, Z. G., et al. (2008). Validation of high gradient magnetic field based drug delivery to magnetizable implants under flow. IEEE Transactions on Biomedical Engineering, 55 (2), 643–649.
Mizuki, T., et al. (2010). Activity of an enzyme immobilized on superparamagnetic particles in a rotational magnetic field. Biochemical and biophysical research communications, 393 (4), 779–782.
Lopez, S., et al. (2022). Magneto-mechanical destruction of cancer-associated fibroblasts using ultra-small iron oxide nanoparticles and low frequency rotating magnetic fields. Nanoscale Advances, 4 (2), 421–436.
Thébault, C., et al. (2021). Magneto-mechanical treatment of human glioblastoma cells with engineered iron oxide powder microparticles for triggering apoptosis. Nanoscale Advances, 3 (21), 6213–6222.
Kim, D.-H., et al. (2010). Biofunctionalized magnetic-vortex microdiscs for targeted cancer-cell destruction. Nature materials, 9 (2), 165–171.
Ade, H., & Stoll, H. (2009). Near-edge X-ray absorption fine-structure microscopy of organic and magnetic materials. Nature materials, 8 (4), 281–290.
Wo, F., et al. (2016). A multimodal system with synergistic effects of magneto-mechanical, photothermal, photodynamic and chemo therapies of cancer in graphene-quantum dot-coated hollow magnetic nanospheres. Theranostics, 6 (4), 485.
Tian, Q., et al. (2013). Sub-10 nm Fe3O4@ Cu2–x S core–shell nanoparticles for dual-modal imaging and photothermal therapy. Journal of the American Chemical Society, 135 (23), 8571–8577.
Wang, X., et al. (2014). Multifunctional graphene quantum dots for simultaneous targeted cellular imaging and drug delivery. Colloids and Surfaces B: Biointerfaces, 122 , 638–644.
Cheng, Z., et al. (2021). Nanomaterials for cancer therapy: Current progress and perspectives. Journal of Hematology & Oncology, 14 (1), 1–27.
Masood, F. (2016). Polymeric nanoparticles for targeted drug delivery system for cancer therapy. Materials Science and Engineering: C, 60 , 569–578.
Vijayan, V., et al. (2013). Optimization and charaterization of repaglinide biodegradable polymeric nanoparticle loaded transdermal patchs: In vitro and in vivo studies. Colloids and Surfaces B: Biointerfaces, 111 , 150–155.
Shastri, V. P. (2003). Non-degradable biocompatible polymers in medicine: Past, present and future. Current pharmaceutical biotechnology, 4 (5), 331–337.
Martín-Saldaña, S., et al. (2017). Polymeric nanoparticles loaded with dexamethasone or α-tocopheryl succinate to prevent cisplatin-induced ototoxicity. Acta biomaterialia, 53 , 199–210.
Bao, S., et al. (2021). Dual targeting EGFR and STAT3 with erlotinib and alantolactone co-loaded PLGA nanoparticles for pancreatic cancer treatment. Frontiers in pharmacology, 12 , 625084.
Le Broc-Ryckewaert, D., et al. (2013). Development of innovative paclitaxel-loaded small PLGA nanoparticles: Study of their antiproliferative activity and their molecular interactions on prostatic cancer cells. International journal of pharmaceutics, 454 (2), 712–719.
Zhang, X., et al. (2019). Trastuzumab-coated nanoparticles loaded with docetaxel for breast cancer therapy. Dose-Response, 17 (3), 1559325819872583.
Liu, Y., et al. (2019). One-dimensional Fe2P acts as a Fenton agent in response to NIR II light and ultrasound for deep tumor synergetic theranostics. Angewandte Chemie, 131 (8), 2429–2434.
Danhier, F., Feron, O., & Préat, V. (2010). To exploit the tumor microenvironment: Passive and active tumor targeting of nanocarriers for anti-cancer drug delivery. Journal of controlled release, 148 (2), 135–146.
Choi, J.-S., & Park, J.-S. (2017). Development of docetaxel nanocrystals surface modified with transferrin for tumor targeting. Drug design, development and therapy, 11 , 17.
Zhou, Y., et al. (2007). Impact of single-chain Fv antibody fragment affinity on nanoparticle targeting of epidermal growth factor receptor-expressing tumor cells. Journal of molecular biology, 371 (4), 934–947.
Bhattacharya, R., et al. (2007). Attaching folic acid on gold nanoparticles using noncovalent interaction via different polyethylene glycol backbones and targeting of cancer cells. Nanomedicine: Nanotechnology, Biology and Medicine, 3 (3), 224–238.
Raj, S., et al. (2021). Specific targeting cancer cells with nanoparticles and drug delivery in cancer therapy. Seminars in Cancer Biology, 69 , 66–177.
Chen, F., et al. (2015). New horizons in tumor microenvironment biology: Challenges and opportunities. BMC medicine, 13 (1), 1–14.
Belli, C., et al. (2018). Targeting the microenvironment in solid tumors. Cancer treatment reviews, 65 , 22–32.
Hu, X., et al. (2017). Nanotechnology based therapeutic modality to boost anti-tumor immunity and collapse tumor defense. Journal of Controlled Release, 256 , 26–45.
Izci, M., et al. (2021). The use of alternative strategies for enhanced nanoparticle delivery to solid tumors. Chemical reviews, 121 (3), 1746–1803.
Wu, T., & Dai, Y. (2017). Tumor microenvironment and therapeutic response. Cancer letters, 387 , 61–68.
Yang, M., et al. (2021). The application of nanoparticles in cancer immunotherapy: Targeting tumor microenvironment. Bioactive materials, 6 (7), 1973–1987.
Ganesh, K., & Massagué, J. (2021). Targeting metastatic cancer. Nature medicine, 27 (1), 34–44.
Fernandes, C., Suares, D., & Yergeri, M. C. (2018). Tumor microenvironment targeted nanotherapy. Frontiers in pharmacology, 9 , 1230.
Palucka, K., & Banchereau, J. (2013). Dendritic-cell-based therapeutic cancer vaccines. Immunity, 39 (1), 38–48.
Melero, I., et al. (2014). Therapeutic vaccines for cancer: An overview of clinical trials. Nature reviews Clinical oncology, 11 (9), 509–524.
Zhang, D. K., Cheung, A. S., & Mooney, D. J. (2020). Activation and expansion of human T cells using artificial antigen-presenting cell scaffolds. Nature Protocols, 15 (3), 773–798.
Otsuka, R., et al. (2020). Efficient generation of thymic epithelium from induced pluripotent stem cells that prolongs allograft survival. Scientific reports, 10 (1), 1–8.
Zhang, Q., et al. (2017). Biomimetic magnetosomes as versatile artificial antigen-presenting cells to potentiate T-cell-based anticancer therapy. ACS Nano, 11 (11), 10724–10732.
Cheng, K., et al. (2018). Sequentially responsive therapeutic peptide assembling nanoparticles for dual-targeted cancer immunotherapy. Nano letters, 18 (5), 3250–3258.
Feng, X., et al. (2019). Immunomodulatory nanosystems. Advanced science, 6 (17), 1900101.
Liu, Z., et al. (2018). Immunomodulating nanomedicine for cancer therapy. Nano letters, 18 (11), 6655–6659.
Li, K., et al. (2022). Targeting innate immune system by nanoparticles for cancer immunotherapy. Journal of Materials Chemistry B, 10 , 1709–1733.
Gao, S., et al. (2021). Nanotechnology for boosting cancer immunotherapy and remodeling tumor microenvironment: The horizons in cancer treatment. ACS Nano, 15 (8), 12567–12603.
Siegel, R. L., et al. (2021). Cancer statistics, 2021. CA: A Cancer Journal for Clinicians, 71 (1), 7–33.
Vasan, N., Baselga, J., & Hyman, D. M. (2019). A view on drug resistance in cancer. Nature, 575 (7782), 299–309.
Hussein, N. A., et al. (2021). The role of endolysosomal trafficking in anticancer drug resistance. Drug Resistance Updates, 57 , 100769.
Levin, M., et al. (2021). Deciphering molecular mechanisms underlying chemoresistance in relapsed AML patients: Towards precision medicine overcoming drug resistance. Cancer cell international, 21 (1), 1–16.
Bukhari, S. N. A. (2022). Emerging nanotherapeutic approaches to overcome drug resistance in cancers with update on clinical trials. Pharmaceutics, 14 (4), 866.
Haider, T., et al. (2020). Drug resistance in cancer: Mechanisms and tackling strategies. Pharmacological Reports, 72 (5), 1125–1151.
Mao, Y., et al. (2021). Insight of nanomedicine strategies for a targeted delivery of nanotherapeutic cues to cope with the resistant types of cancer stem cells. Journal of Drug Delivery Science and Technology, 64 , 102681.
de Oliveira, B. S. A., et al. (2021). Nanotherapeutic approach to tackle chemotherapeutic resistance of cancer stem cells. Life Sciences, 279 , 119667.
Mitamura, T., et al. (2018). Induction of anti-VEGF therapy resistance by upregulated expression of microseminoprotein (MSMP). Oncogene, 37 (6), 722–731.
Kim, S.-J., et al. (2006). Targeting platelet-derived growth factor receptor on endothelial cells of multidrug-resistant prostate cancer. Journal of the National Cancer Institute, 98 (11), 783–793.
Zhao, B. X., et al. (2015). Establishment and biological characteristics of acquired gefitinib resistance in cell line NCI-H1975/gefinitib-resistant with epidermal growth factor receptor T790M mutation. Molecular medicine reports, 11 (4), 2767–2774.
Meijer, C., et al. (1992). Relationship of cellular glutathione to the cytotoxicity and resistance of seven platinum compounds. Cancer research, 52 (24), 6885–6889.
Liang, G., et al. (2020). Engineered exosomes for targeted co-delivery of miR-21 inhibitor and chemotherapeutics to reverse drug resistance in colon cancer. Journal of nanobiotechnology, 18 (1), 1–15.
Wong-Brown, M., van der Westhuizen, A., & Bowden, N. (2020). Targeting DNA repair in ovarian cancer treatment resistance. Clinical Oncology, 32 (8), 518–526.
Sun, W., et al. (2015). Autophagy facilitates multidrug resistance development through inhibition of apoptosis in breast cancer cells. Neoplasma, 62 (2), 199–208.
Teeuwssen, M., & Fodde, R. (2019). Wnt signaling in ovarian cancer stemness, EMT, and therapy resistance. Journal of clinical medicine, 8 (10), 1658.
Wang, L., et al. (2018). EMT-and stroma-related gene expression and resistance to PD-1 blockade in urothelial cancer. Nature communications, 9 (1), 1–12.
Grisoni, F., et al. (2019). De novo design of anticancer peptides by ensemble artificial neural networks. Journal of Molecular Modeling, 25 (5), 1–10.
Sherin, L., Sohail, A., & Shujaat, S. (2019). Time-dependent AI-Modeling of the anticancer efficacy of synthesized gallic acid analogues. Computational biology and chemistry, 79 , 137–146.
Grisoni, F., et al. (2018). Designing anticancer peptides by constructive machine learning. ChemMedChem, 13 (13), 1300–1302.
Liang, G., et al. (2020). The emerging roles of artificial intelligence in cancer drug development and precision therapy. Biomedicine & Pharmacotherapy, 128 , 110255.
Chen, G., et al. (2018). Predict effective drug combination by deep belief network and ontology fingerprints. Journal of biomedical informatics, 85 , 149–154.
Levine, M. N., et al. (2019). Learning health system for breast cancer: Pilot project experience. JCO Clinical Cancer Informatics, 3 , 1–11.
Smaïl-Tabbone, M., & Rance, B. (2020). Contributions from the 2019 literature on bioinformatics and translational informatics. Yearbook of Medical Informatics, 29 (01), 188–192.
Zhu, X., et al. (2012). An intronic polymorphism in GRP78 improves chemotherapeutic prediction in non-small cell lung cancer. Chest, 141 (6), 1466–1472.
Fiorino, C., et al. (2020). Technology-driven research for radiotherapy innovation. Molecular oncology, 14 (7), 1500–1513.
Lou, B., et al. (2019). An image-based deep learning framework for individualising radiotherapy dose: A retrospective analysis of outcome prediction. The Lancet Digital Health, 1 (3), e136–e147.
Meyer, P., et al. (2018). Survey on deep learning for radiotherapy. Computers in biology and medicine, 98 , 126–146.
Jabbari, P., & Rezaei, N. (2019). Artificial intelligence and immunotherapy. Expert Review of Clinical Immunology, 15 (7), 689–691.
Trebeschi, S., et al. (2019). Predicting response to cancer immunotherapy using noninvasive radiomic biomarkers. Annals of Oncology, 30 (6), 998–1004.
Abbasi, J. (2019). “Electronic nose” predicts immunotherapy response. JAMA, 322 (18), 1756–1756.
Tan, S., Li, D., & Zhu, X. (2020). Cancer immunotherapy: Pros, cons and beyond. Biomedicine & Pharmacotherapy, 124 , 109821.
Tran, W. T., et al. (2019). Personalized breast cancer treatments using artificial intelligence in radiomics and pathomics. Journal of Medical Imaging and Radiation Sciences, 50 (4), S32–S41.
Lamy, J.-B., et al. (2019). Explainable artificial intelligence for breast cancer: A visual case-based reasoning approach. Artificial Intelligence in Medicine, 94 , 42–53.
Somashekhar, S., et al. (2018). Watson for Oncology and breast cancer treatment recommendations: agreement with an expert multidisciplinary tumor board. Annals of Oncology, 29 (2), 418–423.
Katiyar, N. K., Goel, G., & Goel, S. (2021). Emergence of machine learning in the development of high entropy alloy and their prospects in advanced engineering applications. Emergent Materials, 4 (6), 1635–1648.
Katiyar, N. K., et al. (2021). A perspective on the catalysis using the high entropy alloys. Nano Energy, 88 , 106261.
Katiyar, N. K., et al. (2021). Electrooxidation of hydrazine utilizing high-entropy alloys: Assisting the oxygen evolution reaction at the thermodynamic voltage. ACS Catalysis, 11 (22), 14000–14007.
Yao, Y., et al. (2018). Carbothermal shock synthesis of high-entropy-alloy nanoparticles. Science, 359 (6383), 1489–1494.
Katiyar, N. K., Biswas, K., & Tiwary, C. (2021). Cryomilling as environmentally friendly synthesis route to prepare nanomaterials. International Materials Reviews, 66 (7), 493–532.
Cantor, B. (2021). Multicomponent high-entropy Cantor alloys. Progress in Materials Science, 120 , 100754.
Tasciotti, E., et al. (2008). Mesoporous silicon particles as a multistage delivery system for imaging and therapeutic applications. Nature nanotechnology, 3 (3), 151–157.
Download references
SG acknowledge the financial support provided by the UKRI via Grant Nos. EP/S036180/1, EP/T001100/1 and EP/T024607/1, feasibility study awards to LSBU from the UKRI National Interdisciplinary Circular Economy Hub (EP/V029746/1) and Transforming the Foundation Industries: a Network + (EP/V026402/1), the Hubert Curien Partnership award 2022 from the British Council, Transforming the Partnership award from the Royal Academy of Engineering (TSP1332) and the Newton Fellowship award from the Royal Society (NIF\R1\191571).
Author information
Authors and affiliations.
School of Engineering, London South Bank University, London, SE10AA, UK
Jaya Verma, Caaisha Warsame, Nirmal Kumar Katiyar & Saurav Goel
Surgical Oncology, PSGIMSR, Coimbatore, India
Rajkumar Kottayasamy Seenivasagam
School of Applied Sciences, Division of Human Sciences, Cancer Biology and Therapy Research Group, London South Bank University, London, SE10AA, UK
Eiman Aleem
Department of Mechanical Engineering, University of Petroleum and Energy Studies, Dehradun, 248007, India
Saurav Goel
You can also search for this author in PubMed Google Scholar
Corresponding author
Correspondence to Saurav Goel .
Ethics declarations
Conflict of interest.
The authors declare no competing interests.
Additional information
Publisher's note.
Springer Nature remains neutral with regard to jurisdictional claims in published maps and institutional affiliations.
Rights and permissions
Open Access This article is licensed under a Creative Commons Attribution 4.0 International License, which permits use, sharing, adaptation, distribution and reproduction in any medium or format, as long as you give appropriate credit to the original author(s) and the source, provide a link to the Creative Commons licence, and indicate if changes were made. The images or other third party material in this article are included in the article's Creative Commons licence, unless indicated otherwise in a credit line to the material. If material is not included in the article's Creative Commons licence and your intended use is not permitted by statutory regulation or exceeds the permitted use, you will need to obtain permission directly from the copyright holder. To view a copy of this licence, visit http://creativecommons.org/licenses/by/4.0/ .
Reprints and permissions
About this article
Verma, J., Warsame, C., Seenivasagam, R.K. et al. Nanoparticle-mediated cancer cell therapy: basic science to clinical applications. Cancer Metastasis Rev 42 , 601–627 (2023). https://doi.org/10.1007/s10555-023-10086-2
Download citation
Received : 03 October 2022
Accepted : 16 January 2023
Published : 24 February 2023
Issue Date : September 2023
DOI : https://doi.org/10.1007/s10555-023-10086-2
Share this article
Anyone you share the following link with will be able to read this content:
Sorry, a shareable link is not currently available for this article.
Provided by the Springer Nature SharedIt content-sharing initiative
- Nanoparticles
- MD simulations
- Find a journal
- Publish with us
- Track your research

- < Previous
Home > Theses and Dissertations > Theses > All Theses > 2172
Comprehensive Cancer Care: an outpatient treatment facility
Braden Abrams Reid , Clemson University
Date of Award
Document type, degree name.
Master of Architecture (MArch)
Legacy Department
Architecture
Committee Chair/Advisor
David Allison
Committee Member
Daniel Harding
John Jacques
Dr. Cynthia Pury
A cancer diagnosis can result in a loss of decision-making and control for patients. The majority of clinical treatments fail to adequately address corollary symptoms and needs that arise both from treatment and from the disease itself. Thus, the architectural problem identified in this thesis inquiry is how can an outpatient cancer centre treat the whole patient comprehensively, while at the same time instilling control and re-empowering the patient? This thesis project proposes a patient-centered environment designed to support the myriad and nuanced needs of the cancer patient, as well as a site-specific proposal for a comprehensive outpatient cancer treatment centre located in Spartanburg County, South Carolina. This cancer centre is designed with the notion of patient-empowerment as a design driver, and incorporates non-traditional complementary treatments in addition to those clinical treatments typically offered in the conventional North American cancer centre.
By way of supporting this topical area of inquiry, the following topics comprise the scope of inquiry undertaken in this project: Clinical Cancer Treatment Architecture: the three main facility types in which cancer treatment occurs - freestanding, aggregated, and monolithic. Complementary/Alternative Treatment Architecture: both cancer-focused and general alternative treatment centres were also investigated, in order to determine what complementary therapies might be appropriate to incorporate with a clinical cancer treatment center. Additionally, these facilities were helpful in giving cues as to what physical design drivers could help guide the subsequent thesis design proposal.
The review of many personal cancer patient blogs and video diaries (all openly available online) was important in framing the experience of the patient. What therapies were commonly feared, personal aversions that developed as a result of treatment, and similar notions written or spoken about all helped to give a sense of what it might be like to be in a patient's situation, and what design decisions might have been helpful in nurturing a more positive experience.
Design principles were developed out of the topical inquiry and architectural investigation, with the focus of patient empowerment at hand. Similarly, site selection, programmatic development, and the final design proposal were each conducted through the lens of the patient experience, and how best to instill control before, during, and after treatment is delivered (that is to say, before the patient arrives, the experience of receiving care, and what is taken away once they leave the treatment centre).
The resulting design proposal developed from this investigation is a 45,000 square foot outpatient cancer treatment centre located in Spartanburg County, South Carolina. The facility comprises a full hematology and infusion therapy suite, a radiation therapy suite, an alternative medicine suite, a physical therapy program, mind-body therapies, and a diet and nutrition area. Patients originating in the Cities of Greenville, Spartanburg, and Greer, and all surrounding areas will utilize this centre.
Recommended Citation
Reid, Braden Abrams, "Comprehensive Cancer Care: an outpatient treatment facility" (2014). All Theses . 2172. https://tigerprints.clemson.edu/all_theses/2172
Since June 16, 2015
Included in
Architecture Commons , Medicine and Health Sciences Commons
To view the content in your browser, please download Adobe Reader or, alternately, you may Download the file to your hard drive.
NOTE: The latest versions of Adobe Reader do not support viewing PDF files within Firefox on Mac OS and if you are using a modern (Intel) Mac, there is no official plugin for viewing PDF files within the browser window.
Advanced Search
- Notify me via email or RSS
- All Collections
- Expert Gallery
- Theses & Dissertations
- Selected Works Gallery
- Student Works
- Conferences
- Open Access Fund Collection
- Historic Collections

Useful Links
- Author Resources
- Author Rights
- Scholarly Publishing Information
- Graduate School
Home | About | FAQ | My Account | Accessibility Statement
Privacy Copyright
How Immunotherapy Can Treat Cancer and Other Diseases: 8 Things to Know
BY KATHY KATELLA , JEREMY LEDGER March 5, 2024
If you were diagnosed with a serious disease, one of your first questions might be, “How is it treated?” For a growing number of patients, the answer is immunotherapy, which works by using the body’s own immune system to fight the disease.
Immunotherapy has been gaining traction over the years in two areas: cancer and autoimmune diseases. For instance, immunotherapy can help the immune system identify and destroy invading cancer cells. For autoimmune diseases like rheumatoid arthritis (RA) and multiple sclerosis (MS) , in which the body mistakenly attacks its own cells—immunotherapy can tamp down the immune system’s power, usually without difficult side effects.
Immunotherapy doesn’t help everyone or every disease, but when it works well, the results can be dramatic. “It’s been absolutely a game-changer,” says David Hafler, MD , chair of the Department of Neurology and an expert on autoimmune diseases, including MS.
Mario Sznol, MD , a Yale Medicine oncologist and co-leader of the Cancer Immunology Program at Yale Cancer Center, agrees and is just as positive about immunotherapy’s use for multiple types of cancer. “We're still a long way from immunotherapy curing cancer without side effects in 100% of patients,” he says. “But even based on what we know today, some patients are getting long, durable responses that would have been impossible in the past."
Below, Drs. Hafler and Sznol help us answer eight questions to help people understand how immunotherapy is making a difference in the treatment of cancer and autoimmune diseases.
1. What is the immune system, and why is it important?
The immune system is made up of a group of organs, cells, and proteins that work together to fight off pathogens—a catchall term that refers to disease-causing microbes, including bacteria, viruses, parasites, and fungi. The immune system further protects against toxins that some pathogens produce, and it also detects and kills cells that are infected by a pathogen or have become cancerous. In short, it’s a system that aims to keep you healthy and free of disease by recognizing and eliminating infectious organisms or cells that don't belong in your body.
When the immune system encounters a pathogen for the first time, certain immune cells detect its antigens (the tiny structures that protrude from its surface) and recognize it as a dangerous invader. The immune cells attach to the pathogen and “activate,” producing thousands of copies of themselves, as well as thousands of antibodies.
At this point, the immune cells and antibodies attack and destroy the pathogenic invaders. If any of the body’s cells have been infected, the immune system kills them to stop the pathogen from spreading throughout the body.
Once the pathogen has been cleared, the immune cells and antibodies begin to decline in number. But a few specialized memory cells remain. These cells preserve a record of the pathogen, so if the immune system ever encounters that pathogen again, it can launch a rapid, powerful response against it.
2. What is immunotherapy?
Immunotherapy is an umbrella term that encompasses different approaches to harnessing the immune system to fight disease. This includes widely used therapies that have been employed for years to treat allergies and stop the body from rejecting transplanted organs.
In recent years, research has focused on the use of immunotherapy to treat cancer. Experts know the immune system can work against cancer as it does against the flu, for example—but not as effectively, Dr. Sznol says. “Cancer cells have ways to outsmart the immune system—sometimes by blocking its ability to recognize them. So, we use immunotherapy to modify the immune system in ways that enable it to work better.” The end goal for its use in cancer care is to push the body’s immune system to better identify and destroy the cancer cells, he adds.
For autoimmune diseases, the task is different. There are more than 80 known diseases, including lupus, in which the immune system becomes overactive to the point that it starts mistakenly attacking healthy cells in the body’s organs and tissues. Immunotherapy can suppress—dampen or put the brakes on—an overactive immune system, minimizing symptoms of a disease and even curing it in some cases.
3. How is immunotherapy administered?
Immunotherapies are delivered in a variety of ways. Depending on the particular treatment, it may be given orally—in pill form—or by intravenous (IV) infusion, which may be given multiple times in a hospital setting. Some treatments are injected under the skin like a flu shot.
Some therapies for cancer involve harvesting cells and then engineering them outside the body to better fight the disease. For this, the patient undergoes a chemotherapy preparative regimen to clear their body of lymphocytes, cells that are central to engaging the immune system. Then, those cells are replaced by the more powerful engineered cells in a single IV treatment.
Patients with certain types of cancer may be given immunotherapy alone, while others may be treated with combined immunotherapy and chemotherapy, for instance.
4. What types of immunotherapy are there?
If a person is eligible for treatment with immunotherapy, the type of treatment offered depends on a number of factors, including their condition (not all diseases can be treated with immunotherapy). And the length of treatment varies widely. For cancer, treatment might mean a single dose or multiple treatments given over the course of one or two years. For autoimmune diseases, such as MS, treatment may be long-term, continuing for over a decade for many patients.
Types of immunotherapy include (but are not limited to):
- Immune checkpoint inhibitors: Checkpoints are a natural part of the immune system that keep immune responses from being so strong that they destroy healthy cells. But this can also inhibit the immune response. As the name implies, immune checkpoint inhibitors block those checkpoints so the immune system can more effectively find and attack cancer cells.
- Adoptive cell therapy (or T-cell therapy): This involves removing immune cells called lymphocytes (which are key cancer fighters) from the body so that they can be genetically engineered in a laboratory to enhance their ability to recognize, bind to, and kill cancer cells. The revamped cells are injected back into the body to attack cancer.
- CAR T-cell therapy: This is a promising type of adoptive cell therapy in which lymphocytes are changed to make a protein called chimeric antigen receptor (CAR) that—once injected back into the body—gives these cells the ability to specifically bind to cancer cells that have the target for CAR on their surface. For certain kinds of cell therapy—for example, TIL cells—the lymphocytes are removed from a patient’s cancer and are already capable of recognizing the tumor cells, but they need to be expanded and activated outside the body. More recently, cell therapy with lymphocytes is being used to treat autoimmune diseases by using CAR-T, which can recognize and kill the immune cells in the body that are attacking normal tissues.
- Monoclonal antibodies (mAbs): These are laboratory-made antibodies that mimic those found in the body. (Since monoclonal means “one type,” a treatment would mean multiple copies of one kind of antibody). They work in different ways. For cancer treatment, they are designed to trigger the immune system to attack cancer cells. For autoimmune diseases, they block inflammatory proteins—for example, interleukin-6—to improve inflammation-related symptoms, such as the joint pain and swelling associated with arthritis.
5. What types of cancers can be treated with immunotherapy?
There are several Food and Drug Administration (FDA)-approved immunotherapies to treat certain types of cancers. Some of these are now used as standard treatments for such diseases as bladder, head and neck, esophageal, lung, and renal cell cancer.
But “ melanoma is the ‘poster child’ for cancer immunotherapy; it is the disease where it has worked best,” Dr. Sznol says. In fact, the first immunotherapy for cancer was a checkpoint inhibitor for melanoma, which was approved by the FDA in 2011. Part of the reason for its success is that melanoma cells contain many mutations, and the immune system sees those cells as foreign invaders and reacts with stronger and more diverse immune responses. Checkpoint inhibitors unleash these immune responses so they can attack the melanoma cells.
Before immunotherapy became available, just 5% of people with advanced melanoma (melanoma that spreads internally to other organs) survived five years or longer; now, with immunotherapy as a treatment option, that figure is close to 50% or more, Dr. Sznol says. What’s even more remarkable is that many people with melanoma who are given immunotherapy and survive their cancer for at least five years will never relapse or require additional treatment, he adds.
But Dr. Sznol cautions that some cancers respond better to immunotherapy than others. “Even with melanoma, it’s important to remember that only half of the patients will respond to immunotherapies, whereas with some other cancers, it might only be 15% or 20% of patients,” he says.
Many immunotherapy treatments for cancer are still being researched, and patients may have access to those in clinical trials.
6. How well does immunotherapy work against autoimmune diseases?
As noted above, there are more than 80 distinct autoimmune diseases—and there are immunotherapies for some of the most common ones, including MS, RA, and psoriasis . When the immune system goes awry and attacks the body’s healthy tissues, it causes inflammation and other complications that exacerbate the disease. Immunotherapy drugs lower that activity, targeting the specific components of the immune system contributing to the disease.
The approach is different from some conventional autoimmune disease treatments that suppress general immune function, which makes people vulnerable to disease-causing bacteria and viruses.
Different immunotherapies target different autoimmune conditions. For instance, MS causes lesions on the brain and spinal cord, Dr. Hafler explains. “The immunotherapy drugs don't make you feel better or fix your existing lesions, but they prevent new ones from occurring,” he says.
For type 1 diabetes , an immunotherapy drug called teplizumab (brand name: Tzield®) was approved by the FDA in 2022. It can delay the onset of an advanced stage of type 1 disease in people as young as 8 years old.
“Similar to cancer, it’s important to remember that immunotherapies for autoimmune diseases have varying degrees of efficacy,” Dr. Hafler says. For example, ocrelizumab, a monoclonal antibody, has 98% efficacy against MS, while adalimumab (Humira®), the most common treatment for RA, may be 60% effective or less, he says.
7. What are the side effects of immunotherapy?
Immunotherapy is often well-tolerated, according to the doctors. As with many drugs, there may be side effects, such as dizziness, fatigue, diarrhea, rash, nausea, joint pain, and weakness. Because immunotherapy can unleash immune reactions that can attack any normal healthy tissue, some patients may develop very severe reactions that affect the colon, kidneys, liver, lungs, heart, endocrine glands, and other internal organs, which require urgent treatment and could even lead to death.
Fortunately, very severe, life-threatening reactions are rare. These can depend on the type of immunotherapy and such factors as a patient’s general health and how advanced the disease is.
For the vast majority of people, most side effects can be reversed, Dr. Sznol says. But if side effects are serious—or if they could become serious—the patient may need a treatment, such as steroids, to “turn off” the immune system. “Generally, treatment with steroids or other immunosuppressants is time-limited; once the side effect resolves, the steroids can be stopped, and the side effects will not recur unless the patient is again treated with a similar type of immunotherapy,” Dr. Sznol says.
For autoimmune diseases, side effects from immunotherapy are usually minimal, Dr. Hafler says, adding that this is a vast improvement over earlier treatments—or, in some cases, no effective treatments at all.
Patients with MS were once treated with a chemotherapy drug called cyclophosphamide, an immune suppression treatment for patients with severe disease, which had such side effects as hair loss and infertility in the short term to an increased risk of leukemia in the long term. “We don't use that anymore,” Dr. Hafler says.
8. What should patients ask their doctors about immunotherapy?
The first step is to consult an appropriate specialist, such as an oncologist or autoimmune disease specialist experienced in the condition you have, the doctors say. “You should ask them, ‘Is immunotherapy a part of my treatment? How is it going to benefit me if I take it?’” Dr. Sznol says.
Not every disease will have an immunotherapy treatment. With cancer, chemotherapy , radiation , and surgery are still key treatment pillars for many patients.
But immunotherapy research is ongoing for a variety of cancers and autoimmune conditions. Scientists are studying how long patients need to be treated with particular immunotherapies, whether they work better when combined with another treatment for particular conditions, and how to determine who will have the best results.
In addition, scientists are studying how to make the treatments more effective for more people with the help of personalized medicine, in which treatments are customized for individual patients based on their genetic profile. “In this endeavor, I think genetic screening will be very useful in helping to get the right drug to the right patient,” Dr. Hafler says.
“The more experts learn, the better equipped they will be to use immunotherapy to treat patients,” Dr. Sznol says.
More news from Yale Medicine
Thank you for visiting nature.com. You are using a browser version with limited support for CSS. To obtain the best experience, we recommend you use a more up to date browser (or turn off compatibility mode in Internet Explorer). In the meantime, to ensure continued support, we are displaying the site without styles and JavaScript.
- View all journals
- Explore content
- About the journal
- Publish with us
- Sign up for alerts
- Review Article
- Published: 13 May 2024
Cancer therapy with antibodies
- Suman Paul ORCID: orcid.org/0000-0001-9357-3702 1 ,
- Maximilian F. Konig ORCID: orcid.org/0000-0001-5045-5255 2 ,
- Drew M. Pardoll 1 ,
- Chetan Bettegowda 3 ,
- Nickolas Papadopoulos 1 ,
- Katharine M. Wright 4 ,
- Sandra B. Gabelli ORCID: orcid.org/0000-0003-1205-5204 4 ,
- Mitchell Ho ORCID: orcid.org/0000-0002-9152-5405 5 ,
- Andrea van Elsas ORCID: orcid.org/0009-0001-3426-2653 6 &
- Shibin Zhou ORCID: orcid.org/0000-0003-1941-4425 1
Nature Reviews Cancer ( 2024 ) Cite this article
1105 Accesses
90 Altmetric
Metrics details
- Antibody therapy
- Cancer immunotherapy
- Cancer therapy
- Drug development
The greatest challenge in cancer therapy is to eradicate cancer cells with minimal damage to normal cells. Targeted therapy has been developed to meet that challenge, showing a substantially increased therapeutic index compared with conventional cancer therapies. Antibodies are important members of the family of targeted therapeutic agents because of their extraordinarily high specificity to the target antigens. Therapeutic antibodies use a range of mechanisms that directly or indirectly kill the cancer cells. Early antibodies were developed to directly antagonize targets on cancer cells. This was followed by advancements in linker technologies that allowed the production of antibody–drug conjugates (ADCs) that guide cytotoxic payloads to the cancer cells. Improvement in our understanding of the biology of T cells led to the production of immune checkpoint-inhibiting antibodies that indirectly kill the cancer cells through activation of the T cells. Even more recently, bispecific antibodies were synthetically designed to redirect the T cells of a patient to kill the cancer cells. In this Review, we summarize the different approaches used by therapeutic antibodies to target cancer cells. We discuss their mechanisms of action, the structural basis for target specificity, clinical applications and the ongoing research to improve efficacy and reduce toxicity.
This is a preview of subscription content, access via your institution
Access options
Access Nature and 54 other Nature Portfolio journals
Get Nature+, our best-value online-access subscription
24,99 € / 30 days
cancel any time
Subscribe to this journal
Receive 12 print issues and online access
195,33 € per year
only 16,28 € per issue
Buy this article
- Purchase on Springer Link
- Instant access to full article PDF
Prices may be subject to local taxes which are calculated during checkout
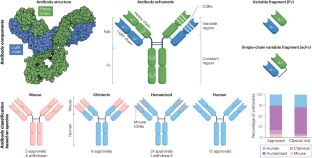
Similar content being viewed by others
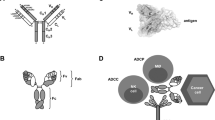
Antibody-based cancer therapy
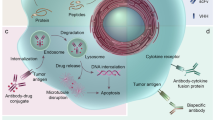
Emerging new therapeutic antibody derivatives for cancer treatment
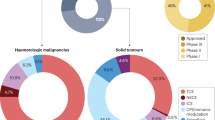
The present and future of bispecific antibodies for cancer therapy
Data availability.
The data used to make Figs. 1 and 6 are available from the American Cancer Society and the Antibody Society .
Sternberger, L. A. & Sternberger, N. H. Monoclonal antibodies distinguish phosphorylated and nonphosphorylated forms of neurofilaments in situ. Proc. Natl Acad. Sci. USA 80 , 6126–6130 (1983).
Article CAS PubMed PubMed Central Google Scholar
Stark, S. E. & Caton, A. J. Antibodies that are specific for a single amino acid interchange in a protein epitope use structurally distinct variable regions. J. Exp. Med. 174 , 613–624 (1991).
Article CAS PubMed Google Scholar
Antibody therapeutics approved or in regulatory review in the EU or US. Antibody Society www.antibodysociety.org/resources/approved-antibodies (2023). The Antibody Society is an association that supports research and development of antibody-based drugs and maintains an updated list of antibodies approved by the FDA and EMA.
June, C. H. & Sadelain, M. Chimeric antigen receptor therapy. N. Engl. J. Med. 379 , 64–73 (2018).
Labanieh, L. & Mackall, C. L. CAR immune cells: design principles, resistance and the next generation. Nature 614 , 635–648 (2023).
Carter, P. Improving the efficacy of antibody-based cancer therapies. Nat. Rev. Cancer 1 , 118–129 (2001).
Weiner, G. J. Building better monoclonal antibody-based therapeutics. Nat. Rev. Cancer 15 , 361–370 (2015).
Ho, M. Inaugural editorial: searching for magic bullets. Antib. Ther. 1 , 1–5 (2018).
CAS PubMed PubMed Central Google Scholar
Kohler, G. & Milstein, C. Continuous cultures of fused cells secreting antibody of predefined specificity. Nature 256 , 495–497 (1975). This seminal paper describes the hybridoma technology that enabled the production of monoclonal antibodies. Kohler and Milstein received the Nobel Prize for this work.
Hwang, W. Y. & Foote, J. Immunogenicity of engineered antibodies. Methods 36 , 3–10 (2005).
Reff, M. E. et al. Depletion of B cells in vivo by a chimeric mouse human monoclonal antibody to CD20. Blood 83 , 435–445 (1994). This paper describes the generation of rituximab, the first chimeric antibody that demonstrated significant tumour reduction and later became the standard of care for the treatment of patients with B cell lymphomas.
Goldstein, N. I., Prewett, M., Zuklys, K., Rockwell, P. & Mendelsohn, J. Biological efficacy of a chimeric antibody to the epidermal growth factor receptor in a human tumor xenograft model. Clin. Cancer Res. 1 , 1311–1318 (1995).
CAS PubMed Google Scholar
Looney, R. J. et al. B cell depletion as a novel treatment for systemic lupus erythematosus: a phase I/II dose-escalation trial of rituximab. Arthritis Rheum. 50 , 2580–2589 (2004).
Jones, P. T., Dear, P. H., Foote, J., Neuberger, M. S. & Winter, G. Replacing the complementarity-determining regions in a human antibody with those from a mouse. Nature 321 , 522–525 (1986). This paper describes the process of CDR grafting that enabled the production of humanized antibodies. The majority of cancer antibodies utilize the humanized antibody format.
Carter, P. et al. Humanization of an anti-p185HER2 antibody for human cancer therapy. Proc. Natl Acad. Sci. USA 89 , 4285–4289 (1992).
Spieker-Polet, H., Sethupathi, P., Yam, P. C. & Knight, K. L. Rabbit monoclonal antibodies: generating a fusion partner to produce rabbit-rabbit hybridomas. Proc. Natl Acad. Sci. USA 92 , 9348–9352 (1995).
Zhang, Y. F. & Ho, M. Humanization of rabbit monoclonal antibodies via grafting combined Kabat/IMGT/Paratome complementarity-determining regions: rationale and examples. MAbs 9 , 419–429 (2017).
Parray, H. A. et al. Hybridoma technology a versatile method for isolation of monoclonal antibodies, its applicability across species, limitations, advancement and future perspectives. Int. Immunopharmacol. 85 , 106639 (2020).
McCafferty, J., Griffiths, A. D., Winter, G. & Chiswell, D. J. Phage antibodies: filamentous phage displaying antibody variable domains. Nature 348 , 552–554 (1990). This paper describes the early phage display technologies that enabled the selection of antibodies against a range of antigens including cancer antigens. G. Smith and G. Winter received the Nobel Prize for their work on phage display-based antibody production.
Lonberg, N. et al. Antigen-specific human antibodies from mice comprising four distinct genetic modifications. Nature 368 , 856–859 (1994). This paper describes the development of transgenic mouse models that led to the production of fully human antibodies.
Lu, R. M. et al. Development of therapeutic antibodies for the treatment of diseases. J. Biomed. Sci. 27 , 1 (2020).
Winter, G., Griffiths, A. D., Hawkins, R. E. & Hoogenboom, H. R. Making antibodies by phage display technology. Annu. Rev. Immunol. 12 , 433–455 (1994).
Lu, S. et al. The rapid and highly parallel identification of antibodies with defined biological activities by SLISY. Nat. Commun. 14 , 17 (2023).
Alfaleh, M. A. et al. Phage display derived monoclonal antibodies: from bench to bedside. Front. Immunol. 11 , 1986 (2020).
Almagro, J. C., Daniels-Wells, T. R., Perez-Tapia, S. M. & Penichet, M. L. Progress and challenges in the design and clinical development of antibodies for cancer therapy. Front. Immunol. 8 , 1751 (2017).
Article PubMed Google Scholar
Booth, B. Human antibody discovery: of mice and phage. Forbes https://www.forbes.com/sites/brucebooth/2017/05/11/human-antibody-discovery-of-mice-and-phage/?sh=1f76520c7f26 (2017).
Jain, T. et al. Biophysical properties of the clinical-stage antibody landscape. Proc. Natl Acad. Sci. USA 114 , 944–949 (2017).
Douglass, J. et al. Bispecific antibodies targeting mutant RAS neoantigens. Sci. Immunol. 6 , eabd5515 (2021).
Hsiue, E. H. et al. Targeting a neoantigen derived from a common TP53 mutation. Science 371 , eabc8697 (2021). This paper describes the generation of bispecific antibodies targeting the most common oncogenic mutation (R175H) in the tumour suppressor protein p53.
Boder, E. T., Midelfort, K. S. & Wittrup, K. D. Directed evolution of antibody fragments with monovalent femtomolar antigen-binding affinity. Proc. Natl Acad. Sci. USA 97 , 10701–10705 (2000).
Georgiou, G. et al. Display of heterologous proteins on the surface of microorganisms: from the screening of combinatorial libraries to live recombinant vaccines. Nat. Biotechnol. 15 , 29–34 (1997).
Ho, M., Nagata, S. & Pastan, I. Isolation of anti-CD22 Fv with high affinity by Fv display on human cells. Proc. Natl Acad. Sci. USA 103 , 9637–9642 (2006).
Lipovsek, D. & Pluckthun, A. In-vitro protein evolution by ribosome display and mRNA display. J. Immunol. Methods 290 , 51–67 (2004).
Wang, J. et al. Durable blockade of PD-1 signaling links preclinical efficacy of sintilimab to its clinical benefit. MAbs 11 , 1443–1451 (2019).
Marcus, R. et al. Obinutuzumab for the first-line treatment of follicular lymphoma. N. Engl. J. Med. 377 , 1331–1344 (2017).
Vitolo, U. et al. Obinutuzumab or rituximab plus cyclophosphamide, doxorubicin, vincristine, and prednisone in previously untreated diffuse large B-cell lymphoma. J. Clin. Oncol. 35 , 3529–3537 (2017).
van Imhoff, G. W. et al. Ofatumumab versus rituximab salvage chemoimmunotherapy in relapsed or refractory diffuse large B-cell lymphoma: the ORCHARRD study. J. Clin. Oncol. 35 , 544–551 (2017).
Maloney, D. G. et al. A phase 3 randomized study (HOMER) of ofatumumab vs rituximab in iNHL relapsed after rituximab-containing therapy. Blood Adv. 4 , 3886–3893 (2020).
Rugo, H. S. et al. Margetuximab versus trastuzumab in patients with previously treated HER2-positive advanced breast cancer (SOPHIA): final overall survival results from a randomized phase 3 trial. J. Clin. Oncol. 41 , 198–205 (2023).
Price, T. J. et al. Panitumumab versus cetuximab in patients with chemotherapy-refractory wild-type KRAS exon 2 metastatic colorectal cancer (ASPECCT): a randomised, multicentre, open-label, non-inferiority phase 3 study. Lancet Oncol. 15 , 569–579 (2014).
Furman, W. L. Monoclonal antibody therapies for high risk neuroblastoma. Biologics 15 , 205–219 (2021).
PubMed PubMed Central Google Scholar
Peter, H. H. et al. Targeting FcRn for immunomodulation: benefits, risks, and practical considerations. J. Allergy Clin. Immunol. 146 , 479–491.e5 (2020).
Roopenian, D. C. & Akilesh, S. FcRn: the neonatal Fc receptor comes of age. Nat. Rev. Immunol. 7 , 715–725 (2007).
Slamon, D. J. et al. Use of chemotherapy plus a monoclonal antibody against HER2 for metastatic breast cancer that overexpresses HER2. N. Engl. J. Med. 344 , 783–792 (2001). This is a groundbreaking clinical trial demonstrating the survival benefit of the HER2-targeting antibody trastuzumab in patients with breast cancer. Slamon received the Lasker–DeBakey award for his work related to HER2 targeting in breast cancer.
Bang, Y. J. et al. Trastuzumab in combination with chemotherapy versus chemotherapy alone for treatment of HER2-positive advanced gastric or gastro-oesophageal junction cancer (ToGA): a phase 3, open-label, randomised controlled trial. Lancet 376 , 687–697 (2010).
Cunningham, D. et al. Cetuximab monotherapy and cetuximab plus irinotecan in irinotecan-refractory metastatic colorectal cancer. N. Engl. J. Med. 351 , 337–345 (2004).
Camidge, D. R. et al. Phase I study of 2- or 3-week dosing of telisotuzumab vedotin, an antibody-drug conjugate targeting c-Met, monotherapy in patients with advanced non-small cell lung carcinoma. Clin. Cancer Res. 27 , 5781–5792 (2021).
Redman, J. M., Hill, E. M., AlDeghaither, D. & Weiner, L. M. Mechanisms of action of therapeutic antibodies for cancer. Mol. Immunol. 67 , 28–45 (2015).
Mone, A. P. et al. Alemtuzumab induces caspase-independent cell death in human chronic lymphocytic leukemia cells through a lipid raft-dependent mechanism. Leukemia 20 , 272–279 (2006).
Meyer, S. et al. New insights in type I and II CD20 antibody mechanisms-of-action with a panel of novel CD20 antibodies. Br. J. Haematol. 180 , 808–820 (2018).
Vidarsson, G., Dekkers, G. & Rispens, T. IgG subclasses and allotypes: from structure to effector functions. Front. Immunol. 5 , 520 (2014).
Article PubMed PubMed Central Google Scholar
Salfeld, J. G. Isotype selection in antibody engineering. Nat. Biotechnol. 25 , 1369–1372 (2007).
Yu, J., Song, Y. & Tian, W. How to select IgG subclasses in developing anti-tumor therapeutic antibodies. J. Hematol. Oncol. 13 , 45 (2020).
Wang, X., Mathieu, M. & Brezski, R. J. IgG Fc engineering to modulate antibody effector functions. Protein Cell 9 , 63–73 (2018).
Weng, W. K. & Levy, R. Two immunoglobulin G fragment C receptor polymorphisms independently predict response to rituximab in patients with follicular lymphoma. J. Clin. Oncol. 21 , 3940–3947 (2003).
Musolino, A. et al. Immunoglobulin G fragment C receptor polymorphisms and clinical efficacy of trastuzumab-based therapy in patients with HER-2/neu-positive metastatic breast cancer. J. Clin. Oncol. 26 , 1789–1796 (2008).
Golay, J. & Taylor, R. P. The role of complement in the mechanism of action of therapeutic anti-cancer mAbs. Antibodies 9 , 58 (2020).
Schneider-Merck, T. et al. Human IgG2 antibodies against epidermal growth factor receptor effectively trigger antibody-dependent cellular cytotoxicity but, in contrast to IgG1, only by cells of myeloid lineage. J. Immunol. 184 , 512–520 (2010).
Rosner, T. et al. Immune effector functions of human IgG2 antibodies against EGFR. Mol. Cancer Ther. 18 , 75–88 (2019).
Cho, H. S. et al. Structure of the extracellular region of HER2 alone and in complex with the Herceptin Fab. Nature 421 , 756–760 (2003). This study of early structural biology has improved our understanding of trastuzumab binding to the HER2 extracellular subdomain 4 to inhibit HER2 function.
Hao, Y., Yu, X., Bai, Y., McBride, H. J. & Huang, X. Cryo-EM structure of HER2-trastuzumab-pertuzumab complex. PLoS ONE 14 , e0216095 (2019).
Weisser, N. E. et al. An anti-HER2 biparatopic antibody that induces unique HER2 clustering and complement-dependent cytotoxicity. Nat. Commun. 14 , 1394 (2023).
Rouge, L. et al. Structure of CD20 in complex with the therapeutic monoclonal antibody rituximab. Science 367 , 1224–1230 (2020).
Liu, R., Oldham, R. J., Teal, E., Beers, S. A. & Cragg, M. S. Fc-engineering for modulated effector functions-improving antibodies for cancer treatment. Antibodies 9 , 64 (2020).
Nimmerjahn, F., Vidarsson, G. & Cragg, M. S. Effect of posttranslational modifications and subclass on IgG activity: from immunity to immunotherapy. Nat. Immunol. 24 , 1244–1255 (2023). This review describes the Fc domain modifications that alter IgG antibody effector functions.
Rugo, H. S. et al. Efficacy of margetuximab vs trastuzumab in patients with pretreated ERBB2-positive advanced breast cancer: a phase 3 randomized clinical trial. JAMA Oncol. 7 , 573–584 (2021).
Ribas, A. & Wolchok, J. D. Cancer immunotherapy using checkpoint blockade. Science 359 , 1350–1355 (2018).
Le, D. T. et al. PD-1 blockade in tumors with mismatch-repair deficiency. N. Engl. J. Med. 372 , 2509–2520 (2015).
Le, D. T. et al. Mismatch repair deficiency predicts response of solid tumors to PD-1 blockade. Science 357 , 409–413 (2017). This paper demonstrates the utility of the PD1 antibody, pembrolizumab, in a range of cancer types with mismatch repair deficiency. This led to the FDA approval of pembrolizumab in any cancer with mismatch repair deficiency which was the first tissue agnostic drug approval in oncology.
Gandhi, L. et al. Pembrolizumab plus chemotherapy in metastatic non-small-cell lung cancer. N. Engl. J. Med. 378 , 2078–2092 (2018).
Marabelle, A. et al. Association of tumour mutational burden with outcomes in patients with advanced solid tumours treated with pembrolizumab: prospective biomarker analysis of the multicohort, open-label, phase 2 KEYNOTE-158 study. Lancet Oncol. 21 , 1353–1365 (2020).
Nghiem, P. T. et al. PD-1 blockade with pembrolizumab in advanced Merkel-cell carcinoma. N. Engl. J. Med. 374 , 2542–2552 (2016).
Sharma, P. et al. The next decade of immune checkpoint therapy. Cancer Discov. 11 , 838–857 (2021).
Chamoto, K., Yaguchi, T., Tajima, M. & Honjo, T. Insights from a 30-year journey: function, regulation and therapeutic modulation of PD1. Nat. Rev. Immunol. 23 , 682–695 (2023). Together with Sharma et al. (2021), this review describes the mechanism of immune checkpoint-inhibiting antibodies in cancer. Allison and Honjo jointly received the Nobel Prize for their discoveries of a cancer therapy by inhibition of the immune checkpoint PD1.
Huard, B. et al. Characterization of the major histocompatibility complex class II binding site on LAG-3 protein. Proc. Natl Acad. Sci. USA 94 , 5744–5749 (1997).
Wang, J. et al. Fibrinogen-like protein 1 is a major immune inhibitory ligand of LAG-3. Cell 176 , 334–347.e12 (2019).
Blackburn, S. D., Shin, H., Freeman, G. J. & Wherry, E. J. Selective expansion of a subset of exhausted CD8 T cells by alphaPD-L1 blockade. Proc. Natl Acad. Sci. USA 105 , 15016–15021 (2008).
Im, S. J. et al. Defining CD8+ T cells that provide the proliferative burst after PD-1 therapy. Nature 537 , 417–421 (2016).
Yost, K. E. et al. Clonal replacement of tumor-specific T cells following PD-1 blockade. Nat. Med. 25 , 1251–1259 (2019).
Romano, E. et al. Ipilimumab-dependent cell-mediated cytotoxicity of regulatory T cells ex vivo by nonclassical monocytes in melanoma patients. Proc. Natl Acad. Sci. USA 112 , 6140–6145 (2015).
Kim, M. J. et al. Deletion of PD-1 destabilizes the lineage identity and metabolic fitness of tumor-infiltrating regulatory T cells. Nat. Immunol. 24 , 148–161 (2023).
Sharma, A. et al. Anti-CTLA-4 immunotherapy does not deplete FOXP3 + regulatory T cells (Tregs) in human cancers. Clin. Cancer Res. 25 , 1233–1238 (2019).
Kamada, T. et al. PD-1 + regulatory T cells amplified by PD-1 blockade promote hyperprogression of cancer. Proc. Natl Acad. Sci. USA 116 , 9999–10008 (2019).
Kumagai, S. et al. The PD-1 expression balance between effector and regulatory T cells predicts the clinical efficacy of PD-1 blockade therapies. Nat. Immunol. 21 , 1346–1358 (2020).
Scapin, G. et al. Structure of full-length human anti-PD1 therapeutic IgG4 antibody pembrolizumab. Nat. Struct. Mol. Biol. 22 , 953–958 (2015).
You, W. et al. A network meta-analysis comparing the efficacy and safety of anti-PD-1 with anti-PD-L1 in non-small cell lung cancer. J. Cancer 9 , 1200–1206 (2018).
Barlesi, F. et al. Avelumab versus docetaxel in patients with platinum-treated advanced non-small-cell lung cancer (JAVELIN Lung 200): an open-label, randomised, phase 3 study. Lancet Oncol. 19 , 1468–1479 (2018).
Lee, N. Y. et al. Avelumab plus standard-of-care chemoradiotherapy versus chemoradiotherapy alone in patients with locally advanced squamous cell carcinoma of the head and neck: a randomised, double-blind, placebo-controlled, multicentre, phase 3 trial. Lancet Oncol. 22 , 450–462 (2021).
Monk, B. J. et al. Chemotherapy with or without avelumab followed by avelumab maintenance versus chemotherapy alone in patients with previously untreated epithelial ovarian cancer (JAVELIN Ovarian 100): an open-label, randomised, phase 3 trial. Lancet Oncol. 22 , 1275–1289 (2021).
Bang, Y. J. et al. Phase III, randomised trial of avelumab versus physician’s choice of chemotherapy as third-line treatment of patients with advanced gastric or gastro-oesophageal junction cancer: primary analysis of JAVELIN Gastric 300. Ann. Oncol. 29 , 2052–2060 (2018).
Lee, J. Y. et al. Structural basis of checkpoint blockade by monoclonal antibodies in cancer immunotherapy. Nat. Commun. 7 , 13354 (2016).
Lee, H. T. et al. Molecular mechanism of PD-1/PD-L1 blockade via anti-PD-L1 antibodies atezolizumab and durvalumab. Sci. Rep. 7 , 5532 (2017).
Lee, H. T., Lee, S. H. & Heo, Y. S. Molecular interactions of antibody drugs targeting PD-1, PD-L1, and CTLA-4 in immuno-oncology. Molecules 24 , 1190 (2019).
Lin, D. Y. et al. The PD-1/PD-L1 complex resembles the antigen-binding Fv domains of antibodies and T cell receptors. Proc. Natl Acad. Sci. USA 105 , 3011–3016 (2008).
Liu, K. et al. Structural basis of anti-PD-L1 monoclonal antibody avelumab for tumor therapy. Cell Res. 27 , 151–153 (2017).
Tang, S. & Kim, P. S. A high-affinity human PD-1/PD-L2 complex informs avenues for small-molecule immune checkpoint drug discovery. Proc. Natl Acad. Sci. USA 116 , 24500–24506 (2019).
He, M. et al. Remarkably similar CTLA-4 binding properties of therapeutic ipilimumab and tremelimumab antibodies. Oncotarget 8 , 67129–67139 (2017).
Brahmer, J. R. et al. Management of immune-related adverse events in patients treated with immune checkpoint inhibitor therapy: American Society of Clinical Oncology Clinical Practice Guideline. J. Clin. Oncol. 36 , 1714–1768 (2018).
Wang, D. Y. et al. Fatal toxic effects associated with immune checkpoint inhibitors: a systematic review and meta-analysis. JAMA Oncol. 4 , 1721–1728 (2018).
Runcie, K., Budman, D. R., John, V. & Seetharamu, N. Bi-specific and tri-specific antibodies — the next big thing in solid tumor therapeutics. Mol. Med. 24 , 50 (2018).
Bargou, R. et al. Tumor regression in cancer patients by very low doses of a T cell-engaging antibody. Science 321 , 974–977 (2008). This paper describes the first-in-human clinical trial of the CD19-targeting bispecific antibody blinatumomab, which was the first bispecific antibody to achieve widespread adoption in the clinic and subsequently set the stage for the widespread adoption of the bispecific antibody format.
Labrijn, A. F., Janmaat, M. L., Reichert, J. M. & Parren, P. Bispecific antibodies: a mechanistic review of the pipeline. Nat. Rev. Drug Discov. 18 , 585–608 (2019). This detailed review describes the different formats of bispecific antibodies.
Liddy, N. et al. Monoclonal TCR-redirected tumor cell killing. Nat. Med. 18 , 980–987 (2012).
Nathan, P. et al. Overall survival benefit with tebentafusp in metastatic uveal melanoma. N. Engl. J. Med. 385 , 1196–1206 (2021).
Ahn, M. J. et al. Tarlatamab for patients with previously treated small-cell lung cancer. N. Engl. J. Med. 389 , 2063–2075 (2023).
Park, K. et al. Amivantamab in EGFR exon 20 insertion-mutated non-small-cell lung cancer progressing on platinum chemotherapy: initial results from the CHRYSALIS phase I study. J. Clin. Oncol. 39 , 3391–3402 (2021).
Moores, S. L. et al. A novel bispecific antibody targeting EGFR and cMet is effective against EGFR inhibitor-resistant lung tumors. Cancer Res. 76 , 3942–3953 (2016).
Dixit, S., Abraham, L., Weiser, N. & Gold, M. R. Super-resolution imaging studies of zanidatamab: providing insights into its bispecific mode of action. Cancer Res. 81 , abstr. 1032 (2021).
Article Google Scholar
Weiser, N. E. et al. The bispecific antibody zanidatamab’s (ZW25’s) unique mechanisms of action and durable anti-tumor activity in HER2-expressing cancers. Cancer Res . 81 , abstr. 1005 (2021).
Meric-Bernstam, F. et al. Zanidatamab, a novel bispecific antibody, for the treatment of locally advanced or metastatic HER2-expressing or HER2-amplified cancers: a phase 1, dose-escalation and expansion study. Lancet Oncol. 23 , 1558–1570 (2022).
Falchi, L., Vardhana, S. A. & Salles, G. A. Bispecific antibodies for the treatment of B-cell lymphoma: promises, unknowns, and opportunities. Blood 141 , 467–480 (2023).
Tsuchikama, K., Anami, Y., Ha, S. Y. Y. & Yamazaki, C. M. Exploring the next generation of antibody-drug conjugates. Nat. Rev. Clin. Oncol. 21 , 203–223 (2024). This review focuses on the current state and future directions of ADCs in oncology.
Fu, Z., Li, S., Han, S., Shi, C. & Zhang, Y. Antibody drug conjugate: the “biological missile” for targeted cancer therapy. Signal Transduct. Target. Ther. 7 , 93 (2022).
Stapleton, N. M. et al. Competition for FcRn-mediated transport gives rise to short half-life of human IgG3 and offers therapeutic potential. Nat. Commun. 2 , 599 (2011).
Natsume, A., Niwa, R. & Satoh, M. Improving effector functions of antibodies for cancer treatment: enhancing ADCC and CDC. Drug Des. Dev. Ther. 3 , 7–16 (2009).
CAS Google Scholar
Flygare, J. A., Pillow, T. H. & Aristoff, P. Antibody-drug conjugates for the treatment of cancer. Chem. Biol. Drug Des. 81 , 113–121 (2013).
Hingorani, D. V. et al. Monomethyl auristatin antibody and peptide drug conjugates for trimodal cancer chemo-radio-immunotherapy. Nat. Commun. 13 , 3869 (2022).
Francisco, J. A. et al. cAC10-vcMMAE, an anti-CD30-monomethyl auristatin E conjugate with potent and selective antitumor activity. Blood 102 , 1458–1465 (2003). This study describes the generation of a CD30-targeting ADC using a peptide cleavable linker. The ADC was later named brentuximab, and it is considered standard first-line therapy for patients with Hodgkin lymphoma and other CD30 + lymphomas. Multiple approved ADCs targeting other cancer antigens now use this drug–linker combination.
Ansell, S. M. et al. Overall survival with brentuximab vedotin in stage III or IV Hodgkin’s lymphoma. N. Engl. J. Med. 387 , 310–320 (2022).
Horwitz, S. et al. The ECHELON-2 trial: 5-year results of a randomized, phase III study of brentuximab vedotin with chemotherapy for CD30-positive peripheral T-cell lymphoma. Ann. Oncol. 33 , 288–298 (2022).
Zhang, D. et al. Catalytic cleavage of disulfide bonds in small molecules and linkers of antibody-drug conjugates. Drug Metab. Dispos. 47 , 1156–1163 (2019).
Moore, K. N. et al. Mirvetuximab soravtansine in FRα-positive, platinum-resistant ovarian cancer. N. Engl. J. Med. 389 , 2162–2174 (2023).
Kovtun, Y. V. et al. Antibody-drug conjugates designed to eradicate tumors with homogeneous and heterogeneous expression of the target antigen. Cancer Res. 66 , 3214–3221 (2006).
Oflazoglu, E. et al. Potent anticarcinoma activity of the humanized anti-CD70 antibody h1F6 conjugated to the tubulin inhibitor auristatin via an uncleavable linker. Clin. Cancer Res. 14 , 6171–6180 (2008).
Conilh, L., Sadilkova, L., Viricel, W. & Dumontet, C. Payload diversification: a key step in the development of antibody-drug conjugates. J. Hematol. Oncol. 16 , 3 (2023).
Ogitani, Y. et al. DS-8201a, a novel HER2-targeting ADC with a novel DNA topoisomerase I inhibitor, demonstrates a promising antitumor efficacy with differentiation from T-DM1. Clin. Cancer Res. 22 , 5097–5108 (2016). This paper describes the generation of tetrapeptide linker that masks the hydrophobicity of the payload and allows attachment of a large number of hydrophobic payloads without affecting antibody pharmacokinetics. This linker–drug combination is now being used in multiple ADCs that are nearing regulatory approval.
Zammarchi, F. et al. ADCT-402, a PBD dimer-containing antibody drug conjugate targeting CD19-expressing malignancies. Blood 131 , 1094–1105 (2018).
Lewis Phillips, G. D. et al. Targeting HER2-positive breast cancer with trastuzumab-DM1, an antibody-cytotoxic drug conjugate. Cancer Res. 68 , 9280–9290 (2008).
Cortes, J. et al. Trastuzumab deruxtecan versus trastuzumab emtansine for breast cancer. N. Engl. J. Med. 386 , 1143–1154 (2022). This phase III clinical trial directly compares two HER2-targeting ADCs, showing the benefit of the ADC trastuzumab deruxtecan, which carries the payload DXd attached by a cleavable linker and possesses a high DAR.
Modi, S. et al. Trastuzumab deruxtecan in previously treated HER2-low advanced breast cancer. N. Engl. J. Med. 387 , 9–20 (2022).
Kreitman, R. J. & Pastan, I. Immunotoxins: from design to clinical application. Biomolecules 11 , 1696 (2021).
Li, M., Mei, S., Yang, Y., Shen, Y. & Chen, L. Strategies to mitigate the on- and off-target toxicities of recombinant immunotoxins: an antibody engineering perspective. Antib. Ther. 5 , 164–176 (2022).
Kreitman, R. J. & Pastan, I. Antibody fusion proteins: anti-CD22 recombinant immunotoxin moxetumomab pasudotox. Clin. Cancer Res. 17 , 6398–6405 (2011). This review of immunotoxins shows the development of the first cancer-targeting immunotoxin moxetumomab that received FDA approval for the treatment of hairy cell leukaemia.
Lin, P., Qi, J. & Liu, W. Expert’s views and perspectives: an interview with distinguished investigator Dr. Ira Pastan at the National Cancer Institute at NIH. Antib. Ther. 3 , 163–166 (2020).
Kreitman, R. J. et al. Moxetumomab pasudotox in relapsed/refractory hairy cell leukemia. Leukemia 32 , 1768–1777 (2018).
Kreitman, R. J. & Pastan, I. Contextualizing the use of moxetumomab pasudotox in the treatment of relapsed or refractory hairy cell leukemia. Oncologist 25 , e170–e177 (2020).
Kim, Y. S. & Brechbiel, M. W. An overview of targeted alpha therapy. Tumour Biol. 33 , 573–590 (2012).
Parakh, S., Lee, S. T., Gan, H. K. & Scott, A. M. Radiolabeled antibodies for cancer imaging and therapy. Cancers 14 , 1454 (2022).
Witzig, T. E. et al. Randomized controlled trial of yttrium-90-labeled ibritumomab tiuxetan radioimmunotherapy versus rituximab immunotherapy for patients with relapsed or refractory low-grade, follicular, or transformed B-cell non-Hodgkin’s lymphoma. J. Clin. Oncol. 20 , 2453–2463 (2002).
Kaminski, M. S. et al. Pivotal study of iodine I 131 tositumomab for chemotherapy-refractory low-grade or transformed low-grade B-cell non-Hodgkin’s lymphomas. J. Clin. Oncol. 19 , 3918–3928 (2001).
Prasad, V. The withdrawal of drugs for commercial reasons: the incomplete story of tositumomab. JAMA Intern. Med. 174 , 1887–1888 (2014).
Alhaj Moustafa, M. et al. Real world long-term follow-up experience with yttrium-90 ibritumomab tiuxetan in previously untreated patients with low-grade follicular lymphoma and marginal zone lymphoma. Clin. Lymphoma Myeloma Leuk. 22 , 618–625 (2022).
Sgouros, G., Bodei, L., McDevitt, M. R. & Nedrow, J. R. Radiopharmaceutical therapy in cancer: clinical advances and challenges. Nat. Rev. Drug Discov. 19 , 589–608 (2020).
Dowden, H. & Munro, J. Trends in clinical success rates and therapeutic focus. Nat. Rev. Drug Discov. 18 , 495–496 (2019).
Wong, C. H., Siah, K. W. & Lo, A. W. Estimation of clinical trial success rates and related parameters. Biostatistics 20 , 273–286 (2019).
Smietana, K., Siatkowski, M. & Moller, M. Trends in clinical success rates. Nat. Rev. Drug Discov. 15 , 379–380 (2016).
Pham, E. et al. Preclinical assessment of a MUC12-targeted BiTE (bispecific T-cell engager) molecule. Mol. Cancer Ther. 20 , 1977–1987 (2021).
Kebenko, M. et al. A multicenter phase 1 study of solitomab (MT110, AMG 110), a bispecific EpCAM/CD3 T-cell engager (BiTE(R)) antibody construct, in patients with refractory solid tumors. Oncoimmunology 7 , e1450710 (2018).
DeFrancesco, L. Cartography biosciences and nested therapeutics: diamonds in the rough. Nat. Biotechnol. https://doi.org/10.1038/d41587-023-00013-9 (2023).
Mullard, A. Claudin-18.2 attracts the cancer crowd. Nat. Rev. Drug Discov. 22 , 683–686 (2023).
Shah, M. A. et al. Zolbetuximab plus CAPOX in CLDN18.2-positive gastric or gastroesophageal junction adenocarcinoma: the randomized, phase 3 GLOW trial. Nat. Med. 29 , 2133–2141 (2023).
Janne, P. A. et al. Efficacy and safety of patritumab deruxtecan (HER3-DXd) in EGFR inhibitor-resistant, EGFR-mutated non-small cell lung cancer. Cancer Discov. 12 , 74–89 (2022).
Wainberg, Z. A. et al. Bemarituzumab in patients with FGFR2b-selected gastric or gastro-oesophageal junction adenocarcinoma (FIGHT): a randomised, double-blind, placebo-controlled, phase 2 study. Lancet Oncol. 23 , 1430–1440 (2022).
Paz-Ares, L. et al. Tarlatamab, a first-in-class DLL3-targeted bispecific T-cell engager, in recurrent small-cell lung cancer: an open-label, phase I study. J. Clin. Oncol. 41 , 2893–2903 (2023).
Picozzi, V. et al. Gemcitabine/nab-paclitaxel with pamrevlumab: a novel drug combination and trial design for the treatment of locally advanced pancreatic cancer. ESMO Open 5 , e000668 (2020).
Kelly, W. K. et al. Xaluritamig, a STEAP1 x CD3 XmAb 2+1 immune therapy for metastatic castration-resistant prostate cancer: results from dose exploration in a first-in-human study. Cancer Discov. 14 , 76–89 (2024).
Dannenfelser, R. et al. Discriminatory power of combinatorial antigen recognition in cancer T cell therapies. Cell Syst. 11 , 215–228.e5 (2020).
Kloss, C. C., Condomines, M., Cartellieri, M., Bachmann, M. & Sadelain, M. Combinatorial antigen recognition with balanced signaling promotes selective tumor eradication by engineered T cells. Nat. Biotechnol. 31 , 71–75 (2013).
Banaszek, A. et al. On-target restoration of a split T cell-engaging antibody for precision immunotherapy. Nat. Commun. 10 , 5387 (2019).
Oostindie, S. C. et al. Logic-gated antibody pairs that selectively act on cells co-expressing two antigens. Nat. Biotechnol. 40 , 1509–1519 (2022).
Iozzo, R. V. & Schaefer, L. Proteoglycan form and function: a comprehensive nomenclature of proteoglycans. Matrix Biol. 42 , 11–55 (2015).
Ilieva, K. M. et al. Chondroitin sulfate proteoglycan 4 and its potential as an antibody immunotherapy target across different tumor types. Front Immunol. 8 , 1911 (2018).
Filmus, J., Capurro, M. & Rast, J. Glypicans. Genome Biol. 9 , 224 (2008).
Ho, M. & Kim, H. Glypican-3: a new target for cancer immunotherapy. Eur. J. Cancer 47 , 333–338 (2011).
Li, N., Gao, W., Zhang, Y. F. & Ho, M. Glypicans as cancer therapeutic targets. Trends Cancer 4 , 741–754 (2018).
Li, N. et al. The IgG4 hinge with CD28 transmembrane domain improves V(H)H-based CAR T cells targeting a membrane-distal epitope of GPC1 in pancreatic cancer. Nat. Commun. 14 , 1986 (2023).
Kato, D. et al. GPC1 specific CAR-T cells eradicate established solid tumor without adverse effects and synergize with anti-PD-1 Ab. eLife 9 , e49392 (2020).
Li, N., Fu, H., Hewitt, S. M., Dimitrov, D. S. & Ho, M. Therapeutically targeting glypican-2 via single-domain antibody-based chimeric antigen receptors and immunotoxins in neuroblastoma. Proc. Natl Acad. Sci. USA 114 , E6623–E6631 (2017).
Bosse, K. R. et al. Identification of GPC2 as an oncoprotein and candidate immunotherapeutic target in high-risk neuroblastoma. Cancer Cell 32 , 295–309.e12 (2017).
Li, N. et al. CAR T cells targeting tumor-associated exons of glypican 2 regress neuroblastoma in mice. Cell Rep. Med. 2 , 100297 (2021).
Phung, Y., Gao, W., Man, Y.-G., Nagata, S. & Ho, M. High-affinity monoclonal antibodies to cell surface tumor antigen glypican-3 generated through a combination of peptide immunization and flow cytometry screening. mAbs 4 , 592–599 (2012).
Li, D. et al. Persistent polyfunctional chimeric antigen receptor T cells that target glypican 3 eliminate orthotopic hepatocellular carcinomas in mice. Gastroenterology 158 , 2250–2265.e20 (2020).
Nakano, K. et al. Anti-glypican 3 antibodies cause ADCC against human hepatocellular carcinoma cells. Biochem. Biophys. Res. Commun. 378 , 279–284 (2009).
Shi, D. et al. Chimeric antigen receptor-glypican-3 T-cell therapy for advanced hepatocellular carcinoma: results of phase I trials. Clin. Cancer Res. 26 , 3979–3989 (2020).
Loffler, A. et al. A recombinant bispecific single-chain antibody, CD19 x CD3, induces rapid and high lymphoma-directed cytotoxicity by unstimulated T lymphocytes. Blood 95 , 2098–2103 (2000). This paper describes the production of large quantities of a stable bispecific antibody targeting CD19 on B cell lymphomas. The CD19-targeting bispecific antibody was later named blinatumomab and was the first successful bispecific antibody that gained widespread adoption for cancer treatment.
Kantarjian, H. et al. Blinatumomab versus chemotherapy for advanced acute lymphoblastic leukemia. N. Engl. J. Med. 376 , 836–847 (2017). This phase III clinical trial establishes the survival benefit of blinatumomab therapy over chemotherapy in patients with ALL.
Maloney, D. G. et al. Phase I clinical trial using escalating single-dose infusion of chimeric anti-CD20 monoclonal antibody (IDEC-C2B8) in patients with recurrent B-cell lymphoma. Blood 84 , 2457–2466 (1994).
Coiffier, B. et al. CHOP chemotherapy plus rituximab compared with CHOP alone in elderly patients with diffuse large-B-cell lymphoma. N. Engl. J. Med. 346 , 235–242 (2002).
de Weers, M. et al. Daratumumab, a novel therapeutic human CD38 monoclonal antibody, induces killing of multiple myeloma and other hematological tumors. J. Immunol. 186 , 1840–1848 (2011).
Carpenter, R. O. et al. B-cell maturation antigen is a promising target for adoptive T-cell therapy of multiple myeloma. Clin. Cancer Res. 19 , 2048–2060 (2013).
Frerichs, K. A. et al. Preclinical activity of JNJ-7957, a novel BCMAxCD3 bispecific antibody for the treatment of multiple myeloma, is potentiated by daratumumab. Clin. Cancer Res. 26 , 2203–2215 (2020).
Nichakawade, T. D. et al. TRBC1-targeting antibody–drug conjugates for the treatment of T cell cancers. Nature https://doi.org/10.1038/s41586-024-07233-2 (2024).
Paul, S. et al. TCR β chain-directed bispecific antibodies for the treatment of T cell cancers. Sci. Transl. Med . https://doi.org/10.1126/scitranslmed.abd3595 (2021).
Maciocia, P. M. et al. Targeting the T cell receptor β-chain constant region for immunotherapy of T cell malignancies. Nat. Med. 23 , 1416–1423 (2017).
Pearlman, A. H. et al. Targeting public neoantigens for cancer immunotherapy. Nat. Cancer 2 , 487–497 (2021). This review describes the prevalence of targetable public neoantigens in cancer.
Hwang, M. S. et al. Structural engineering of chimeric antigen receptors targeting HLA-restricted neoantigens. Nat. Commun. 12 , 5271 (2021).
Hattori, T. et al. Creating MHC-restricted neoantigens with covalent inhibitors that can be targeted by immune therapy. Cancer Discov. 13 , 132–145 (2023).
Wright, K. M. et al. Hydrophobic interactions dominate the recognition of a KRAS G12V neoantigen. Nat. Commun. 14 , 5063 (2023).
Wu, D., Gallagher, D. T., Gowthaman, R., Pierce, B. G. & Mariuzza, R. A. Structural basis for oligoclonal T cell recognition of a shared p53 cancer neoantigen. Nat. Commun. 11 , 2908 (2020).
Trenevska, I., Li, D. & Banham, A. H. Therapeutic antibodies against intracellular tumor antigens. Front. Immunol. 8 , 1001 (2017).
Hamid, O. et al. 728O results from phase I dose escalation of IMC-F106C, the first PRAME × CD3 ImmTAC bispecific protein in solid tumors. Ann. Oncol. 33 , S875 (2022).
Hofheinz, R. D. et al. Stromal antigen targeting by a humanised monoclonal antibody: an early phase II trial of sibrotuzumab in patients with metastatic colorectal cancer. Onkologie 26 , 44–48 (2003).
Scott, A. M. et al. A phase I dose-escalation study of sibrotuzumab in patients with advanced or metastatic fibroblast activation protein-positive cancer. Clin. Cancer Res. 9 , 1639–1647 (2003).
Benson, A. B. III et al. A phase II randomized, double-blind, placebo-controlled study of simtuzumab or placebo in combination with gemcitabine for the first-line treatment of pancreatic adenocarcinoma. Oncologist 22 , 241-e15 (2017).
Hecht, J. R. et al. A phase II, randomized, double-blind, placebo-controlled study of simtuzumab in combination with FOLFIRI for the second-line treatment of metastatic KRAS mutant colorectal adenocarcinoma. Oncologist 22 , 243-e23 (2017).
Bejarano, L., Jordao, M. J. C. & Joyce, J. A. Therapeutic targeting of the tumor microenvironment. Cancer Discov. 11 , 933–959 (2021).
Gomez-Roca, C. et al. Anti-CSF-1R emactuzumab in combination with anti-PD-L1 atezolizumab in advanced solid tumor patients naive or experienced for immune checkpoint blockade. J. Immunother. Cancer 10 , e004076 (2022).
Ira Seth, W. et al. A phase 1a dose-escalation study of PY159, a monoclonal antibody targeting TREM1 (triggering receptor expressed on myeloid cells 1). J. Clin. Oncol. 41 , 2523 (2023).
Amita P. et al. A phase 1a dose-escalation study of PY314, a TREM2 (triggering receptor expressed on macrophages 2) targeting monoclonal antibody. J. Clin. Oncol . https://doi.org/10.1200/JCO.2022.40.16_suppl.2648 (2022).
Byrne, K. T. & Vonderheide, R. H. CD40 stimulation obviates innate sensors and drives T cell immunity in cancer. Cell Rep. 15 , 2719–2732 (2016).
Byrne, K. T. et al. Neoadjuvant selicrelumab, an agonist CD40 antibody, induces changes in the tumor microenvironment in patients with resectable pancreatic cancer. Clin. Cancer Res. 27 , 4574–4586 (2021).
O’Hara, M. H. et al. CD40 agonistic monoclonal antibody APX005M (sotigalimab) and chemotherapy, with or without nivolumab, for the treatment of metastatic pancreatic adenocarcinoma: an open-label, multicentre, phase 1b study. Lancet Oncol. 22 , 118–131 (2021).
Curigliano, G. et al. Phase I/Ib clinical trial of sabatolimab, an anti-TIM-3 antibody, alone and in combination with spartalizumab, an anti-PD-1 antibody, in advanced solid tumors. Clin. Cancer Res. 27 , 3620–3629 (2021).
Kim, H. R. et al. Cobolimab with dostarlimab and docetaxel in patients with advanced non-small cell lung cancer (NSCLC): COSTAR lung. J. Thorac. Oncol . https://doi.org/10.1016/j.jtho.2022.07.183 (2022).
Spiegel, J. Y. et al. CAR T cells with dual targeting of CD19 and CD22 in adult patients with recurrent or refractory B cell malignancies: a phase 1 trial. Nat. Med. 27 , 1419–1431 (2021).
Bluhm, J. et al. CAR T cells with enhanced sensitivity to B cell maturation antigen for the targeting of B cell non-Hodgkin’s lymphoma and multiple myeloma. Mol. Ther. 26 , 1906–1920 (2018).
Friedman, K. M. et al. Effective targeting of multiple B-cell maturation antigen-expressing hematological malignances by anti-B-cell maturation antigen chimeric antigen receptor T cells. Hum. Gene Ther. 29 , 585–601 (2018).
Golay, J. et al. CD20 levels determine the in vitro susceptibility to rituximab and complement of B-cell chronic lymphocytic leukemia: further regulation by CD55 and CD59. Blood 98 , 3383–3389 (2001).
Teeling, J. L. et al. The biological activity of human CD20 monoclonal antibodies is linked to unique epitopes on CD20. J. Immunol. 177 , 362–371 (2006).
Perez, H. L. et al. Antibody-drug conjugates: current status and future directions. Drug Discov. Today 19 , 869–881 (2014).
Sharma, P. & Kranz, D. M. Recent advances in T-cell engineering for use in immunotherapy. F1000Res https://doi.org/10.12688/f1000research.9073.1 (2016).
Cancer Research Institute. Approval timeline of active immunotherapies. Cancer Research Institute https://www.cancerresearch.org/regulatory-approval-timeline-of-active-immunotherapies (2024).
Johnson, P. C., Gainor, J. F., Sullivan, R. J., Longo, D. L. & Chabner, B. Immune checkpoint inhibitors — the need for innovation. N. Engl. J. Med. 388 , 1529–1532 (2023).
Revisiting checkpoint blockade. Nat. Biotechnol . 40 , 981 (2022).
Postel-Vinay, S. et al. First-in-human phase I study of the OX40 agonist GSK3174998 with or without pembrolizumab in patients with selected advanced solid tumors (ENGAGE-1). J. Immunother. Cancer 11 , e005301 (2023).
Davis, E. J. et al. First-in-human phase I/II, open-label study of the anti-OX40 agonist INCAGN01949 in patients with advanced solid tumors. J. Immunother. Cancer 10 , e004235 (2022).
Andre, P. et al. Anti-NKG2A mAb is a checkpoint inhibitor that promotes anti-tumor immunity by unleashing both T and NK cells. Cell 175 , 1731–1743.e13 (2018).
Qin, S. et al. Novel immune checkpoint targets: moving beyond PD-1 and CTLA-4. Mol. Cancer 18 , 155 (2019).
Tawbi, H. A. et al. Relatlimab and nivolumab versus nivolumab in untreated advanced melanoma. N. Engl. J. Med. 386 , 24–34 (2022).
Gilead. Gilead statement on the discontinuation of magrolimab study in AML with TP53 mutations. Gilead https://www.gilead.com/news-and-press/company-statements/gilead-statement-on-the-discontinuation-of-magrolimab-study-in-aml-with-tp53-mutations (2023).
Gilead. Gilead to discontinue phase 3 ENHANCE study of magrolimab plus azacitidine in higher-risk MDS. Gilead https://www.gilead.com/news-and-press/press-room/press-releases/2023/7/gilead-to-discontinue-phase-3-enhance-study-of-magrolimab-plus-azacitidine-in-higher-risk-mds (2023).
Zhao, B., Zhao, H. & Zhao, J. Efficacy of PD-1/PD-L1 blockade monotherapy in clinical trials. Ther. Adv. Med. Oncol. 12 , 1758835920937612 (2020).
Johnson, D. B., Nebhan, C. A., Moslehi, J. J. & Balko, J. M. Immune-checkpoint inhibitors: long-term implications of toxicity. Nat. Rev. Clin. Oncol. 19 , 254–267 (2022).
Ying Cheng et al. Phase I/II combination study of tifcemalimab with toripalimab in patients with refractory extensive stage small cell lung cancer (ES-SCLC). J. Clin. Oncol. 41 , 8579 (2023).
Shenderov, E. et al. Neoadjuvant enoblituzumab in localized prostate cancer: a single-arm, phase 2 trial. Nat. Med. 29 , 888–897 (2023).
Ansell, S. M. et al. Safety and activity of varlilumab, a novel and first-in-class agonist anti-CD27 antibody, for hematologic malignancies. Blood Adv. 4 , 1917–1926 (2020).
Claus, C., Ferrara-Koller, C. & Klein, C. The emerging landscape of novel 4-1BB (CD137) agonistic drugs for cancer immunotherapy. MAbs 15 , 2167189 (2023).
Elizabeth Budde, M. et al. Preliminary results of a phase 1 dose escalation study of the first-in-class IgM based bispecific antibody Igm-2323 (anti-CD20 x anti-CD3) in patients with advanced B-cell malignancies. Blood 136 , 45–46 (2020).
Wang, B. T. et al. Multimeric anti-DR5 IgM agonist antibody IGM-8444 is a potent inducer of cancer cell apoptosis and synergizes with chemotherapy and BCL-2 inhibitor ABT-199. Mol. Cancer Ther. 20 , 2483–2494 (2021).
Li, K., Yun, R., Chai, M., Yakkundi, P. & Rosete, R. Novel CD38xCD3 bispecific IgM T cell engager, IGM-2644, potently kills multiple myeloma cells though complement and T cell dependent mechanisms. Cancer Res. 83 , 2959 (2023).
Wang, B. et al. Anti-DR5 agonist IgM antibody IGM-8444 combined with SMAC mimetic birinapant induces strong synergistic tumor cytotoxicity. Cancer Res. 82 , 1068 (2022).
Klein, C., Brinkmann, U., Reichert, J. M. & Kontermann, R. E. The present and future of bispecific antibodies for cancer therapy. Nat. Rev. Drug Discov. https://doi.org/10.1038/s41573-024-00896-6 (2024). This review focuses on the current state and future directions of bispecific antibodies in oncology.
Dickinson, M. J. et al. Glofitamab for relapsed or refractory diffuse large B-cell lymphoma. N. Engl. J. Med. 387 , 2220–2231 (2022).
Bacac, M. et al. CD20-TCB with obinutuzumab pretreatment as next-generation treatment of hematologic malignancies. Clin. Cancer Res. 24 , 4785–4797 (2018).
Nolan-Stevaux, O. et al. AMG 509 (xaluritamig), an anti-STEAP1 XmAb 2+1 T-cell redirecting immune therapy with avidity-dependent activity against prostate cancer. Cancer Discov. 14 , 90–103 (2024).
Li, G. et al. Novel CD123xCD3 bispecific IgM antibody, IGM-2537, potently induces T-cell mediated cytotoxicity of acute myeloid leukemia cells with minimal cytokine release. Cancer Res. 83 , 2933 (2023).
Genevive, H. H. et al. Pharmacodynamics and biomarker correlates of imvotamab (IGM-2323), the first-in-class CD20xCD3 bispecific IgM antibody with dual mechanisms of action, in patients with advanced B cell malignancies. Blood 140 , 6436–6438 (2022).
Luke, J. J. et al. The PD-1- and LAG-3-targeting bispecific molecule tebotelimab in solid tumors and hematologic cancers: a phase 1 trial. Nat. Med. 29 , 2814–2824 (2023).
Dovedi, S. J. et al. Design and efficacy of a monovalent bispecific PD-1/CTLA4 antibody that enhances CTLA4 blockade on PD-1 + activated T cells. Cancer Discov. 11 , 1100–1117 (2021).
Gao, X. et al. Safety and antitumour activity of cadonilimab, an anti-PD-1/CTLA-4 bispecific antibody, for patients with advanced solid tumours (COMPASSION-03): a multicentre, open-label, phase 1b/2 trial. Lancet Oncol. 24 , 1134–1146 (2023).
Chen, B. et al. A phase Ib/II study of cadonilimab (PD-1/CTLA-4 bispecific antibody) plus anlotinib as first-line treatment in patients with advanced non-small cell lung cancer. Br. J. Cancer 130 , 450–456 (2024).
Li, Q. et al. The anti-PD-L1/CTLA-4 bispecific antibody KN046 in combination with nab-paclitaxel in first-line treatment of metastatic triple-negative breast cancer: a multicenter phase II trial. Nat. Commun. 15 , 1015 (2024).
Kvarnhammar, A. M. et al. The CTLA-4 x OX40 bispecific antibody ATOR-1015 induces anti-tumor effects through tumor-directed immune activation. J. Immunother. Cancer 7 , 103 (2019).
Karin Lee et al. Preclinical studies support clinical development of AZD2936, a monovalent bispecific humanized antibody targeting PD-1 and TIGIT. J. Immunother. Cancer 10 , A489 (2023).
Google Scholar
Shapir Itai, Y. et al. Bispecific dendritic-T cell engager potentiates anti-tumor immunity. Cell 187 , 375–389.e18 (2024).
Zhao, L. et al. A novel CD19/CD22/CD3 trispecific antibody enhances therapeutic efficacy and overcomes immune escape against B-ALL. Blood 140 , 1790–1802 (2022).
Wu, L. et al. Trispecific antibodies enhance the therapeutic efficacy of tumor-directed T cells through T cell receptor co-stimulation. Nat. Cancer 1 , 86–98 (2020).
Pardon, E. et al. A general protocol for the generation of nanobodies for structural biology. Nat. Protoc. 9 , 674–693 (2014).
Li, D. et al. Camel nanobody-based B7-H3 CAR-T cells show high efficacy against large solid tumours. Nat. Commun. 14 , 5920 (2023).
Xu, J. et al. Nanobodies from camelid mice and llamas neutralize SARS-CoV-2 variants. Nature 595 , 278–282 (2021). This paper describes the generation of a transgenic mouse (nanomouse), which expresses camelid variable heavy domain of heavy chain (VHH) genes. Such transgenic mouse platforms enable the production of nanobodies from mice.
Hu, Y. et al. RenNano® mice: a heavy-chain-only antibody platform for the generation of nanobody therapeutics. Cancer Res. 83 , LB210 (2023).
De Genst, E. et al. Molecular basis for the preferential cleft recognition by dromedary heavy-chain antibodies. Proc. Natl Acad. Sci. USA 103 , 4586–4591 (2006).
Berdeja, J. G. et al. Ciltacabtagene autoleucel, a B-cell maturation antigen-directed chimeric antigen receptor T-cell therapy in patients with relapsed or refractory multiple myeloma (CARTITUDE-1): a phase 1b/2 open-label study. Lancet 398 , 314–324 (2021).
Munshi, N. C. et al. Idecabtagene vicleucel in relapsed and refractory multiple myeloma. N. Engl. J. Med. 384 , 705–716 (2021).
Jovcevska, I. & Muyldermans, S. The therapeutic potential of nanobodies. BioDrugs 34 , 11–26 (2020).
Lucchi, R., Bentanachs, J. & Oller-Salvia, B. The masking game: design of activatable antibodies and mimetics for selective therapeutics and cell control. ACS Cent. Sci. 7 , 724–738 (2021). This review describes the recent advancements in activatable antibodies in cancer and other diseases.
Sulea, T. et al. Structure-based engineering of pH-dependent antibody binding for selective targeting of solid-tumor microenvironment. MAbs 12 , 1682866 (2020).
Kang, J. C. et al. Engineering a HER2-specific antibody-drug conjugate to increase lysosomal delivery and therapeutic efficacy. Nat. Biotechnol. 37 , 523–526 (2019).
Zhang, Y. et al. Hijacking antibody-induced CTLA-4 lysosomal degradation for safer and more effective cancer immunotherapy. Cell Res. 29 , 609–627 (2019).
Desnoyers, L. R. et al. Tumor-specific activation of an EGFR-targeting probody enhances therapeutic index. Sci. Transl. Med. 5 , 207ra144 (2013).
Autio, K. A., Boni, V., Humphrey, R. W. & Naing, A. Probody therapeutics: an emerging class of therapies designed to enhance on-target effects with reduced off-tumor toxicity for use in immuno-oncology. Clin. Cancer Res. 26 , 984–989 (2020).
Boni, V. et al. Praluzatamab ravtansine, a CD166-targeting antibody-drug conjugate, in patients with advanced solid tumors: an open-label phase I/II Trial. Clin. Cancer Res. 28 , 2020–2029 (2022).
Boustany, L. M. et al. A probody T cell-engaging bispecific antibody targeting EGFR and CD3 inhibits colon cancer growth with limited toxicity. Cancer Res. 82 , 4288–4298 (2022).
Lajoie, M. J. et al. Designed protein logic to target cells with precise combinations of surface antigens. Science 369 , 1637–1643 (2020).
Xu, S. Internalization, trafficking, intracellular processing and actions of antibody-drug conjugates. Pharm. Res. 32 , 3577–3583 (2015).
Saunders, K. O. Conceptual approaches to modulating antibody effector functions and circulation half-life. Front. Immunol. 10 , 1296 (2019).
Brandl, F., Busslinger, S., Zangemeister-Wittke, U. & Pluckthun, A. Optimizing the anti-tumor efficacy of protein-drug conjugates by engineering the molecular size and half-life. J. Control. Release 327 , 186–197 (2020).
Bardia, A. et al. Sacituzumab govitecan in metastatic triple-negative breast cancer. N. Engl. J. Med. 384 , 1529–1541 (2021).
Senior, M. Cancer-targeting antibody-drug conjugates drive dealmaking frenzy. Nat. Biotechnol. 42 , 362–366 (2024).
Weng, W. et al. Antibody-exatecan conjugates with a novel self-immolative moiety overcome resistance in colon and lung cancer. Cancer Discov. 13 , 950–973 (2023).
Li, B. T. et al. Trastuzumab deruxtecan in HER2-mutant non-small-cell lung cancer. N. Engl. J. Med. 386 , 241–251 (2022).
Siena, S. et al. Trastuzumab deruxtecan (DS-8201) in patients with HER2-expressing metastatic colorectal cancer (DESTINY-CRC01): a multicentre, open-label, phase 2 trial. Lancet Oncol. 22 , 779–789 (2021).
Esfandiari, A., Cassidy, S. & Webster, R. M. Bispecific antibodies in oncology. Nat. Rev. Drug Discov. 21 , 411–412 (2022).
do Pazo, C., Nawaz, K. & Webster, R. M. The oncology market for antibody-drug conjugates. Nat. Rev. Drug Discov. 20 , 583–584 (2021).
Criscitiello, C., Morganti, S. & Curigliano, G. Antibody-drug conjugates in solid tumors: a look into novel targets. J. Hematol. Oncol. 14 , 20 (2021).
Michael, L. W. et al. Zilovertamab vedotin targeting of ROR1 as therapy for lymphoid cancers. NEJM Evid. https://doi.org/10.1056/EVIDoa2100001 (2021).
Saura Manich, C. et al. LBA15 — primary outcome of the phase III SYD985.002/TULIP trial comparing [vic-]trastuzumab duocarmazine to physician’s choice treatment in patients with pre-treated HER2-positive locally advanced or metastatic breast cancer. Ann. Oncol. 32 , S1283–S1346 (2021).
Powles, T. B. et al. LBA6 EV-302/KEYNOTE-A39: Open-label, randomized phase III study of enfortumab vedotin in combination with pembrolizumab (EV+P) vs chemotherapy (Chemo) in previously untreated locally advanced metastatic urothelial carcinoma (la/mUC). Ann. Oncol. 34 , S1340 (2023).
Antignani, A. et al. Targeting receptors on cancer cells with protein toxins. Biomolecules https://doi.org/10.3390/biom10091331 (2020).
Pemmaraju, N. et al. Tagraxofusp in blastic plasmacytoid dendritic-cell neoplasm. N. Engl. J. Med. 380 , 1628–1637 (2019).
Saleh, M. N. et al. Antitumor activity of DAB389IL-2 fusion toxin in mycosis fungoides. J. Am. Acad. Dermatol. 39 , 63–73 (1998).
Sartor, O. et al. Lutetium-177-PSMA-617 for metastatic castration-resistant prostate cancer. N. Engl. J. Med. 385 , 1091–1103 (2021).
Strosberg, J. et al. Phase 3 trial of (177)Lu-dotatate for midgut neuroendocrine tumors. N. Engl. J. Med. 376 , 125–135 (2017).
Michael, S. H. et al. First-in-human safety, imaging and dosimetry of [68Ga]Ga-DPI-4452, a novel CA IX-targeting peptide, in patients with clear cell renal cell carcinoma. J. Clin. Oncol . https://doi.org/10.1200/JCO.2024.42.4_suppl.37 (2024).
Dolgin, E. Radioactive drugs emerge from the shadows to storm the market. Nat. Biotechnol. 36 , 1125–1127 (2018).
Sathekge, M. M. et al. Actinium-225-PSMA radioligand therapy of metastatic castration-resistant prostate cancer (WARMTH Act): a multicentre, retrospective study. Lancet Oncol. 25 , 175–183 (2024).
Slamon, D. J. et al. Human breast cancer: correlation of relapse and survival with amplification of the HER-2/neu oncogene. Science 235 , 177–182 (1987).
Rubin, I. & Yarden, Y. The basic biology of HER2. Ann. Oncol. 12 , S3-8 (2001).
Verma, S. et al. Trastuzumab emtansine for HER2-positive advanced breast cancer. N. Engl. J. Med. 367 , 1783–1791 (2012).
Morgan, R. A. et al. Case report of a serious adverse event following the administration of T cells transduced with a chimeric antigen receptor recognizing ERBB2. Mol. Ther. 18 , 843–851 (2010).
Saunders, L. R. et al. A DLL3-targeted antibody-drug conjugate eradicates high-grade pulmonary neuroendocrine tumor-initiating cells in vivo. Sci. Transl. Med. 7 , 302ra136 (2015).
Vos, J. L. et al. Neoadjuvant immunotherapy with nivolumab and ipilimumab induces major pathological responses in patients with head and neck squamous cell carcinoma. Nat. Commun. 12 , 7348 (2021).
Download references
Acknowledgements
S.P. M.F.K., C.B., N.P. and S.Z. were supported by The Virginia and D.K. Ludwig Fund for Cancer Research, the Lustgarten Foundation for Pancreatic Cancer Research, The Commonwealth Fund, the Bloomberg~Kimmel Institute for Cancer Immunotherapy, and the National Institutes of Health (NIH) Cancer Center Support grant P30 CA006973. S.P. was supported by NCI grant K08CA270403, the Leukaemia Lymphoma Society Translation Research Program award, the American Society of Hematology Scholar award, and the Swim Across America Translational Cancer Research Award. M.F.K. was supported by NIH/NIAID grant 1R21AI176764-01, the Jerome Greene Foundation, the Cupid Foundation, the Lupus Research Alliance Lupus Innovation Award, the Rheumatology Research Foundation Investigator Award, the Harrington Discovery Institute Harrington Scholar-Innovator Award, the Sol Goldman MS Research Program, and the Bisciotti Foundation Translational Fund. C.B. was supported by NCI grant R37 CA230400. M.H. was supported by the Intramural Research Program of NIH, NCI and Center for Cancer Research (Z01 BC010891, ZIA BC010891 and ZIC BC 011891).
Author information
Authors and affiliations.
Department of Oncology, Johns Hopkins School of Medicine, Baltimore, MD, USA
Suman Paul, Drew M. Pardoll, Nickolas Papadopoulos & Shibin Zhou
Division of Rheumatology, Department of Medicine, The Johns Hopkins University School of Medicine, Baltimore, MD, USA
Maximilian F. Konig
Department of Neurosurgery, The Johns Hopkins University School of Medicine, Baltimore, MD, USA
Chetan Bettegowda
Discovery Chemistry, Merck Research Laboratory, Merck and Co, West Point, PA, USA
Katharine M. Wright & Sandra B. Gabelli
Antibody Engineering Program, Laboratory of Molecular Biology, Center for Cancer Research, National Cancer Institute, Bethesda, MD, USA
Mitchell Ho
Third Rock Ventures, San Francisco, CA, USA
Andrea van Elsas
You can also search for this author in PubMed Google Scholar
Contributions
S.P., K.M.W., S.B.G. and M.H. researched data for the article. S.P., S.B.G., M.H. and A.vE. contributed substantially to discussion of the content. S.P., K.M.W., S.B.G., M.H., A.vE. and S.Z. wrote the article. All authors reviewed and/or edited the manuscript before submission.
Corresponding authors
Correspondence to Suman Paul , Sandra B. Gabelli , Mitchell Ho or Andrea van Elsas .
Ethics declarations
Competing interests.
The Johns Hopkins University has filed patent applications related to technologies described in this paper on which S.P., M.F.K., N.P., D.M.P. and S.Z. are listed as inventors. M.H. is an inventor on NIH patents in antibody and cell therapies and may receive blinded royalties from the NIH. N.P. is a founder of Thrive Earlier Detection, an Exact Sciences company. N.P. is a consultant to Thrive Earlier Detection. N.P. and S.Z. hold equity in Exact Sciences. N.P. and S.Z. are founders of and/or consultants to and own equity in ManaT Bio., Neophore and Personal Genome Diagnostics. N.P. holds equity in Haystack Oncology and CAGE Pharma. N.P. is a consultant to Vidium. S.P. owns equity in Gilead, is a consultant to Merck and received payment from IQVIA. M.F.K. is a consultant to Argenx, Atara Biotherapeutics, Revel Pharmaceuticals, Sana Biotechnology and Sanofi. S.Z. has a research agreement with BioMed Valley Discoveries, Inc. C.B. is a consultant for Depuy-Synthes, Bionaut Labs, Galectin Therapeutics, Haystack Oncology and Privo Technologies. C.B. is a co-founder of OrisDx and Belay Diagnostics. D.M.P. reports grant and patent royalties through institution from BMS, a grant from Compugen, stock from Trieza Therapeutics and Dracen Pharmaceuticals, and founder equity from Potenza; is a founder of and consultant to and owns equity in ManaT Bio; is a consultant for Aduro Biotech, Amgen, Astra Zeneca (Medimmune/Amplimmune), Bayer, DNAtrix, Dynavax Technologies Corporation, Ervaxx, FLX Bio, Rock Springs Capital, Janssen, Merck, Tizona and Immunomic-Therapeutics; is on the scientific advisory board of Five Prime Therapeutics, Camden Nexus II, WindMil; and is on the board of directors for Dracen Pharmaceuticals. K.M.W. and S.B.G. are employees of Merck & Co., Inc. at the time of submission and may have stock ownership in Merck & Co., Inc., Rahway, NJ, USA.
Peer review
Peer review information.
Nature Reviews Cancer thanks Falk Nimmerjahn and the other, anonymous, reviewer(s) for their contribution to the peer review of this work.
Additional information
Publisher’s note Springer Nature remains neutral with regard to jurisdictional claims in published maps and institutional affiliations.
Related links
American Cancer Society: https://www.cancer.org/research/cancer-facts-statistics/all-cancer-facts-figures/2023-cancer-facts-figures.html
Antibody Society: https://www.antibodysociety.org/resources/approved-antibodies/
Supplementary information
Supplementary information.
A severe and potentially life-threatening reaction owing to exposure to an allergen such as an antibody or other medication. Common symptoms of anaphylaxis include swelling of the face and throat, difficulty in breathing, an increase in heart rate, a drop in blood pressure and loss of consciousness.
(ADCC). A mechanism through which antibodies bind to target cells followed by recruitment of immune cells such as NK cells and macrophages to kill the target cells. The immune cells secrete cytotoxic granules (perforins and granzymes), and induce FAS signalling leading to target cell death.
(ADCP). A mechanism through which antibodies bind to target cells, which in turn stimulates immune cells such as macrophages to engulf and degrade the target cells.
Isolation and expansion of single B cells that produce the desired monoclonal antibodies, to obtain the antibody-coding sequence.
With antibody–drug conjugates (ADCs), refers to a phenomenon wherein neighbouring cells near the target cancer cell are killed by the released cytotoxic payload. This effect can enhance the overall potency of the ADC by causing a broader destruction of cancer cells beyond the primary target cell.
Condition characterized by the leakage of fluid from small blood vessels (capillaries) into surrounding tissues. This leakage leads to a decrease in blood volume and can result in low blood pressure along with oedema (swelling) in various parts of the body, including the lungs, and organ failure.
(CDRs). Specific regions within the antibody heavy and light chain variable domains that bind to the target antigen.
(CDC). A mechanism through which antibodies bind to the target cell followed by activation of the complement system, leading to lysis of the target cells.
(CRS). Systemic inflammation characterized by a constellation of symptoms such as fever, hypotension and hypoxia and mediated by the release of multiple cytokines from the immune cells of patients. CRS is a typical adverse effect observed with the use of T cell engager (TCE) bispecific antibodies.
A reduction in the number of circulating blood cells, such as red blood cells (erythrocytes), white blood cells (leukocytes) and/or platelets (thrombocytes). Cytopenias can be caused by several factors including exposure to drugs or antibodies that hinder the growth of new cells.
(DAR). The number of drugs attached to each antibody in an ADC.
(Fab). An antibody which consists of two identical Fab fragments and one Fc fragment. Each Fab fragment is responsible for binding to a specific antigen. The Fab fragment is obtained by cleaving the antibody at specific sites using enzymes, such as papain or pepsin.
(Fc). Fc fragment interacts with various immune cells through Fc receptors and with complement proteins that contribute to the immune response generated by the antibody. Each antibody class and subclass has unique Fc regions. Understanding the Fc fragment is crucial in the design of therapeutic antibodies because modifications to this region can impact the pharmacokinetics, effector functions and therapeutic efficacy of an antibody.
A rare but serious condition that is characterized by the combination of haemolytic anaemia (destruction of red blood cells), thrombocytopenia (low platelet count) and acute kidney injury. It can be mediated by bacterial infections (such as Escherischia coli ) or exposure to drugs and antibodies.
Refers to the property of being repelled by water. Hydrophobic substances are insoluble or poorly soluble in water. The hydrophobicity of the payload can affect the overall stability of the ADC. Highly hydrophobic payloads may lead to aggregation or destabilization of the ADC structure, potentially impacting its efficacy and safety.
(MSI-H). Cells with mismatch-repair deficiency resulting in high mutation burden and altered microsatellite (tract of repetitive DNA) sequences. MSI-H cancers are associated with a higher response to immune checkpoint-inhibiting antibodies.
Identical antibodies that bind to a specific part of the target antigen (epitope) and are derived from single clones of immune cells (such as B cells, plasma cells or hybridoma cells).
A group of disorders characterized by abnormal production and maturation of blood cells in the bone marrow. In myelodysplastic syndromes, the bone marrow fails to produce enough healthy blood cells, leading to low levels of red blood cells (anaemia), white blood cells (leukopenia) and platelets (thrombocytopenia).
(FcRn). A receptor expressed by vascular endothelial cells and immune cells, which binds to the Fc portion of IgG antibodies. IgG antibody binding to FcRn leads to receptor-mediated internalization and recycling of the IgG, which is responsible for the long IgG half-life (about 21 days) in circulation.
A potential side effect that can occur owing to the cytotoxic payload component of the ADC affecting the peripheral nerves. Peripheral neuropathy caused by ADCs can manifest as numbness, tingling, burning sensations or pain in the hands, feet or other extremities.
A public neoantigen is derived from a mutated protein and is found in multiple individuals with the same type of cancer. This shared characteristic makes public neoantigens particularly important in cancer immunotherapy because therapies targeting these common neoantigens can benefit a broad patient population. Common public neoantigens include BRAF V600E , KRAS G12D , KRAS G12C and TP53 R175H . By contrast, private neoantigens are unique to an individual patient with cancer. Targeting private neoantigens requires the development of personalized therapies such as custom cancer vaccines and T cell-based therapies.
(scFv). An engineered antibody fragment composed of variable regions of the heavy and light chains combined into a single peptide chain by a linker. The scFv retains the ability to bind specifically to a target antigen, similar to a full-size antibody. The advantages of scFv include its smaller size (~25 kDa), which facilitates easier production and manipulation.
Also known as nanobodies, are antibodies derived from camelids that consist of only a variable heavy domain and as a result have a relatively low molecular weight (~15 kDa), hence the name nanobody. By contrast, human antibodies consist of variable heavy and light domains and have higher molecular weights (a full-length IgG antibody is ~150 kDa and an scFv is ~ 25 kDa).
Enzymes that maintain proper function and stability of DNA by cleaving DNA to relieve torsional strain and supercoiling occurring owing to processes such as DNA replication. Topoisomerase inhibitors disrupt this ability to maintain DNA and cause cell death.
Proteins and other antigenic molecules expressed on the surface of tumour cells that can be targeted by therapeutic antibodies.
Rights and permissions
Springer Nature or its licensor (e.g. a society or other partner) holds exclusive rights to this article under a publishing agreement with the author(s) or other rightsholder(s); author self-archiving of the accepted manuscript version of this article is solely governed by the terms of such publishing agreement and applicable law.
Reprints and permissions
About this article
Cite this article.
Paul, S., Konig, M.F., Pardoll, D.M. et al. Cancer therapy with antibodies. Nat Rev Cancer (2024). https://doi.org/10.1038/s41568-024-00690-x
Download citation
Accepted : 29 March 2024
Published : 13 May 2024
DOI : https://doi.org/10.1038/s41568-024-00690-x
Share this article
Anyone you share the following link with will be able to read this content:
Sorry, a shareable link is not currently available for this article.
Provided by the Springer Nature SharedIt content-sharing initiative
Quick links
- Explore articles by subject
- Guide to authors
- Editorial policies
Sign up for the Nature Briefing: Cancer newsletter — what matters in cancer research, free to your inbox weekly.

Doctor Says He’s Cancer-Free 1 Year After Using His Own Experimental Therapy on Brain Tumor
Professor Richard Scolyer's incurable stage 4 glioblastoma diagnosis was revealed in June 2023
An Australian doctor remains brain cancer-free a year after undergoing a world-first treatment based on his own research.
Professor Richard Scolyer, 57, used his own pioneering studies on melanoma to treat his incurable stage 4 glioblastoma following his diagnosis in June 2023 .
On Monday, May 13, he shared an update about the experimental treatment on X (formerly known as Twitter) alongside two images from a recent MRI scan.
"I had brain #MRI scan last Thursday looking for recurrent #glioblastoma (&/or treatment complications). I found out yesterday that there is still no sign of recurrence. I couldn’t be happier!!!!!," wrote Scolyer.
"Thank you to the fabulous team looking after me so well especially my wife Katie & wonderful family!" he added.
Scolyer's diagnosis was revealed in June 2023 after he was taken ill while traveling for work in Poland. He then became the first brain cancer patient to undergo pre-surgery combination immunotherapy.
Per the Cancer Research Institute , cancer immunotherapy "is a form of cancer treatment that uses the power of the body’s own immune system to prevent, control, and eliminate cancer."
The pathologist — who was named the 2024 Australian of the Year alongside his Melanoma Institute Australia co-medical director Georgina Long over their life-saving research — told the BBC , "To be honest, I was more nervous than I have been for any previous scan."
"I'm just thrilled and delighted... couldn't be happier," he added to the outlet.
"By undertaking an experimental treatment with risk of shortening his life, he has advanced the understanding of brain cancer and is benefiting future patients," the University of Sydney said as it named Scolyer and Long the Australians of the Year, per Sky News .
After having a difficult couple of months of treatment toward the start of the year, suffering from epileptic seizures, liver issues and pneumonia, Scolyer is now feeling "the best" he's "felt for yonks," he told the BBC.
The doctor is back to exercising daily, and regularly does 15km runs. "It certainly doesn't mean that my brain cancer is cured... but it's just nice to know that it hasn't come back yet, so I've still got some more time to enjoy my life with my wife Katie and my three wonderful kids," he told the outlet.
Never miss a story — sign up for PEOPLE's free daily newsletter to stay up-to-date on the best of what PEOPLE has to offer, from celebrity news to compelling human interest stories.
His research partner Long insisted they're "not there yet" when it comes to developing an approved and regulated course of treatment. "We've generated a whole heap of data, to then make a foundation for that next step, so that we can help more people," she said, per the BBC.
"What we have to really focus on is showing that this pre-surgery, combination immunotherapy type of approach works in a large number of people," Long added.
The Melanoma Institute Australia didn't immediately respond to PEOPLE when approached for comment.
For more People news, make sure to sign up for our newsletter!
Read the original article on People .
Merck stops testing combo drug for skin cancer as more patients discontinue
- Medium Text
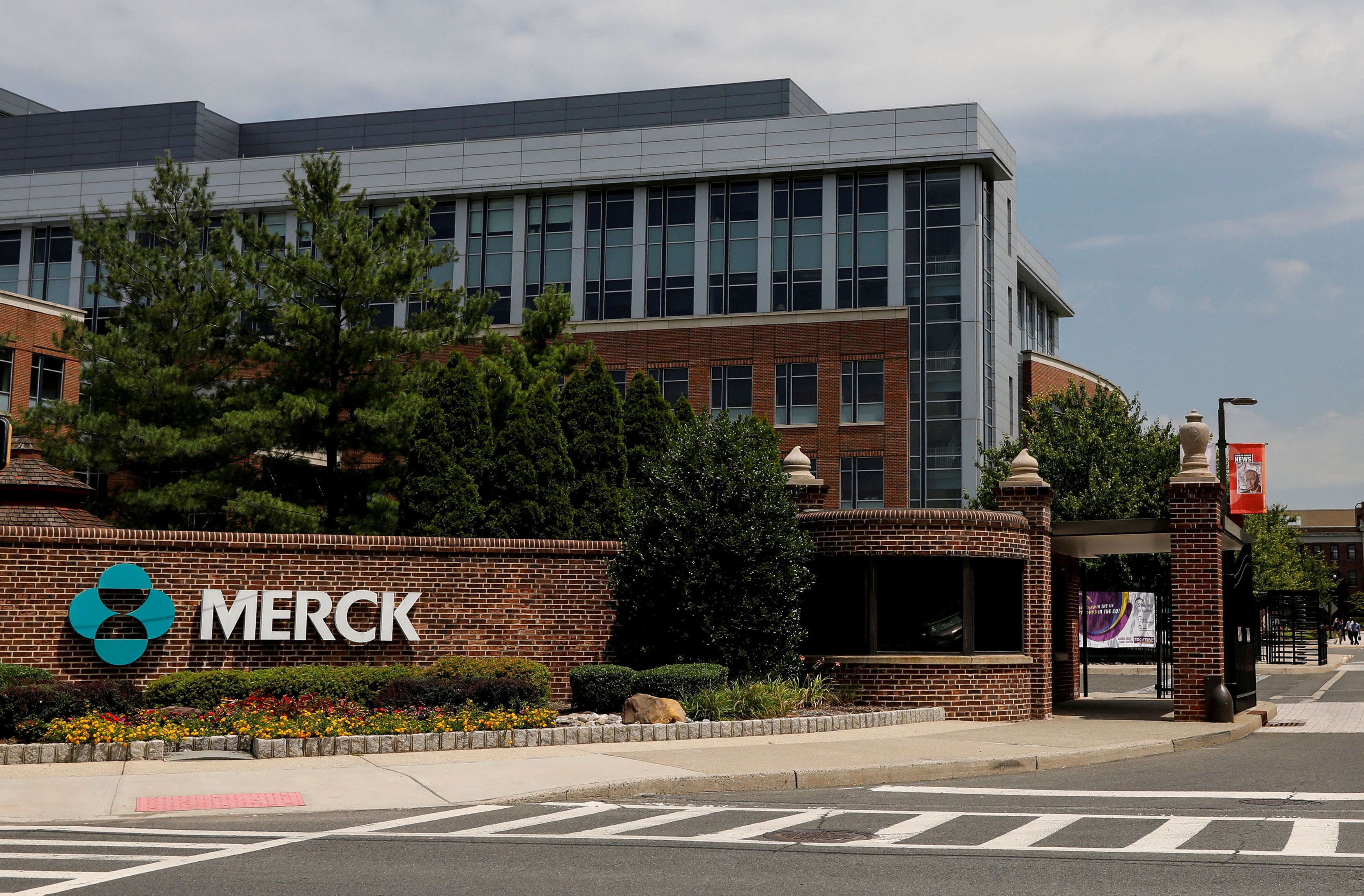
- Company Merck & Co Inc Follow
- Company Arcus Biosciences Inc Follow
- Company Gilead Sciences Inc Follow
Sign up here.
Reporting by Mariam Sunny and Leroy Leo in Bengaluru; Editing by Tasim Zahid and Sriraj Kalluvila
Our Standards: The Thomson Reuters Trust Principles. New Tab , opens new tab
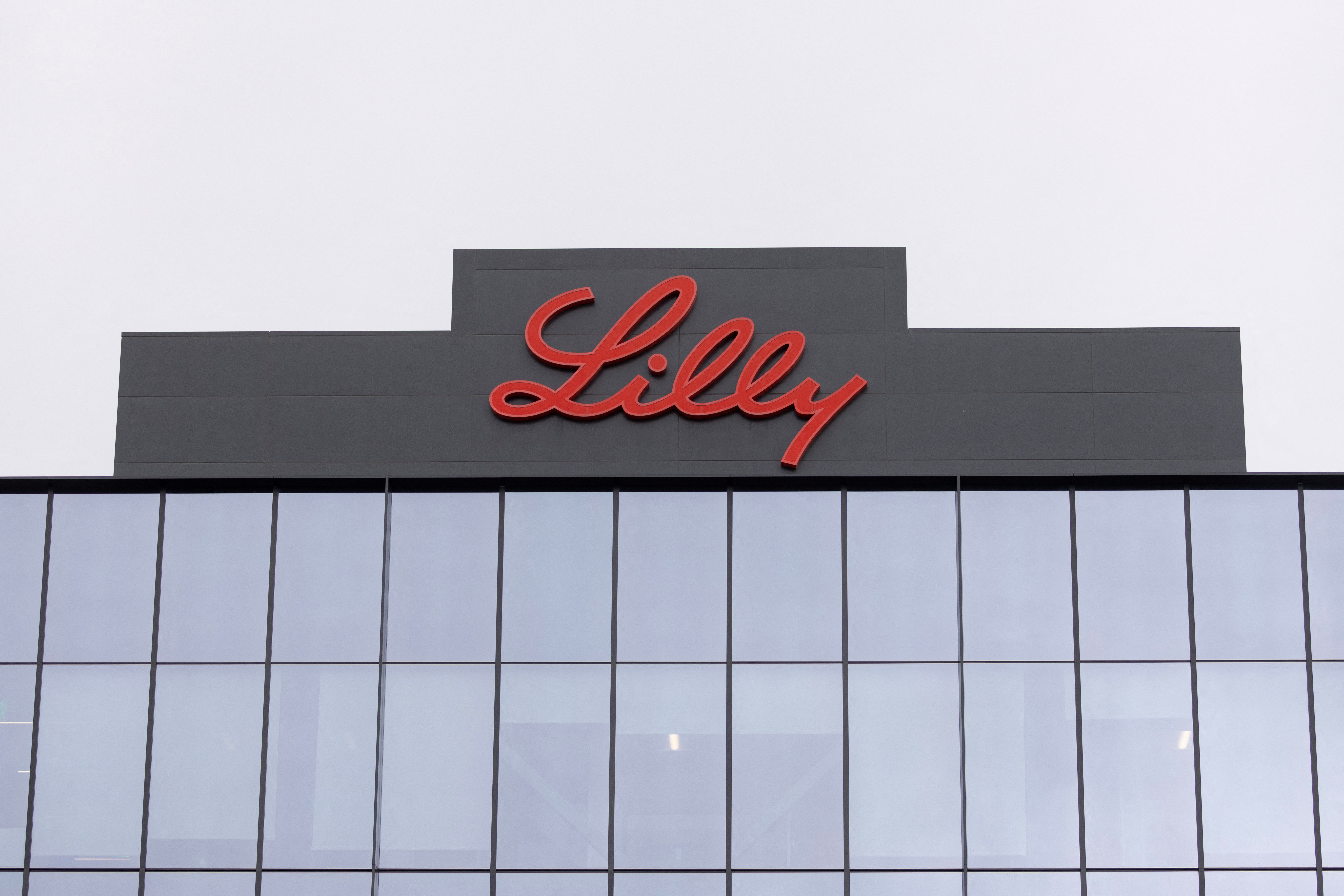
Business Chevron
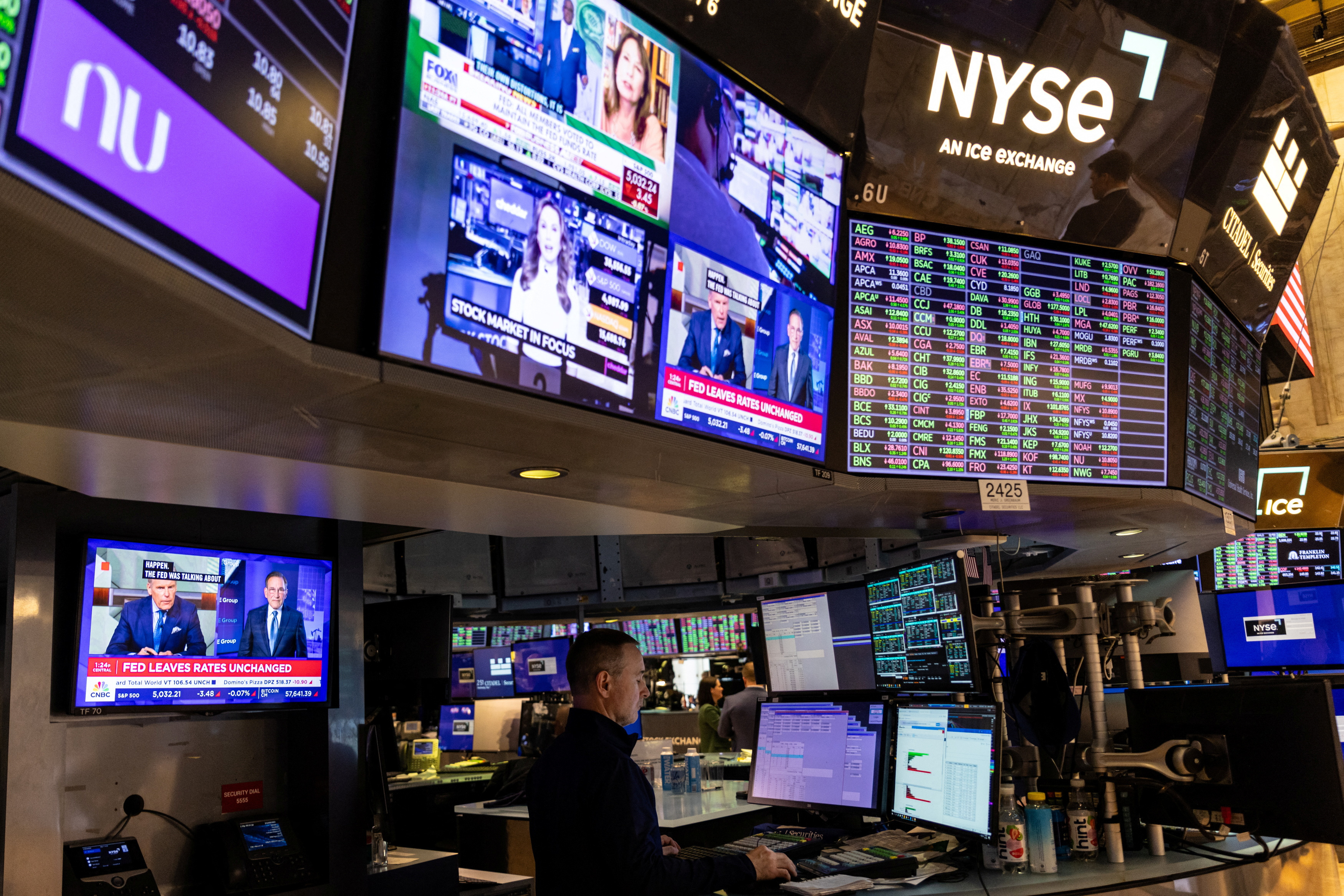
VIEW Dow hits 40,000 on earnings boost, rate cut bets
The Dow Jones Industrial Average surpassed 40,000 points for the first time on Thursday, the blue-chip index's fastest 10,000 point-climb, powered by strong quarterly results and rising bets of interest rate cuts by the Federal Reserve.
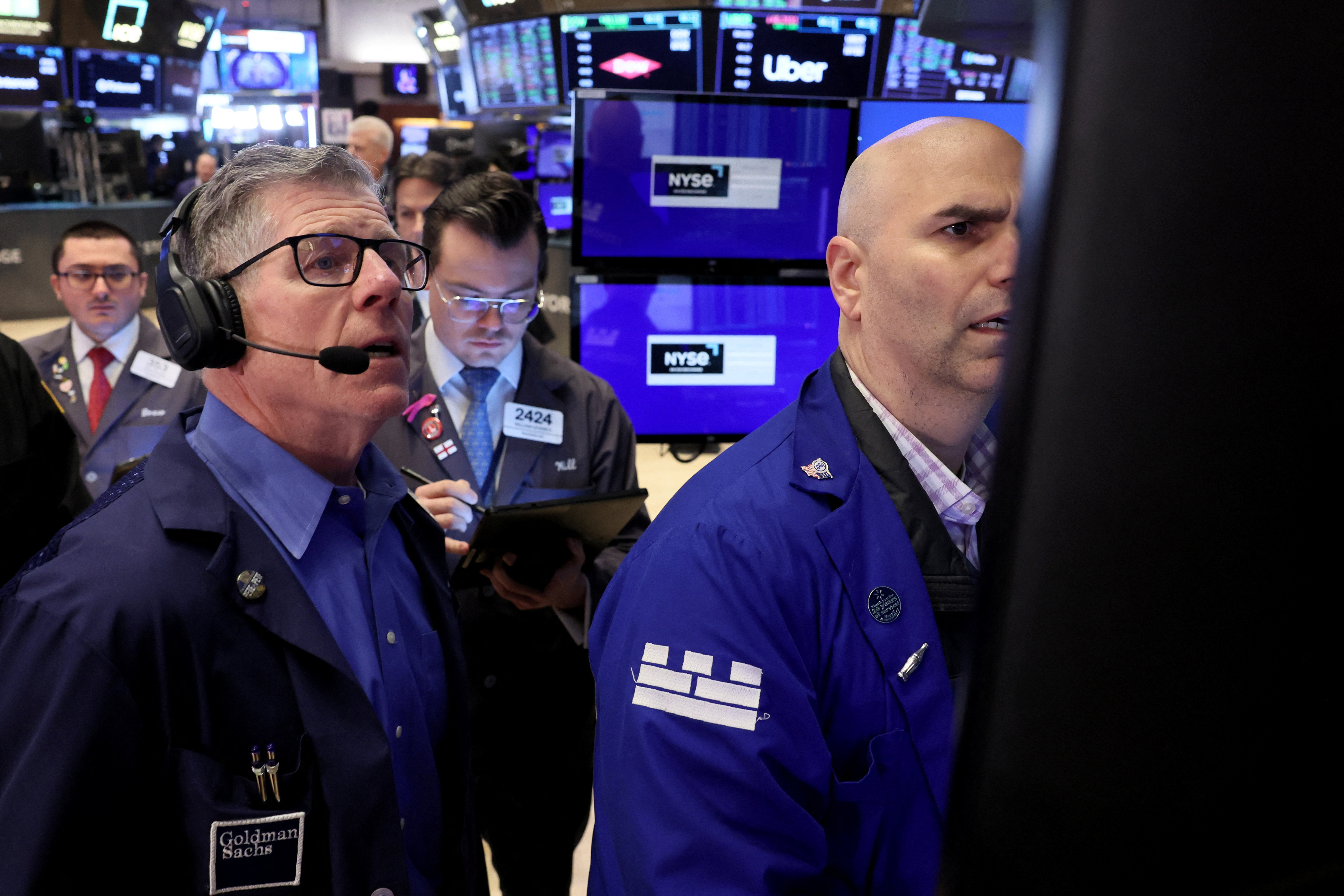
BREAKING: Dow hits 40,000: The signature stock index's new high comes amid renewed hopes for the U.S. economy
Olivia Munn had hysterectomy amid cancer treatment, froze her eggs in hope of more kids
Olivia Munn has revealed she underwent a hysterectomy, opening up about her jo urney with breast cancer in an interview with Vogue published Sunday on Mother’s Day.
Munn, 43, had revealed in March that she was diagnosed with luminal B breast cancer in both breasts and underwent a double mastectomy.
Since then, she has completed a round of egg retrieval with the hope to one day continue expanding her family with her partner, comedian John Mulaney, 41.
Munn also revealed in the Vogue interview that she underwent a hysterectomy last month.
It wasn't an easy decision for Munn, the "Newsroom" actor, but one she made for her 2-year-old son, Malcolm.
“It was a big decision to make, but it was the best decision for me because I needed to be present for my family," she said. "I had friends try to cheer me up by saying, ‘Malcolm’s not going to remember this. Don’t worry.’
"But I just kept thinking to myself, ‘I’m going to remember this, that I missed all these things,’” she continued. “It’s his childhood, but it’s my motherhood, and I don’t want to miss any of these parts if I don’t have to.”
Munn's cancer journey had a surprising start. She took a genetic test that returned negative for the breast cancer gene in April 2023 — as well as clear mammograms and an ultrasound. However, her OB-GYN calculated her breast cancer risk assessment score was 37% and ordered an MRI of her breasts, leading to the discovery of masses.
Soon after, Munn underwent four operations in 10 months: a full double mastectomy, a lymph node dissection, reconstructive surgery and a nipple delay.
“I knew there was cancer happening inside of me, which was already giving me a panic,” Munn said.
After intensive procedures, Munn was placed on Lupron, an estrogen-suppressing drug, which drained her energy.
“The side effects of the medication hit me almost immediately,” she told Vogue. “It was next-level, debilitating exhaustion. I would wake up in the morning and almost immediately need to get back into bed.
“If you asked Malcolm, ‘Where does daddy work?’ He’d run to his [Mulaney’s] desk, and if you asked him, ‘Where does mommy work?’ He’d point to my bed. It was so sweet. But at the same time, it was breaking my heart because this is his image of me,” she continued.
So she asked her doctors whether there was an alternative to Lupron, which led to an oophorectomy, a procedure to remove ovaries, and a hysterectomy, which removes the uterus.
“ I took out my uterus, fallopian tubes and ovaries,” Munn said, noting the procedures took place last month.
The procedures removed hormones from her body to prevent the cancer from returning — but at the cost of carrying any future children.
Before those procedures, she did one last shot at an egg retrieval at age 42 — her third attempt.
“It’s interesting because my 33-year-old eggs were great. My 39-year-old eggs? None of them worked. As you get older, one month can have great eggs, the other not so much. Clearly, the month we did at 39 was not a good month,” she said.
“After my diagnosis, we decided to try one more round of egg retrievals and hoped it was a good month," she said. "John and I talked about it a lot and we don’t feel like we’re done growing our family, but didn’t know if I would have to do chemotherapy or radiation,” which could affect her ability to get pregnant.
The latest round worked, and her doctor called saying she and Mulaney had two healthy embryos.
“John and I just started crying. It was just so exciting because not only did we get it in one retrieval, but it also meant that I didn’t have to keep putting myself at risk. It was just amazing,” Munn said.
“This journey has made me realize how grateful I am to have options for not only fighting cancer, but also having more children if we want, because I know a lot of people don’t have those options,” she added.
On Sunday, Mulaney shared a photo of Munn and Malcolm on his Instagram story, writing: “My son has the most incredible mommy and he knows it. It’s the most loving and silliest relationship I’ve ever seen. Happy Mother’s Day, Liv.”
Breaking News Reporter

An official website of the United States government
The .gov means it’s official. Federal government websites often end in .gov or .mil. Before sharing sensitive information, make sure you’re on a federal government site.
The site is secure. The https:// ensures that you are connecting to the official website and that any information you provide is encrypted and transmitted securely.
- Publications
- Account settings
Preview improvements coming to the PMC website in October 2024. Learn More or Try it out now .
- Advanced Search
- Journal List
- Cancers (Basel)

Targeted Therapies for Pancreatic Cancer: Overview of Current Treatments and New Opportunities for Personalized Oncology
Simple summary.
The lack of early diagnosis and the absence of suitable biomarkers coupled with resistance to available therapeutic options render pancreatic cancer one of the deadliest cancer types. Therefore, new therapeutic approaches are needed to be developed, taking into account the genetic and molecular profile of pancreatic tumors. Here, we critically review past and current efforts that have resulted in the development of potent and specific antitumor compounds that, if employed in the appropriate combination therapy, may change this recalcitrant cancer type into a manageable one.
Cytotoxic chemotherapy remains the only treatment option for most pancreatic ductal adenocarcinoma patients. Currently, the median overall survival of patients with advanced disease rarely exceeds 1 year. The complex network of pancreatic cancer composed of immune cells, endothelial cells, and cancer-associated fibroblasts confers intratumoral and intertumoral heterogeneity with distinct proliferative and metastatic propensity. This heterogeneity can explain why tumors do not behave uniformly and are able to escape therapy. The advance in technology of whole-genome sequencing has now provided the possibility of identifying every somatic mutation, copy-number change, and structural variant in a given cancer, giving rise to personalized targeted therapies. In this review, we provide an overview of the current and emerging treatment strategies in pancreatic cancer. By highlighting new paradigms in pancreatic ductal adenocarcinoma treatment, we hope to stimulate new thoughts for clinical trials aimed at improving patient outcomes.
1. Introduction
Pancreatic ductal adenocarcinoma (thereafter PCa) remains one of the deadliest malignancies with a 5-year overall survival (OS) of only 9% in 2020 [ 1 ]. The reason for this lies on the fact that, due to the late diagnosis, about 80% of patients arriving to the clinic have already locally advanced and unresectable PCa as a result of local invasion of adjacent structures. Based on the tumor stage at the time of diagnosis, PCa can be treated with surgery, chemotherapy, radiation therapy, and targeted therapy with different recommendations [ 2 , 3 , 4 ]. At a resectable PCa setting, surgery can have a curative (when all the tumor can be removed) purpose. According to recent clinical practice guidelines of the American Society of Clinical Oncology, modified FOLFIRINOX (folinic acid, 5-fluorouracil, irinotecan and oxaliplatin, thereafter mFOLFIRINOX) must be the preferred adjuvant therapy for patients with pancreatic adenocarcinoma who have undergone an R0 or R1 resection and have not received prior neoadjuvant chemotherapy [ 5 ]. The term “modified” refers to the reduction of irinotecan from 180 to 150 mg/m 2 and the exclusion of the 5-FU bolus due to the emergence of adverse effects of FOLFIRINOX. Moreover, in the adjuvant/post-operative setting, conventionally fractionated radiation is recommended for patients with high-risk features such as positive lymph nodes and margins. For patients with locally advanced and metastatic disease (unresectable PCa), systemic chemotherapy, generally mFOLFIRINOX or gemcitabine/nab-paclitaxel combination, followed by radiation therapy is recommended, and depending on the presence of alterations, including specific genetic mutations, mismatch repair deficiency, or high microsatellite instability, may receive additional targeted therapies. However, in such advanced stage and due to the aggressive cell biology of PCa with continuing therapy resistance, the available treatment options are not sufficient for curative outcomes. While chemotherapy clearly improves the OS of PCa patients at the preoperative and postoperative setting, radiotherapy is subjected to controversy due to conflicting clinical trial results and its association with a narrow therapeutic index. The impact of radiotherapy in the management of PCa has been extensively reviewed elsewhere [ 6 ].
Aside from the general aging of our society, obesity and type-2 diabetes play a role in the etiology of PCa. In this case, a chronic low-grade inflammation may be a potential mechanism linking obesity to increased PCa incidence and progression [ 7 , 8 , 9 ]. Moreover, lifestyle habits, including alcohol abuse and tobacco use appear to contribute to PCa development [ 10 ]. Lastly, there are some genetic syndromes characterized by specific mutations such as BRCA1 , BRCA2 (Breast and ovarian cancer syndrome) , ATM (Ataxia telangiectasia), STK11 (Peutz-Jeghers syndrome) , PRSS1 (hereditary pancreatitis), MLH1, and MSH2/6 (Lynch syndrome) that are associated with PCa for a subgroup of patients, representing additional risk factors [ 11 ]. A deeper understanding of the pathology of PCa may explain therapeutic resistance, survival differences, and responses to specific therapies. The genetic landscape of PCa is characterized by somatic mutations in one or more of the four major genes: KRAS, CDKN2A, TP53, and SMAD4 [ 12 ]. Besides these mutations, the development of PCa depends on the tumor microenvironment (TME). Targeting single deregulated pathways is often ineffective owing to redundant signaling and complex crosstalk. Moreover, the high degree of inter-tumoral genetic heterogeneity of PCa suggests that it is unlikely that a single targeted therapy will work [ 13 , 14 ]. Therefore, a combination of druggable key signaling hubs needs to be identified and targeted. In this review, we critically summarize the latest targeted combination therapies for advanced/metastatic PCa and discuss new viewpoints for therapeutic approaches currently under preclinical evaluation.
To search for clinical trials, we used the https://www.clinicaltrials.gov (accessed on 20 December 2020) database. We selected for randomized clinical trial studies for PCa patients with reported results (339 studies). From these, we excluded overlapping studies, studies not aimed for combination therapies, and studies for resectable PCa. Moreover, the terminated trials without results are mentioned in the context only if the reason for termination was lack of efficacy and/or toxicity. In addition, when appropriate, we refer to some ongoing clinical trials specially for new targeted therapies, i.e., chimeric antigen receptor T cells (CAR-T) due to the lack of finalized trials.
3. Targeting DNA Repairing Deficiency and Microsatellite Instability
Genomic instability is a key feature of almost all human cancers [ 15 ]. Such modifications benefit the clonal growth of cancer cells, including improvements in gene copy numbers, rearrangements, and mutations. Nevertheless, these same defects often produce cancer cell vulnerabilities that could be used for anticancer therapies. A substantial population of PCa patients harbors germline or somatic mutations in genes that are involved in the DNA damage repair (DDR) pathway, such as BRCA1/2 and ATM [ 16 ], suggesting that these patients may benefit from personalized targeted therapies. Moreover, PCa cells may be selectively sensitive to DDR inhibitors because KRAS mutations, which are present in 95% of PCa cases, are associated with increased replication stress due to depletion of nucleotide pools [ 17 ] and the slowing of replication fork activity [ 18 ].
3.1. PARP Inhibitors
Poly (ADP-ribose) polymerase (PARP) enzymes detect and bind to single-strand DNA breaks (SSB), leading to the recruitment of hundreds of proteins to repair the SSBs. However, if SSBs are not repaired, they cause stall of replications forks and eventually progress to double-strand breaks, which are highly cytotoxic to cells. Thus, cancer cells with mutations that prevent homologous recombination repair, such as BRCA1/2 loss-of-function mutations, are often synthetically lethal with PARP inhibitors due to significantly lower DDR [ 19 ]. A phase II clinical trial with olaparib, a small molecule PARP inhibitor, in a small cohort of BRCA1/2 mutated advanced PCa patients with gemcitabine resistance, evidenced a tumor response rate of 50.0% (4/8 patients), while 25% showed stable disease ≥8 weeks with a median overall survival (OS) of 9.8 months [ 20 ]. Similarly, a phase III clinical trial with olaparib that included 154 metastatic PCa patients with a germline BRCA1 or BRCA2 mutation resulted in a median progression-free survival (PFS) of 7.4 months in the olaparib group vs. 3.8 months of the placebo group [ 21 ]. Thus, the documented efficacy of PARP inhibitors in PCa patients with germline BRCA1 or BRCA2 mutation underscores the importance of germline testing for all patients with PCa.
In addition to PARP inhibitors alone, several trials are currently underway to evaluate PARP inhibitor combinations with other classes of therapies causing DNA damage in PCa patients. However, in >80% of patients, PARP inhibitors, when combined with chemotherapy, including olaparib plus gemcitabine and olaparib plus irinotecan or cisplatin, showed substantial toxicity [ 22 , 23 ]. Clinical trials designed to assess the effectiveness of certain PARP inhibitor combinations of chemotherapy and/or dose-reduced chemotherapy will help decide whether or not such combinations will play a role in providing therapeutic efficacy in PCa patients.
3.2. ATM Inhibition
In contrast to PARP inhibitors, the use of other DDR inhibitors is currently restricted to early clinical studies. Ataxia Telangiectasia Mutated (ATM) is a serine/threonine kinase involved in DDR signaling and it is one of the most commonly mutated DDR genes, with a number of somatic or germline mutations identified in PCa [ 24 , 25 ]. A mouse model of ATM deficient PCa evidenced an increased number of pancreatic intraepithelial precursor lesions, fibrosis, and a greater degree of epithelial to mesenchymal transition compared to the control mice, suggesting a role in PCa progression [ 26 ]. ATM inhibitors such as AZD0156 in combination with olaparib, irinotecan, or fluorouracil in patients with advanced solid tumors are currently in phase I clinical trials ( {"type":"clinical-trial","attrs":{"text":"NCT02588105","term_id":"NCT02588105"}} NCT02588105 ). Cancer cells may compensate the loss of ATM by upregulating ATR, indicating that ATR inhibitors may display efficacy in ATM-deficient tumors, including PCa. However, ATM-deficient PCa cell lines undergo cell death only when incubated with olaparib plus AZD6738, an ATR inhibitor, but neither agent alone [ 27 ]. Thus, patients with ATM deficient PCa may benefit from combination therapies targeting PARP and ATR.
Intriguingly, recent preclinical data showed that the addition of DNA-PK inhibitors with PARP and ATR inhibitors provide synergistic antitumor effects in both ATM-deficient and ATM-proficient cells [ 28 ]. If this approach turns out to be feasible in the clinic, it will considerably extend the target population that can benefit from such combination therapies.
3.3. ATR Inhibition
ATR is one of the primary targets of DDR inhibitors since both SSBs and double-strand DNA breaks (DSBs) are the main regulatory features of ATR [ 29 ]. There are currently five ongoing clinical trials assessing ATR targeting compounds: AZD6738 ( {"type":"clinical-trial","attrs":{"text":"NCT02630199","term_id":"NCT02630199"}} NCT02630199 , {"type":"clinical-trial","attrs":{"text":"NCT03669601","term_id":"NCT03669601"}} NCT03669601 ), M6620 ( {"type":"clinical-trial","attrs":{"text":"NCT03718091","term_id":"NCT03718091"}} NCT03718091 ), M4344 ( {"type":"clinical-trial","attrs":{"text":"NCT02278250","term_id":"NCT02278250"}} NCT02278250 ), and BAY1895344 ( {"type":"clinical-trial","attrs":{"text":"NCT03188965","term_id":"NCT03188965"}} NCT03188965 ). In PDAC cell lines, AZD6738 inhibited gemcitabine-induced Chk1 activation, prevented cell cycle arrest, and strongly induced replication stress. Interestingly, the combination of AZD6738 and gemcitabine induced tumor regression in a subgroup of tumors in the KRAS G12D ; p53 R172H ; Pdx-cre (KPC) mouse model in vivo [ 30 ]. Similar to human PCa, KPC mouse tumors are known to be refractory to therapy, suggesting that the combination of ATR with chemotherapy may be effective in a subset of human PCa patients. M6620 is the first ATR inhibitor tested for monotherapy and combined with various chemotherapies, including topotecan, carboplatin, gemcitabine, and cisplatin [ 31 , 32 , 33 ]. M6620 monotherapy was well tolerated without observation of dose limiting toxicities and the combination with carboplatin showed clinical activity in patients with advanced solid tumors [ 33 ]. Nevertheless, chemotherapy combinations were associated with higher rates of bone marrow toxicity which required M6620 dose reduction. Thus, when used in combination with systemic DNA damaging chemotherapy, it is necessary to optimize the dose/frequency of ATR inhibitors to allow normal tissue recovery. More ongoing phase I clinical trials aim at determining the safety and maximum tolerated dose of ATR inhibitors in combination with chemotherapy in patients with advanced solid tumors. Of these, {"type":"clinical-trial","attrs":{"text":"NCT02630199","term_id":"NCT02630199"}} NCT02630199 and {"type":"clinical-trial","attrs":{"text":"NCT03669601","term_id":"NCT03669601"}} NCT03669601 are testing AZD6738 in combination with paclitaxel and gemcitabine, respectively, while the {"type":"clinical-trial","attrs":{"text":"NCT02278250","term_id":"NCT02278250"}} NCT02278250 aims at testing M4344 in combination with carboplatin.
3.4. DNA-PK Inhibition
The DNA-dependent protein kinase (DNA-PK) is involved in the non-homologous end joining (NHEJ) pathway [ 34 ]. This class of drugs is particularly important in combination with ionizing radiation (IR) because NHEJ is the prevailing repair mechanism for IR-induced DNA double-strand breaks [ 35 ]. Indeed, DNA-PK genetic deficiencies sensitize cells to IR and other DSB-inducing agents [ 36 ]. M3814 (peposertib), a highly potent and selective inhibitor of DNA-PK, sensitized pancreatic cancer cells to IR in vitro and provided complete tumor regression upon treatment with IR in vivo [ 37 ]. In the clinical setting, M3814 has been tested in a phase I clinical trial ( {"type":"clinical-trial","attrs":{"text":"NCT02316197","term_id":"NCT02316197"}} NCT02316197 ) in which it was well-tolerated when given orally as a single agent in doses up to 400 mg BID [ 38 ]. Based on the promising preclinical and clinical trials, M3814 has progressed into a phase I/II clinical testing in combination with hypofractionated radiation therapy for the treatment of unresectable locally advanced PCa ( {"type":"clinical-trial","attrs":{"text":"NCT04172532","term_id":"NCT04172532"}} NCT04172532 ). This study, which is currently recruiting participants, is expected to be concluded in 2024.
3.5. CHK1/2 Inhibition
Cell cycle progression is monitored by mechanisms that control the transition from quiescence (G0) to proliferation, ensuring genetic transcript fidelity. Checkpoint kinase 1 (CHK1) and CHK2 are serine/threonine protein kinases that are part of the recognition of DNA damage. CHK1 is an important signal transducer and the trigger of G2 checkpoint activation, while CHK2 is involved in the repair of DNA, cell cycle, and apoptosis in DDR. CHEK2 gene mutations have been identified in a wide variety of cancers, including PCa [ 39 ]. A CHK1/2 inhibitor, AZD7762, alone or in combination with gemcitabine significantly sensitized PCa cells (MiaPaCa-2) to radiation. Interestingly, the radiosensitization was associated with abrogation of the G2 checkpoint, inhibition of Rad51 focus formation, inhibition of homologous recombination repair, and persistent gamma-H2AX expression [ 40 ]. However, AZD7762 in combination with gemcitabine provided only a partial objective response in gemcitabine-naïve patients ( {"type":"clinical-trial","attrs":{"text":"NCT00413686","term_id":"NCT00413686"}} NCT00413686 ). Moreover, AZD7762 was found to be cardiotoxic, which occurred at doses as low as 30 mg and had to be stopped [ 41 , 42 ].
A phase I/II trial with rabusertib (LY2603618), a highly selective CHK1 inhibitor, assessed whether combination with gemcitabine could prolong OS compared to gemcitabine alone in 99 patients with unresectable PCa ( {"type":"clinical-trial","attrs":{"text":"NCT00839332","term_id":"NCT00839332"}} NCT00839332 ). The results from this study evidenced that the combination of rabusertib with gemcitabine did not confer a greater survival advantage compared to gemcitabine alone [ 43 ]. Recently, another preclinical study showed that prexasertib (LY2606368), a drug currently in phase I clinical trials, increases the sensitivity of PCa cells to gemcitabine and S-1 (an orally available fluoropyrimidine derivative) [ 44 ]. Prexasertib is currently being evaluated in combination with olaparib ( {"type":"clinical-trial","attrs":{"text":"NCT03057145","term_id":"NCT03057145"}} NCT03057145 ) or multiple other targeted drugs ( {"type":"clinical-trial","attrs":{"text":"NCT02124148","term_id":"NCT02124148"}} NCT02124148 ) in advanced solid tumors.
Overall, the lack of obvious clinical efficacy and the reported increased cardiotoxicity warrant further studies to clearly assess whether CHK1 inhibitors can be used for PCa therapy.
The Wee1 protein kinase phosphorylates CDK1 Tyr15 , resulting in G2/M checkpoint activation [ 45 ]. Thus, Wee1 inhibition prevents initiation of G2 checkpoints, causing transformed cells with damaged DNA to go through mitosis and cell death. There are multiple completed or ongoing clinical trials with Wee1 inhibitors in many tumor types that have been extensively reviewed by Ghelli Luserna di Rorà et al. [ 46 ]. One of these clinical trials examined AZD1775, a Wee1 kinase inhibitor, as monotherapy or in combination with chemotherapy (gemcitabine, cisplatin, or carboplatin) in patients with refractory solid tumors ( {"type":"clinical-trial","attrs":{"text":"NCT00648648","term_id":"NCT00648648"}} NCT00648648 ). Of 176 patients that were given combination therapy, 94 (53%) had stable disease and 17 (10%) achieved a partial response. Interestingly, the response rate in TP53 -mutated patients ( n = 19) was 21% compared with 12% in TP53 wild-type patients ( n = 33) [ 47 ]. In PDAC, AZD1775 was tested in a dose escalation study alone or combined with gemcitabine (+radiation) in a cohort of 34 patients with locally advanced unresectable PCa ( {"type":"clinical-trial","attrs":{"text":"NCT02037230","term_id":"NCT02037230"}} NCT02037230 ). In this trial, the combination of AZD1775 with gemcitabine and radiation resulted in an OS of 22 months compared to the 11.9 to 13.6 months of gemcitabine/radiation alone [ 48 ]. Currently, the benefits of adding the AZD1775 into a gemcitabine + nanoparticle albumin-bound (nab)-paclitaxel are being evaluated in a phase I/II clinical trial in patients with previously untreated unresectable or metastatic PCa ( {"type":"clinical-trial","attrs":{"text":"NCT02194829","term_id":"NCT02194829"}} NCT02194829 ).
Preclinical evidence has indicated that Wee1 inhibitors show synergistic effects when combined with histone deacetylase (HDAC) inhibitors [ 49 ], proteasome inhibitors [ 50 ], tyrosine kinase inhibitors [ 51 ], anti-apoptotic protein inhibitors (enhance dependency on BCL-2 and/or MCL-1 inhibition) [ 52 ], and mammalian (or mechanic) target of rapamycin (mTOR) inhibitors [ 53 ]. This latter study is particularly noteworthy for PCa as mTOR inhibition was found to synergize with Wee1 inhibition in KRAS mutant tumors. Future research is necessary to assess whether the combination of Wee1 with mTOR inhibitors may be an effective therapeutic strategy for the treatment of PCa.
4. Targeting Epigenetic Alterations
Despite particular mutations in DNA or loss of genes, the rate of gene expression is regulated by complex mechanisms controlling access to DNA and transcription functionality. Notably, epigenetic changes are considered essential for the initiation and progression of PCa as well as resistance to therapy [ 54 ].
MicroRNAs are non-coding RNAs that interact with mRNA leading to its degradation or reduced translation [ 54 ]. miRNAs regulate and are regulated by a number of key pathways that involve cell differentiation, proliferation, and apoptosis [ 55 ]. In PCa, many miRNAs are consistently upregulated (miR-21, miR-155, and miR-221), while others are downregulated (miR-34, miR-200 family, miR-15a, miR-506, miR-96, miR-145, miR-155) compared to healthy pancreatic tissue. Moreover, the discovery that miRNAs are detectable in blood (miR-21, miR-155, miR-196a, miR-221) [ 56 , 57 ], pancreatic juice (miR-21, miR-155) [ 58 , 59 ] or stool samples [ 60 ] suggests that miRNAs can be used as biomarkers in PCa. Indeed, miRNAs are not only differentially expressed in PCa compared to healthy tissue, but their expression is also strongly associated with PCa staging. In a study with 47 PCa patients evaluating the correlation between plasma miR-221 concentrations and clinicopathological factors, the patients with high plasma miR-221 concentration showed positive correlation with the presence of distant metastasis and advanced (non-resectable) cancer status [ 61 ].
Accumulating evidence suggests that miRNAs by modulating key targets and pathways such as KRAS, PI3K/AKT, TP53, NF-κB, and Hedgehog signaling are associated with resistance of PDAC to chemotherapy. In gemcitabine-treated PDAC cells, the expression of miR-21 dampens the anti-tumor activity of gemcitabine. Moreover, evidence suggests that miR-21 inhibits the tumor suppressor gene, phosphatase and tensin homologue (PTEN), thereby activating the PI3K/AKT pathway. Intriguingly, in human PCa tissue, miR-21 overexpression correlates with worse outcome of patients treated with gemcitabine [ 62 ]. Similarly, Li et al. showed that miR-200b, miR-200c, and let-7 family (let-7b, let-7c, let-7d, let-7e) are down-regulated in gemcitabine-resistant PCa cells. Accordingly, restoration of miR-200 and let-7 resulted in increased PCa sensitivity to gemcitabine [ 63 ]. Moreover, miR-365 overexpression induced gemcitabine resistance by directly targeting the adaptor protein Src homology 2 domain of 1 (SHC1), as well as BAX protein promoters of apoptosis [ 64 ]. Overall, the re-expression or inhibition of miRNAs seems to be an effective strategy for the treatment of PCa, yet more advanced pre-clinical and clinical studies are required to better understand the potential of miRNAs modulation for PCa therapy.
The DNA methyltransferase 1 (DNMT1) is required for DNA methylation during replication [ 65 ]. Zagorac et al. found that pancreatic cancer stem cells (CSCs) evidenced hypermethylation via DNMT1 upregulation. Pharmacologic or genetic targeting of DNMT1 in CSCs reduced their self-renewal and in vivo tumorigenic potential [ 66 ]. Interestingly, aberrant hypermethylation begins at early stages of PanINs and its incidence progressively increases during neoplastic development; therefore, DNMT1 inhibitors are under intense clinical investigation [ 67 , 68 ]. Decitabine (a DNMT1 inhibitor) is being tested in combination with gemcitabine for the treatment of refractory PCa and the study is expected to complete in early 2021 ( {"type":"clinical-trial","attrs":{"text":"NCT02959164","term_id":"NCT02959164"}} NCT02959164 ). Moreover, there is an ongoing clinical trial testing decitabine in combination with anti-PD-1 antibodies and chemotherapy in relapsed or refractory malignancies ( {"type":"clinical-trial","attrs":{"text":"NCT02961101","term_id":"NCT02961101"}} NCT02961101 ). Lastly, a new nucleoside analog, 5-aza-4-thio-2-deoxycytidine (Aza-TdC), has been shown to decrease DNMT1 levels and suppress tumorigenesis in lung tumor xenografts [ 69 ]. Aza-TdC is currently in phase I clinical trials in patients with advanced solid tumors ( {"type":"clinical-trial","attrs":{"text":"NCT03366116","term_id":"NCT03366116"}} NCT03366116 ).
4.3. HATs and HDACs
Targeting the pattern of histone acetylation with either histone deacetylase (HDAC) or histone acetyl transferases (HAT) inhibitors result in increased or decreased histone acetylation, respectively. HDAC inhibitors induce hyperacetylation of histones and thus reactivate tumor suppressor gene expression, leading to suppression of cell proliferation, cell differentiation, and apoptosis [ 70 ]. Although the HAT inhibitors are still in pre-clinical phases, the HDAC inhibitors have progressed into clinical assessment and there have been many clinical phase I/II trials over the years. A phase II randomized clinical study with CI-994, an oral HDAC inhibitor, and gemcitabine in patients with advanced PCa ( {"type":"clinical-trial","attrs":{"text":"NCT00004861","term_id":"NCT00004861"}} NCT00004861 ) did not show greater efficacy compared to gemcitabine alone [ 71 ]. Vorinostat, a HDAC inhibitor, was assessed in phase I trials together with capecitabine and radiation therapy in 21 patients with non-metastatic PCa ( {"type":"clinical-trial","attrs":{"text":"NCT00983268","term_id":"NCT00983268"}} NCT00983268 ). The most common adverse events that were observed with vorinostat were lymphopenia (76%) and nausea (14%), suggesting that the drug is overall well tolerated. However, despite an encouraging median OS, the small number of patients enrolled in this trial prevented the proper evaluation of this therapy [ 72 ]. Moreover, the treatment with panobinostat for patients that progressed on gemcitabine-based therapy ( {"type":"clinical-trial","attrs":{"text":"NCT01056601","term_id":"NCT01056601"}} NCT01056601 ) or advanced solid tumors ( {"type":"clinical-trial","attrs":{"text":"NCT00550199","term_id":"NCT00550199"}} NCT00550199 ) had to be terminated because of a complete lack of treatment response and early treatment-related toxicity [ 73 ]. Thus, HDAC inhibitors seem to have serious adverse effects, requiring careful clinical evaluation. Moreover, patient stratification based on transcriptome or epigenetic signatures is essential for the correct assessment of these targeted therapies.
4.4. Bromodomain Proteins
The limited success of HDAC inhibitors in clinical trials against solid tumors increased the interest in targeting epigenetic “readers” of histone acetylation, in particular members of bromodomain (BRD) and extra terminal domain (BET) family of proteins. These proteins recognize acetylated lysine residues and regulate molecular interactions with relevance to transcriptional control. In PCa, BRD4 was found to promote cell proliferation, enhance gemcitabine resistance [ 74 ], and block the proliferation of PCa cells in three-dimensional collagen [ 75 ]. Moreover, BET inhibition decreased desmoplastic pancreatic stromal cell proliferation and suppressed the growth of patient-derived pancreatic tumor xenografts (PDX) by inhibiting Hedgehog and TGF-β pathways [ 76 ]. I-BET762, a benzodiazepine compound, inhibited PDAC cell proliferation and enhanced the therapeutic effect of gemcitabine in Panc1-derived xenograft tumors [ 77 ]. However, in clinical trials, many BET inhibitors showed adverse effects and lack of efficacy in solid tumors [ 78 ]. Molibresib (GSK525762) was tested in 19 patients with nuclear protein in testis (NUT) midline carcinomas ( {"type":"clinical-trial","attrs":{"text":"NCT01587703","term_id":"NCT01587703"}} NCT01587703 ). NUT midline carcinoma is a rare and invariably lethal cancer caused by the fusion protein BRD4-NUT, which spurs faulty gene expression [ 79 ]. Molibresib was well tolerated, and out of the 19 patients, 4 achieved partial response, 8 had stable disease as best response, and 4 were progression-free for more than 6 months, providing a promising future for this inhibitor [ 80 ]. MK-8628, an oral BET inhibitor targeting BRD2 and BRD4 is in phase Ib clinical trial in patients with selected advanced solid tumors, but 38/46 (83%) patients manifested treatment-related adverse events ( {"type":"clinical-trial","attrs":{"text":"NCT02259114","term_id":"NCT02259114"}} NCT02259114 ) [ 81 ]. Moreover, another clinical study of 13 patients with MK-8628 was terminated due to limited efficacy ( {"type":"clinical-trial","attrs":{"text":"NCT02698176","term_id":"NCT02698176"}} NCT02698176 ).
In summary, epigenetic approaches to PCa therapy have considerable potential but face challenging clinical translation due to poor response and treatment-related adverse events. In order to effectively implement this clinical approach, it is important to first determine the tumor subtypes and specific cancer vulnerabilities to tailor specific drug combinations.
5. Targeting Key Signaling Pathways
5.1. kras and main targets.
KRAS is mutated in >90% of PDACs [ 82 ]. This high prevalence has contributed to considerable interest in therapies that selectively target mutated KRAS. Inhibitors targeting KRAS G12C are currently in clinical development (i.e ARS-1620 and AMG 510), showing promising results in colorectal and lung cancers [ 83 , 84 ]. However, KRAS G12C mutations are rare in PCa (~1% of all KRAS mutations), excluding them as a therapeutic option. Because inhibitors that specifically target the most prevalent KRAS mutations in PCa do not exist, there is a lot of interest in developing means to deliver small interfering RNAs (RNAi) in vivo via exosomes or small extracellular vesicles ( Figure 1 ). This strategy has recently entered a phase-I trial for metastatic PCa patients and is expected to be completed in early 2022 ( {"type":"clinical-trial","attrs":{"text":"NCT03608631","term_id":"NCT03608631"}} NCT03608631 ).

Targeting key signaling pathways in patients with pancreatic ductal adenocarcinoma (PCa). EGFR, epidermal growth factor receptor; RAS, rat sarcoma viral oncogene; AKT, protein kinase B; CDK4/CDK6, cyclin-dependent kinase 4/6; RAF, rapidly accelerated fibrosarcoma; MEK, mitogen-activated protein kinase; ERK, extracellular signal-regulated kinases; PI3K, phosphoinositide 3-kinase; mTOR, mechanistic target of rapamycin; FAK, focal adhesion kinase; BTK, Bruton’s tyrosine kinase.
Another approach that is currently being tested to target mutated KRAS is with peptide-based vaccines. This approach is described in the context of the “vaccine therapy” section below.
In view of the difficulty to directly target KRAS, during the last four decades efforts were focused on developing treatments aimed at targeting its main downstream effector pathways, including the RAS-RAF-MEK-ERK and the PI3K-AKT-mTOR signaling pathways ( Figure 1 ). However, in patients with metastatic PDAC, no clinical benefit was observed with MEK inhibitors plus gemcitabine compared with gemcitabine alone ( {"type":"clinical-trial","attrs":{"text":"NCT01016483","term_id":"NCT01016483"}} NCT01016483 , {"type":"clinical-trial","attrs":{"text":"NCT01231581","term_id":"NCT01231581"}} NCT01231581 ) [ 85 , 86 ]. Despite these deceptive findings, recent research has helped to clarify a potential resistance mechanism of PCa to MAPK inhibition. Indeed, results by two different research groups showed that pharmacological inhibition of the ERK or MEK signaling pathway in KRAS-mutant PCa elicited a protective increase of autophagy [ 87 , 88 ]. Moreover, related to MAPK inhibition, a recent study revealed that combination MEK1/2 and CDK4/6 inhibitors triggers senescence-associated secretory phenotype (SASP)–mediated increase in CD31 + cells and endothelial activation, promoting CD8 + T cell tumor infiltration in PCa [ 89 ]. Consequently, the addition of gemcitabine or anti-programmed death-1 (anti-PD-1) therapy to the above-described combination resulted in tumor regression, suggesting that combining senescence-inducing therapies with both chemotherapy and immunotherapy may be an effective strategy to treat PCa. However, care should be taken to first assess the impact of this therapeutic regiment in metastatic PCa because of the increase in CD31 + . Even though it suppresses the primary tumor, it may contribute to the metastatic potential.
There are multiple alterations affecting the PI3K pathway in PCa. PCa has been shown to harbor activating mutations in PIK3CA (~4%) and/or loss of the tumor suppressor PTEN (25–70%) [ 82 , 90 ]. Moreover, increased AKT activity has been identified in about 60% of PCa samples and amplification of AKT2 occurs in 10–20% of the cases [ 91 ]. This mutational profile, together with the highly promising preclinical antitumor efficacy of the PI3K pathway inhibitors in combination with clinically relevant interventions [ 92 , 93 , 94 ], provided the rationale for the initiation of clinical trials. A first clinical trial with Copanlisib (BAY 80-6946), a potent pan-class I PI3K inhibitor, showed promising anti-tumor pharmacodynamic activity and clinical benefit in patients with advanced solid tumors, including PCa ( {"type":"clinical-trial","attrs":{"text":"NCT00962611","term_id":"NCT00962611"}} NCT00962611 ) [ 95 ]. In parallel, BKM120, a pan-class I PI3K inhibitor, was tested in combination with mFOLFOX6 (5-FU/leucovorin plus oxaliplatin) in metastatic PCa patients ( {"type":"clinical-trial","attrs":{"text":"NCT01571024","term_id":"NCT01571024"}} NCT01571024 ). Unfortunately, this combination resulted in increased toxicity compared with that expected from either the PI3K inhibitor or the chemotherapy alone [ 96 ]. Similarly, increased toxicity was found when BKM120 was given in combination with the MEK inhibitor, trametinib (GSK1120212), in patients with advanced BRAF/KRAS mutant solid tumors ( {"type":"clinical-trial","attrs":{"text":"NCT01155453","term_id":"NCT01155453"}} NCT01155453 ) [ 97 ]. This toxicity was somehow expected as also other PI3K or AKT or dual PI3K/mTOR and MEK inhibitor combination studies have shown similar toxicity, with fatigue, gastrointestinal, and cutaneous toxicity being most predominant [ 97 , 98 , 99 ]. Currently, there is an ongoing clinical trial with a dual PI3K/mTOR inhibitor, gedatolisib (PF-05212384), in combination with a CDK4/6 inhibitor, palbociclib (PD-0332991), for patients with advanced solid tumors including PCa. These trials will assess the safety and efficacy of these compounds in order to determine whether these combinations can be applied in PCa patients.
Lastly, there are multiple trials focusing on mTOR inhibitors ( Figure 1 ). AZD2014, a potent dual mTORC1 and mTORC2 inhibitor showed promising preclinical results in the KPC ( LSL-Kras G12D ; Trp53 R172H ; Pdx1-Cre ) mouse model. However, the first phase I clinical study with AZD2014 evidenced that, out of the 56 patients, only 2 showed confirmed partial response. Interestingly, one patient with confirmed partial response was a patient with acinar PCa bearing KRAS , PDGFRA , APC , ERB4 , KIT, and FBXW7 mutations [ 100 ]. AZD2014 was also tested in a cohort of 27 patients with TSC1/2 mutated refractory solid cancer as monotherapy ( {"type":"clinical-trial","attrs":{"text":"NCT03166176","term_id":"NCT03166176"}} NCT03166176 ). However, the study was withdrawn due to lack of efficacy. AZD2014 is currently undergoing evaluation in combination therapies in patients with solid tumors, including one with the Bcl-2 inhibitor, navitoclax ( {"type":"clinical-trial","attrs":{"text":"NCT03366103","term_id":"NCT03366103"}} NCT03366103 ) or with selumetinib (AZD6244), a MEK1/2 inhibitor ( {"type":"clinical-trial","attrs":{"text":"NCT02583542","term_id":"NCT02583542"}} NCT02583542 ).
Preclinical research has focused on restoring/reactivating wild-type p53 or to destabilize mutant p53 [ 11 , 101 ]. Statins recently showed a promising potential in depleting mutant p53 via inhibition of the mevalonate pathway [ 102 ]. Nevertheless, either ineffective or sluggish clinical translation of targeting p53 was achieved [ 103 ]. The safety of SGT-53, a liposomal formulation encapsulating a plasmid carrying a human wild-type p53, has been recently evaluated in a phase I trial for the treatment of solid tumors ( {"type":"clinical-trial","attrs":{"text":"NCT00470613","term_id":"NCT00470613"}} NCT00470613 ) [ 104 ]. This trial not only showed that systemically delivered SGT-53 is well tolerated and exhibits anticancer activity, but also provided evidence of targeted delivery of SGT-53 to metastatic lesions. A phase II trial is currently carried out in combination with gemcitabine/nab-paclitaxel and SGT-53 for metastatic PCa and is expected to be finalized at the end of 2021 ( {"type":"clinical-trial","attrs":{"text":"NCT02340117","term_id":"NCT02340117"}} NCT02340117 ).
SMAD4 mutations are widespread in PCa patients (detected in 16–44% of patients) [ 105 ]. Loss of SMAD4 accelerates PCa progression and correlates with metastasis and poor prognosis [ 106 ]. Recent evidence suggested that metformin inhibits PCa progression and improves the survival of patients with SMAD4-deficient PCa, while this is not the case in patients with SMAD4-normal PCa [ 107 ]. These findings suggest that metformin could be used to selectively treat patients with SMAD4 deficiency and should be further investigated in the clinical setting.
5.4. Tyrosine Kinase Signaling
Tyrosine kinases (TKs) play an important role in malignancy [ 108 ]. They are able to initiate a range of intracellular signals that govern proliferation, cell survival, transformation, differentiation, migration, and metastases [ 109 , 110 ]. Compared to other mutant KRAS tumors, such as lung cancer, the activation in TKs is not a common future of PCa, yet targeting TKs was proved to prevent the insurgence of resistance mechanisms [ 111 ]. The epidermal growth factor receptor (EGFR) inhibitor, erlotinib, is one of the only targeted drugs with significant survival benefit in the clinic that received approval for the treatment of patients with metastatic PCa ( Figure 1 ). Erlotinib treatment prolongs the survival of PCa patients partly by blocking gemcitabine-induced MAPK signaling activation [ 112 ]. Nimotuzumab, which is a humanized EGFR-optimized antibody, increased the 1-year survival rate of PCa patients by 2-fold in a phase II trial randomized (gemcitabine plus nimotuzumab vs. gemcitabine plus placebo) [ 113 ]. Oddly, the patients carrying wild type KRAS showed better survival compared to those carrying mutant KRAS.
The ERBB2 ( HER2 ) gene is frequently amplified (24%) in PCa, and it is associated with worse prognosis partly by mediating resistance to gemcitabine and irinotecan/SN-38 treatment [ 114 , 115 ]. Afatinib, an irreversible EGFR, HER2, and HER4 small molecule inhibitor, is currently being evaluated in clinical studies in combination with capecitabine ( {"type":"clinical-trial","attrs":{"text":"NCT02451553","term_id":"NCT02451553"}} NCT02451553 ) or selumetinib (AZD6244), a MEK1/2 inhibitor ( {"type":"clinical-trial","attrs":{"text":"NCT02450656","term_id":"NCT02450656"}} NCT02450656 ) for the treatment of advanced stage PCa or with gemcitabine/nab-paclitaxel for metastatic PCa ( {"type":"clinical-trial","attrs":{"text":"NCT02975141","term_id":"NCT02975141"}} NCT02975141 ).
Focal adhesion kinase (FAK) is a non-receptor TK with a role in invasion, growth, and metastasis, which is found elevated in high-grade mutant KRAS non-small cell lung cancer and PCa [ 116 , 117 ]. In PDAC, FAK interacts with both tumor and stromal cells and its activity levels are associated with immunosuppressive TME and poor survival. The administration of a FAK inhibitor (VS-6063) in combination with gemcitabine and nab-paclitaxel has been shown to delay tumor growth in PDX models compared to chemotherapy alone [ 118 ]. Moreover, a FAK inhibitor (VS-4718) in the KPC mouse model (p48-CRE; LSL-KRas G12D/wt ; p53 flox/wt ) decreased fibrosis, TAMs, myeloid-derived suppressor cells (MDSCs), regulatory T (Treg) cell infiltration, and improved mice survival [ 117 ]. However, the signal transducer and activator of transcription 3 (STAT3) signaling-triggered compensatory survival pathways and rendered tumors resistant to FAK inhibition [ 119 ]. Given the role of FAK in modulating the TME, a clinical trial is in progress to examine the interaction of FAK inhibitors with immune checkpoint inhibitors (defactinib/pembrolizumab: {"type":"clinical-trial","attrs":{"text":"NCT02758587","term_id":"NCT02758587"}} NCT02758587 ). Moreover, another ongoing trial is currently evaluating whether reprograming the tumor microenvironment by targeting FAK following chemotherapy can potentiate the anti-PD-1 antibody-mediated anti-tumor response ( {"type":"clinical-trial","attrs":{"text":"NCT03727880","term_id":"NCT03727880"}} NCT03727880 ).
The non-receptor TK, Bruton’s tyrosine kinase (BTK), plays a role in proliferation of leukemic cells in many B-cell malignancies [ 120 ]. The BTK inhibitor, ibrutinib, has already shown clinical activity in hematological malignant patients. In mouse models of PCa, administration of ibrutinib contributed to the reprogramming of M2 macrophages to an antitumor M1 phenotype that boosted CD8+ T cell cytotoxicity and suppressed PCa growth [ 121 ]. A combination therapy has been carried out with acalabrutinib (ACP-196), a BTK inhibitor, in combination with pembrolizumab (an FDA approved checkpoint inhibitor targeting PD-1) in patients with advanced or metastatic PCa ( {"type":"clinical-trial","attrs":{"text":"NCT02362048","term_id":"NCT02362048"}} NCT02362048 ) [ 122 ]. The results from this trial evidenced that although the combination of acalabrutinib and pembrolizumab was well tolerated and peripheral reduction of MDSCs was observed, the overall response rate was limited for both regimens, with responses in 0% of patients undergoing monotherapy and 7.9% of those receiving combination therapy.
6. Targeting the Tumor Microenvironment and Related Metabolic Reprogramming
The TME of PCa consists of stromal cells, a dense ECM, and immune cells. PCa stroma is a crucial feature, regulating tumor growth, vascularization, drug responsiveness, immune landscape, and metastasis. Indeed, stromal fibroblasts (termed cancer associated fibroblasts, CAFs) play an important role in promoting PCa progression and in dampening chemotherapeutic response [ 123 ]. The ECM that occupies the bulk of tumor mass is a dense network of structural and adaptor proteins, proteoglycans, and enzymes [ 124 ]. Among these components, hyaluronic acid (HA), a glycosaminoglycan, limits the accessibility of chemotherapeutic agents to cancer cells by increasing interstitial pressure leading to vascular collapse and reduced tumor perfusion [ 125 ]. Extensive preclinical research evidenced that recombinant human hyaluronidase 20 (PEGPH20) reduces total intratumoral pressure and vascular collapse [ 125 , 126 ], which encouraged further clinical testing. However, among the trials that have been performed in combination with chemotherapy in metastatic PCa patients provided discouraging outcomes ( Table 1 ). One trial in unselected for tumor HA status patients reported treatment-related grade 3 to 4 toxicity in the PEGPH20 + mFOLFIRINOX arm vs mFOLFIRINOX alone and failed to show any difference in OS [ 127 ]. Similarly, a phase III trial of gemcitabine plus nab-paclitaxel with or without PEGPH20 failed to meet the expected primary end point of OS or PFS and had to be terminated ( {"type":"clinical-trial","attrs":{"text":"NCT02715804","term_id":"NCT02715804"}} NCT02715804 ) [ 128 ]. Currently there is an ongoing trial of PEGPH20 in combination with pembrolizumab for patients with previously treated, HA-high metastatic PCa ( {"type":"clinical-trial","attrs":{"text":"NCT03634332","term_id":"NCT03634332"}} NCT03634332 ).
Summary of clinical trials modulating the tumor microenvironment and metabolic reprogramming in PCa patients.
Gem: Gemcitabine; nab-PTX: Nab-paclitaxel; HCQ: Hydroxychloroquine; OS: Overall survival; PFS: Progression-free survival.
Given the rich stromal component of PCa TME, one would expect that eliminating stromal components may be an effective strategy for PCa therapy. However, preclinical studies proved that this is not the case because radical stromal depletion via Hedgehog deletion resulted in more aggressive, poorly differentiated, and highly vascularized tumors [ 137 ]. Similarly, complete depletion of αSMA+ fibroblasts during PCa development accelerated disease progression and decreased mouse survival [ 138 ]. Importantly, two clinical trials with vismodegib, a Hedgehog pathway inhibitor, in combination with gemcitabine or gemcitabine/nab-paclitaxel in metastatic PCa patients did not improve the overall response rate, PFS, or OS compared to gemcitabine or gemcitabine/nab-paclitaxel alone ( Table 1 ) [ 129 , 130 ]. On the other hand, suppression of the lipid-triggered pro-fibrotic plasminogen activator inhibitor-1 (PAI-1) pathway limited desmoplasia and immunosuppression, and delayed tumor progression without enhancing tumor vascularization in preclinical KPC and orthotopic mouse models of PCa [ 139 ]. These results suggest that limiting some stromal components rather than completely eliminating them may help to gradually allow therapeutic agents to reach tumors without risking enhancing metastases formation. Currently, there are many ongoing clinical trials targeting different aspects of stromal cell biology, which have been extensively summarized by Hosein and colleagues [ 140 ].
The previously described desmoplastic environment of PCa limits the availability of nutrients and oxygen in cancer cells. Indeed, PCa is highly hypoxic, with median pO2 < 5.3 mmHg compared to the surrounding normal pancreatic tissue where the median pO2 reaches 24.3–92.7 mmHg [ 141 ]. Moreover, due to the already highly hypoxic environment of PCa, anti-angiogenic therapy is not an option as worsens tumor hypoxia, promoting chemoresistance, cancer stem cell enrichment, invasion, and metastasis [ 142 , 143 ]. Indeed, chronic treatment with an anti-vascular endothelial growth factor (anti-VEGF) antibody in a mouse PCa model ( LSL-Kras G12D ; Cdkn2a lox/lox ; p48 Cre ) increased collagen deposition, epithelial plasticity, and metastasis [ 144 ]. Accordingly, a series of phase III clinical trials with VEGF inhibitors, such as bevacizumab (a humanized anti-VEGF-A monoclonal antibody), axitinib, or sorafenib with various drug combinations (gemcitabine or gemcitabine/erlotinib) in patients with advanced or metastatic PCa failed to improve OS ( Table 1 ) [ 131 , 132 , 133 , 134 ].
In absence of oxygen and nutrients, cancer cells must rely on several metabolic rearrangements for survival such as a shift from oxidative phosphorylation to glycolysis, inhibition of fatty-acid desaturation, increased scavenging of lipids and proteins and high basal autophagy ( Figure 2 ) [ 145 , 146 , 147 , 148 , 149 ]. An acute effect of hypoxia is the shift from oxidative phosphorylation to glycolysis due to hypoxia inducible factor 1a (HIF1a) stabilization [ 150 ]. As the glycolytic shift of hypoxic tumors could be considered as a targetable vulnerability, attempts have been made to develop inhibitors that would target the glycolytic pathway; 2-deoxy-D-glucose (2DG) is a synthetic glucose analog and phase I clinical trials in solid tumors evidenced that 2DG can be safely combined with docetaxel because the adverse effects were tolerable ( {"type":"clinical-trial","attrs":{"text":"NCT00096707","term_id":"NCT00096707"}} NCT00096707 ) [ 151 ]. However, since then, there have been no new clinical trials assessing 2DG in combination with other compounds. Another promising approach would be to target lactate transporters (monocarboxylate transporters: MCTs). MCT1/2-selective inhibitors (AZD3965) are currently being tested in advanced tumors ( {"type":"clinical-trial","attrs":{"text":"NCT01791595","term_id":"NCT01791595"}} NCT01791595 ). However, one patient developed malignant hyperlactaemic acidosis upon treatment with AZD3965 due to a preexisting metabolic disorder (hyper-Warburgism) [ 152 ]. Thus, patients with elevated plasma lactate must be excluded from the treatment with MCT inhibitors ( Figure 2 ).

Targeting metabolic reprogramming in patients with PCa. KRAS-mutant tumors rely on metabolic reprogramming, exposing critical vulnerabilities to target for therapy. MCT1/2, monocarboxylate transporters 1/2; ACSL3, acyl-CoA synthetase long-chain 3.
KRAS-transformed cells scavenge extracellular proteins through macropinocytosis as a major source of amino acids, which are the source of the central carbon metabolism and tricarboxylic acid cycle [ 147 , 148 ]. Inhibition of macropinocytosis by amiloride inhibited in vitro PCa cell proliferation and tumor growth in vivo [ 153 , 154 ], suggesting that that may be an interesting strategy in clinical trials for PCa therapy ( Figure 2 ). Similarly, KRAS-mutant tumors rely on scavenging of extracellular lipids [ 149 , 155 , 156 ], which is a potential vulnerability. Indeed, we showed that inhibition of acyl-CoA synthetase long chain 3 (ACSL3), an enzyme that activates extracellularly-derived fatty acids by adding a CoA moiety, delayed tumor growth, suppressed fibrosis, and enhanced the activation and abundance of CD8+ T cells in KPC mice [ 139 ].
Autophagy is a catabolic process of degradation in which autophagosomes engulf macromolecules and specific organelles and then fuse with lysosomes to provide the cell with recycled building blocks and substrates for metabolism and energy ( Figure 2 ) [ 157 ]. PCa cancer cells have been shown to actively use high autophagy to survive and proliferate [ 158 ]. The antimalarial drug chloroquine inhibits autophagy and suppresses the proliferation of PCa cells in vitro, leading to xenografted mouse tumor regression [ 158 ]. In the pre-operative setting, the combination of hydroxychloroquine (HCQ) with gemcitabine/nab-paclitaxel evidenced improved tumor and serum biomarker response (CA 19-9) and enhanced tumor immune cell infiltration compared to gemcitabine/nab-paclitaxel alone [ 135 ]. Despite these promising trials, preliminary results of a similar ongoing study in metastatic PCa patients ( {"type":"clinical-trial","attrs":{"text":"NCT01506973","term_id":"NCT01506973"}} NCT01506973 ) showed that the addition of HCQ to gemcitabine/nab-paclitaxel did not improve the primary endpoint of overall survival at 12 months [ 136 ], suggesting that more rationalized approaches must be followed ( Table 1 ). For instance, upon certain interventions, autophagy has been shown to be highly activated acting as a pro-survival mechanism [ 87 , 88 ], indicating that, in some contexts, autophagy inhibitors may be particularly successful.
7. Targeting Immune Regulatory Networks
The induction of an immune response against tumors in many advanced-stage cancers has been proven to be extremely effective. However, like with other antitumor strategies, PCa is highly refractory. The reason for this is that the TME of PCa is occupied by an impressive number of highly immunosuppressive cell populations, namely tumor associated macrophages (TAMs), myeloid-derived suppressor cells (MDSCs), neutrophils, and regulatory T cells (Tregs) that allow immune evasion and limit the effectiveness of chemotherapy ( Figure 3 ) [ 159 ]. Moreover, TAMs not only affect the activity of cytidine deaminase, a key enzyme in gemcitabine metabolism, hence driving resistance to gemcitabine-based chemotherapy [ 160 ], but also mediate angiogenesis by releasing cytokines and growth factors such as VEGF [ 161 ]. Furthermore, because PCa is known to contain relatively few genomic mutations in protein coding regions, there is a limited number of neoantigens that could be used for immunotherapy. Several immunotherapeutic strategies (vaccination, adoptive cell transfers, and targeting immune checkpoints) are being evaluated in PCa. The choice of the chemotherapeutic drug to combine with immunotherapy has to be carefully considered since many chemotherapeutic drugs suppress immune activation. Chemotherapy that is able to induce immunogenic cell death such as oxaliplatin, cyclophosphamide, and gemcitabine are preferred [ 162 ]. For instance, immediately after the initial course of gemcitabine treatment, naïve, activated immune functions are triggered [ 163 ]. However, there is also a decline in memory T cells, suggesting that rationalized treatment protocols must be used in clinical trials when combining chemotherapy with immunotherapy.

Targeting immune regulatory networks in PCa patients. Some of the current actionable interventions for pancreatic cancer.
7.1. Vaccine Therapy
Vaccines prime the patient’s own T cells against cancer-specific antigens, promoting effective anticancer immunity. Cancer vaccines which can be whole cell, peptide-, dendritic cell (DC)- or DNA-based, have shown high potential in triggering persistent increase in T cell response, can be easily evaluated for personalized target development and, most importantly, are well tolerated ( Table 2 ).
Summary of clinical trials targeting immune regulatory networks.
CP: Cyclophosphamide; Gem: Gemcitabine; CT: Chemotherapy; nab-PTX: Nab-paclitaxel; HCQ: Hydroxychloroquine; OS: Overall survival; PFS: Progression-free survival; CR: Complete response; PR: Partial response; SD: Stable disease; PD: Progressive disease; Combo: Combination; #: means the patient number.
GVAX pancreas is an irradiated allogeneic whole pancreatic tumor cell vaccine in which cancer cells are engineered to express granulocyte-macrophage colony stimulating factor (GM-CSF). GVAX pancreas combined with low dose cyclophosphamide to inhibit Treg cells, and a cancer vaccine, CRS-207 (live, attenuated Listeria monocytogenes expressing mesothelin), which stimulates innate and adaptive immunity in previously treated metastatic PCa, improved OS of patients compared to historical OS achieved with chemotherapy ( {"type":"clinical-trial","attrs":{"text":"NCT01417000","term_id":"NCT01417000"}} NCT01417000 ) [ 179 ]. However, when a phase IIb trial was performed in a bigger cohort of previously treated metastatic PCa patients with cyclophosphamide + GVAX + CRS-207 the OS did not improve compared to chemotherapy ( Table 2 ) [ 164 ].
Regarding peptide-based vaccination, administration of a cocktail containing mutated RAS peptides combined with GM-CSF in advanced PCa patients showed vaccine-induced immune response—32% of the patients had stable disease after peptide vaccination and all of the patients with stable disease showed an immunological response, while 45% of patients showing an immune response to the vaccine had progression of disease [ 165 ]. This triggered a second study in which previously resected PCa patients, treated as above (but with a pool of 7 peptides against mutant RAS) were followed up for 10 years [ 166 ]. This study showed the persistence of T cells recognizing vaccine peptides many years after the last vaccination ( Table 2 ). However, in a pilot study, out of the 9 evaluable patients that were vaccinated with a 21-mer peptide containing the corresponding KRAS mutation of the patient’s tumor, only 1 patient showed immune response [ 180 ]. In this case, the extremely low number of patients, coupled with the inconsistent number of vaccinations performed, may have limited this study. Taken together, these results suggest that cancer peptides targeting mutant KRAS should be included in the standard of care of all PCa patients, but before this, carefully designed combination therapies need to be performed ( Figure 3 ).
Another tumor-specific antigen that has been tested in PCa is the human telomerase reverse transcriptase (hTERT) with the GV1001 vaccine consisting of 16 amino acids of hTERT. A phase III trial of 1062 patients treated either with gemcitabine/capecitabine alone or concurrently or sequentially with GV1001 provided no survival benefit in patients with advanced PCa. However, T cell proliferation was positive in 31% of patients given sequential immunotherapy and 14.7% of patients given concurrent chemoimmunotherapy, suggesting that sequential immunotherapy should be preferred [ 167 ].
Survivin, a member of the inhibitor of apoptosis (IAP) family, is another potentially attractive target. A phase II study in advanced, previously treated PCa patients with SVN-2B, a peptide derived from survivin 2B protein in combination with interferon β (IFNβ), showed longer OS in patients receiving the combination vs SVN-2B alone or placebo [ 168 ]. These data also show that IFNβ is a promising adjuvant for peptide vaccination therapy. Moreover, it was subsequently shown that treatment with SVN-2B triggered a dense infiltration of CD8 + T cells in some patient lesions and a high rate of programmed cell death ligand 1 (PD-L1) expression in cancer cells, indicating emergence of resistance against CTL attack [ 181 ]. This clearly provides a rationale for the combination of SVN-2B with anti-PD1 or anti-PD-L1 therapies.
DCs are powerful antigen presenting cells that can be manipulated in vitro to develop a cancer vaccine in order to increase antigen presentation, break tolerance against tumor-associated antigens where it is lacking, or enhance T cell priming ( Figure 3 ). Unfortunately, the clinical studies performed with DC-based vaccines are only limited to early phase with a small number of patients. Of these, one study used gemcitabine followed by DCs pulsed with the MHC-I, -II, or -I/II–restricted epitopes of Wilms tumor (WT1), which is highly expressed in PCa [ 169 ]. Importantly, 50% of the patients showed increased OS, PFS, and specific T cell response.
7.2. Monoclonal Antibodies
CD40 activation can reverse immune suppression and drive antitumor T cell responses ( Figure 3 ) [ 182 ]. Preclinical evidence in KPC mice (Kras LSLG12D/+ , Trp53 LSL-R172H/+ , Pdx1-Cre) suggested that CD40 agonist antibody combined with immune checkpoint inhibitors (ICI), trigger tumor regressions, and immunological memory. Of note, this is not the case when ICIs are given alone [ 183 ]. The combination of a CD40 agonist antibody with gemcitabine in a small cohort of patients with chemotherapy-naïve, surgically incurable PCa evidenced improved OS and PFS ( Table 2 ) [ 170 ]. Moreover, there is an ongoing trial testing the APX005M (CD40 antibody) with gemcitabine and nab-paclitaxel with or without nivolumab (anti-PD-1) in patients with previously untreated metastatic PCa ( {"type":"clinical-trial","attrs":{"text":"NCT03214250","term_id":"NCT03214250"}} NCT03214250 ) or CDX-1140 (CD40 antibody), either alone or in combination with CDX-301 (FLT3L), pembrolizumab or chemotherapy ( {"type":"clinical-trial","attrs":{"text":"NCT03329950","term_id":"NCT03329950"}} NCT03329950 ). Given the previously reported antitumor efficacy of CD40 agonists when combined with ICIs and their capacity to stimulate tumoricidal macrophage infiltration in tumors [ 170 ], if safe, these combinations are promising in bringing new hope for PCa treatment.
7.3. Immune Checkpoints Inhibitors
The objective of ICIs is to intensify existing anti-cancer responses by improving stimulatory or blocking activity of the immune system regulators to allow better clearing of cancer cells ( Figure 3 ). Monotherapy with ipilimumab (anti CTLA-4) on locally advanced and metastatic PCa did not show any benefit ( {"type":"clinical-trial","attrs":{"text":"NCT00112580","term_id":"NCT00112580"}} NCT00112580 ). Moreover, combination of ipilimumab with gemcitabine did not prove to be more effective than gemcitabine alone in advanced PCa in one phase Ib trial [ 171 ]. However, when gemcitabine was combined with 10 or 15 mg/kg tremelimumab, the OS was longer compared to historical data of gemcitabine monotherapy, suggesting that a high dose of ICIs should be obtained for increased efficacy ( Table 2 ) [ 172 ]. Interestingly, when ipilimumab was combined with GVAX in patients with previously treated PDAC, 3 out of 15 patients showed stable disease, and 7 out of 15 patients manifested a decline in CA19-9 [ 173 ]. Pembrolizumab (anti PD-1) is approved for patients with advanced PCa that harbor high microsatellite instability, DNA mismatch repair deficiency, or high tumor mutational burden. A phase Ib/II trial of a combination of pembrolizumab with gemcitabine/nab-paclitaxel in chemotherapy naïve metastatic patients showed improved efficacy vs historical gemcitabine/nab-paclitaxel [ 174 ].
Based on recent preclinical and diagnostic approaches, CD73, a nucleotide metabolizing enzyme that sustains immune homeostasis, is highly expressed in tumor PCa cells and it is associated with poor survival independently of the number of tumor-infiltrating lymphocytes or TNM stage [ 184 ]. Clinical trials combining a CD73 Inhibitor, LY3475070 ( {"type":"clinical-trial","attrs":{"text":"NCT04148937","term_id":"NCT04148937"}} NCT04148937 ) or CPI-006 ( {"type":"clinical-trial","attrs":{"text":"NCT03454451","term_id":"NCT03454451"}} NCT03454451 ) alone or in combination with pembrolizumab in patients with advanced cancers, including PCa are currently ongoing. Thus, CD73 may be a novel immunotherapeutic target and a promising immune prognostic biomarker for PCa in the future.
7.4. Adoptive Cell Transfer Therapy
Adoptive cell transfer (ACT) therapy uses ex vivo expanded tumor-infiltrating lymphocytes and is the most prominent form of immunotherapy with impressive results in B-cells malignancies and 20–25% of patients with metastatic melanoma [ 185 , 186 ]. Two trials assessing ex vivo-expanded, cytokine-induced killer (CIK) cells in gemcitabine-refractory advanced PCa, reported no OS or PFS improvement in patients compared with previous trials, yet the treatment improved patients’ quality of life (pain, gastrointestinal distress, jaundice, body image alterations, altered bowel habits, health satisfaction, and sexuality) [ 176 ]. Related to mutant KRAS targeting, a phase II clinical trial designed to test whether the ACT of ex vivo expanded tumor-infiltrating lymphocytes targeting personalized cancer neoepitopes can mediate regression of metastatic solid cancers, identified CD8 + T cells reactive to KRAS G12D ( {"type":"clinical-trial","attrs":{"text":"NCT01174121","term_id":"NCT01174121"}} NCT01174121 ). Expansion and infusion of KRAS G12D reactive CD8 + T cells back to a colorectal cancer patient resulted in regression of metastatic lung lesions [ 187 ]. If successful, this may be a potential strategy to provide in combination studies for PCa treatment.
Currently, there are many ongoing chimeric antigen receptors (CAR)-T cell therapies for PCa. In a phase I study, patients with chemotherapy-refractory metastatic PDAC, received T cells engineered to transiently express an mRNA encoding a CAR specific for mesothelin ( Table 2 ) [ 177 ]. The results from this trial revealed stable disease in 2 out of the 6 patients, underscoring the potential of mesothelin-specific CAR T cell therapy for PCa. Moreover, a phase I/II adoptive T cell trial in 7 locally advanced and metastatic PCa patients using infusions of anti-CD3/anti-EGFR bispecific antibody armed activated T cells, induced anti-cancer cytotoxicity, and increased innate immune responses [ 178 ].
Lastly, there are many ongoing clinical studies against multiple PCa relevant targets such as prostate stem cell antigen (PSCA) {"type":"clinical-trial","attrs":{"text":"NCT02744287","term_id":"NCT02744287"}} NCT02744287 , carcinoembryonic antigen positive (CEA+) for liver metastases ( {"type":"clinical-trial","attrs":{"text":"NCT03818165","term_id":"NCT03818165"}} NCT03818165 ), and CD133 ( {"type":"clinical-trial","attrs":{"text":"NCT02541370","term_id":"NCT02541370"}} NCT02541370 ). Moreover, new potentially promising combination therapies are ongoing targeting the tumor cell-associated antigen Nectin 4 and FAP present in CAFs in patients with Nectin4-positive/FAP-positive advanced solid tumors ( {"type":"clinical-trial","attrs":{"text":"NCT03932565","term_id":"NCT03932565"}} NCT03932565 ).
7.5. Other Immune Regulators
Preclinical studies revealed that colony-stimulating factor-1 (CSF1) and receptor (CSF1R) blockade not only decreases the number of the immunosuppressive TAMs, but also reprograms the remaining ones to support antigen presentation and bolster T cell activation within the tumor microenvironment ( Figure 3 ) [ 188 ]. Based on this strong rationale, a trial assessing a CSF1R inhibitor, ARRY-382, in combination with pembrolizumab in patients with advanced solid tumors has been finalized and the results are pending ( {"type":"clinical-trial","attrs":{"text":"NCT02880371","term_id":"NCT02880371"}} NCT02880371 ). Given the role of CSF1/CSF1R blockade in TAM reprogramming, this therapeutic option may be successful in combination therapies.
CXCR-4 is an alpha-chemokine receptor specific for stromal-derived-factor-1 which has been found to cause immune suppression and promote PCa [ 189 ]. The combination of CXCR4 antagonist, BL-8040, with pembrolizumab in patients with metastatic PCa, is currently being assessed ( {"type":"clinical-trial","attrs":{"text":"NCT02907099","term_id":"NCT02907099"}} NCT02907099 and {"type":"clinical-trial","attrs":{"text":"NCT02826486","term_id":"NCT02826486"}} NCT02826486 ). Moreover, more trials are underway with plerixafor (CXCR4 antagonist), in combination with cemiplimab (anti-PD-1) in metastatic PCa patients ( {"type":"clinical-trial","attrs":{"text":"NCT04177810","term_id":"NCT04177810"}} NCT04177810 ).
Natural killer (NK) cells have the ability to target and destroy tumor cells without prior sensitization, via activation of NK cell-activating receptors against ligands present on target tumor cells, providing a promising tool in the field of cancer immunotherapy [ 190 ]. Currently, there is an ongoing trial with FT500, an induced pluripotent stem cell-derived NK cell product in combination with ICIs ( {"type":"clinical-trial","attrs":{"text":"NCT03841110","term_id":"NCT03841110"}} NCT03841110 ). Although it is early to say, one expected drawback of therapy with NK cells in PCa is their relatively short lifespan, which causes a reduction in in vivo persistence and therapeutic efficacy. Future combination studies will provide information on the possibility to add NK cells in patients’ treatment options.
8. The Road to Personalized Oncology
The ideal world of personalized oncology is that of a patient going to the clinic, having an image-guided percutaneous core needle biopsy, and subsequently screened to identify its mutational and transcriptional profiles, which would allow for the optimal design of a personalized treatment regimen. Although pancreatic tumors are considered generally “cold” or non-T cell inflamed, some tumor subtypes called immunogenic display significant immune infiltrate, antigen presentation, CD4 + , and CD8 + T cells and show upregulation of CTLA-4 and PD-1 suppressive pathways [ 191 ]. These results suggest that these patients may benefit from immunotherapy with checkpoint inhibitors.
With the emergence of new technologies such as whole genome and single-cell RNA sequencing, there are many ongoing trials assessing the genome and transcriptional profiling of patients with advanced pancreatic tumors to identify predictive biomarkers and new actionable targets for therapy. For example, GATA6 expression in tumors was found to be a robust biomarker in basal-like tumor subtypes having significantly lower levels compared to classical subtype tumors. Interestingly, the basal-like tumor subtypes were found in the metastatic PCa patients, suggesting that this subtype may be present in more advanced tumors. Interestingly, 20 potentially actionable somatic mutations were found in 30% of the patients assessed involving ARID1A ( n = 8%), BRAF ( n = 2%), CDK4/6 ( n = 7%), PIK3CA ( n = 7%), PTEN ( n = 5%), and RNF43 ( n = 3%), giving rise to possibilities for personalized treatment for some of these patients [ 192 ].
The major limit to the success of personalized oncology for PCa patients is the lack of time from biopsy to treatment due to the fast disease progression that gives only a little time for personalized screening strategies. Moreover, the tumor site differences and the high intratumoral heterogeneity of PCa suggest that an exact treatment protocol may not be possible. There are many actionable targets currently under development (summarized in Table 3 ), but more rationalized and controlled clinical trials are needed. For instance, many studies described in this review were performed with a very low number of patients and/or lack of adequate controls within the same population. Moreover, there are many safe and potentially promising targeted therapies, yet some studies did not screen the patients for expression of specific targets before treatment, making difficult any interpretation. Since not all patients are positive for a specific tumor associated antigen, every clinical trial should first screen the target population for patients that are destined to respond (i.e mesothelin-high vs low) in order to obtain meaningful results. We hope that in the near future, biopsy-based genomic, transcriptomic, and immune landscape analysis for all PCa patients will become the standard of care.
Summary of mechanisms of oncological treatment.
9. Conclusions
Pancreatic ductal adenocarcinoma is a critical and increasing global health concern, hence it is highly essential to improve the effectiveness of the currently available therapeutic options. The complexity and the signaling pathway redundancy of RAS have reduced the successful targeting of RAS-mediated oncogene dependence at the clinical level [ 193 ]. Thus, combination therapies are the only option to treat RAS-mutant tumors. Recent experimental evidence made significant progress towards a better understanding of the unique characteristics of PCa progression and in relation to its surrounding microenvironment. From past successes and failures, we learned that an effective therapeutic strategy depends on the timing of its application. This model suggests that tumors should “ask” for a specific intervention and the timing of the addition of a compound may be determinant for the efficacy of a combination therapy.
Acknowledgments
The graphical abstract has been created with BioRender.com.
Author Contributions
C.L. and G.K. wrote the review and prepared the figures. All authors have read and agreed to the published version of the manuscript.
Funding was provided by the Swiss National Science Foundation (SNSF) Professorship #PP00P3_194810.
Conflicts of Interest
The authors declare no conflict of interest.
Publisher’s Note: MDPI stays neutral with regard to jurisdictional claims in published maps and institutional affiliations.

IMAGES
VIDEO
COMMENTS
Functions and regulation of Ron receptor tyrosine kinase in human pancreatic cancer and its therapeutic applications, Yi Zou. Theses/Dissertations from 2011 PDF. Coordinate detection of new targets and small molecules for cancer therapy, Kurt Fisher. PDF. The role of c-Myc in pancreatic cancer initiation and progression, Wan-Chi Lin. PDF
Cancer is a global health problem responsible for one in six deaths worldwide. Treating cancer has been a highly complex process. Conventional treatment approaches, such as surgery, chemotherapy, and radiotherapy, have been in use, while significant advances are being made in recent times, including stem cell therapy, targeted therapy, ablation therapy, nanoparticles, natural antioxidants ...
Advances in cancer therapy have prompted scientists to look for natural tumoricidal chemicals, such as antimicrobial peptides (AMPs), that could be used for cancer treatment. 202, 203, 204 In addition to antibacterial action, several AMPs have been shown to have intrinsic anticancer potential. 205, 206 These molecules constitute an intriguing ...
Extracellular vesicles for cancer diagnosis and therapy. EVs are classified in two categories based on their biogenesis. Specifically, exosomes are small vesicles of around 30-150 nm originated from endosomes in physiological and pathological conditions and released by a fusion of multivesicular bodies (MVBs) to the cell membrane [55, 56], while shed microvesicles (sMVs), with a typical size ...
The advent of nanotechnology has revolutionized the arena of cancer diagnosis and treatment. Nanoparticles (1-100 nm) can be used to treat cancer due to their specific advantages such as biocompatibility, reduced toxicity, more excellent stability, enhanced permeability and retention effect, and precise targeting.
In the United States, 13% of women are diagnosed with invasive breast cancer in their lifetime. and 6% of breast cancer patients have metastatic disease at initial diagnosis [1]. Moreover, nearly. 30% of women with early stage breast cancer will develop metastatic disease [2]. About 42,000.
Extensive research is underway to develop new therapeutic strategies to counteract therapy resistance in cancers. This review presents various strategies to achieve this objective. First, we discuss different vectorization platforms capable of releasing drugs in cancer cells. Second, we delve into multitarget therapies using drug combinations and dual anticancer agents. This section will ...
Abstract. Peptides exhibit lower affinity and a shorter half-life in the body than antibodies. Conversely, peptides demonstrate higher efficiency in tissue penetration and cell internalization ...
Department of Pharmaceutical Science Thesis Investigator: Radhika Narayanaswamy 3 TARGETED NANOPREPARATIONS FOR CANCER THERAPY STATEMENT OF HYPOTHESIS ... Cancer therapy in the recent years has evolved with the development of novel targeted drug delivery systems. The conventional chemotherapy approach is plagued by side effects and numerous
Cabozantinib targeting these receptor tyrosine kinases (RTKs) poses a promising therapeutic strategy for improving breast cancer treatment. However, clinical experience with Cabozantinib is very limited in metastatic breast cancer. In addition, bone lesions are a prominent challenge for treatment of MBC. Nearly 75% of patients with HR+ MBC ...
The advanced cancer therapy options include immunotherapy, which is used to manipulate the immune system so as to eliminate tumorous cells (Palucka and Banchereau, 2012; Jiang et al., 2012; Abbas and Rehman, 2018). In this chapter, cancer prevalence, types, available cancer therapeutic approaches, and their adverse side effects are discussed in ...
Introduction. Therapy with curative intent for breast cancer achieves optimal outcomes when it is administered to completion within a defined timeframe; its success depends on appropriate referrals for timely and personalized multimodality treatment after the receipt of a definitive diagnosis. 1 The provision of cancer treatment requires an organized, multidisciplinary approach in which ...
Tumor hypoxia has been a concern for cancer therapy 1,2.Hypoxia can increase tumor resistance to chemotherapy, radiation and lead to malignant progression and even metastasis 3,4.Hypoxia or some ...
Every sixth person in the world dies due to cancer, making it the second leading severe cause of death after cardiovascular diseases. According to WHO, cancer claimed nearly 10 million deaths in 2020. The most common types of cancers reported have been breast (lung, colon and rectum, prostate cases), skin (non-melanoma) and stomach. In addition to surgery, the most widely used traditional ...
Thermal therapy is a highly effective treatment for cancer because cancer cells are vulnerable to heat, and thermal therapy has been studied for a long time because of its minimal adverse effects ...
A novel protein-targeted near infrared photosensitizer was successfully prepared for highly efficient cancer photodynamic therapy. Under irradiated with near infrared laser, the reactive oxygen species produced by the photosensitizer directly destroys the intracellular biologically active proteins, causing cell dysfunction and finally inducing apoptosis.
Introduction. Radioactive iodine (131 I) has been used as an adjuvant treatment for high-risk, well-differentiated thyroid cancer after thyroidectomy for residual or recurrent thyroid cancer 1,2.Decreasing the use of 131 I for the treatment of low-risk thyroid cancer may be necessary owing to the controversial effects of this treatment on well-differentiated thyroid cancer 3,4.
Modern cancer treatment has evolved over several years to reach the current era . of precision therapy. Exciting developments in all modalities of cancer treatment and rapidly growing arena of translational research are contributing to the steady improvement in clinical outcomes. Although several old and new challenges have to
Modern chemotherapies offer a broad approach to cancer treatment but eliminate both cancer and non-cancer cells indiscriminately and, thus, are associated with a host of side effects. Advances in precision oncology have brought about new targeted therapeutics, albeit mostly limited to a subset of patients with an actionable mutation. They too come with side effects and, ultimately, 'self ...
This thesis project proposes a patient-centered environment designed to support the myriad and nuanced needs of the cancer patient, as well as a site-specific proposal for a comprehensive outpatient cancer treatment centre located in Spartanburg County, South Carolina. This cancer centre is designed with the notion of patient-empowerment as a ...
Depressive spectrum disorders are extremely common in cancer, although their prevalence varies considerably depending on the studies, the treatment settings (outpatient clinics, hospital and palliative care settings), the stage of cancer (early diagnosis, recurrence, survivorship or advanced stages) and the type of assessment methods and tools ...
CAR T-cell therapy: This is a promising type of adoptive cell therapy in which lymphocytes are changed to make a protein called chimeric antigen receptor (CAR) that—once injected back into the body—gives these cells the ability to specifically bind to cancer cells that have the target for CAR on their surface. For certain kinds of cell ...
The greatest challenge in cancer therapy is to eradicate cancer cells with minimal damage to normal cells. Targeted therapy has been developed to meet that challenge, showing a substantially ...
The U.S. health regulator on Wednesday approved the expanded use of Bristol Myers Squibb's cancer cell therapy Breyanzi for the treatment of adults with a type of blood cancer called follicular ...
Per the Cancer Research Institute, cancer immunotherapy "is a form of cancer treatment that uses the power of the body's own immune system to prevent, control, and eliminate cancer."
Mid-2024, Intensity Therapeutics will start Phase 3 and Phase 2/3 trials respectively in soft tissue sarcoma and triple-negative breast cancer. INT230-6 has shown up to 95% tumor necrosis ...
Merck said on Monday it had discontinued an experimental combination treatment testing a new type of immunotherapy in patients with a severe form of skin cancer after side effects led to high ...
This study was part of a PhD thesis entitled development and psychometric evaluation of motivation for healing questionnaire in cancer patients that was conducted from November 2019 to November 2020 in the Kashan University of Medical Sciences, Iran. ... The present study showed that trust in the treatment could promote optimism in cancer ...
Munn's cancer journey had a surprising start. She took a genetic test that returned negative for the breast cancer gene in April 2023 — as well as clear mammograms and an ultrasound.
Moreover, it was subsequently shown that treatment with SVN-2B triggered a dense infiltration of CD8 + T cells in some patient lesions and a high rate of programmed cell death ligand 1 (PD-L1) expression in cancer cells, indicating emergence of resistance against CTL attack . This clearly provides a rationale for the combination of SVN-2B with ...