133 Biodiversity Topics & Examples
🔝 top-10 biodiversity topics for presentation, 🏆 best biodiversity project topics, 💡 most interesting biodiversity assignment topics, 📌 simple & easy biodiversity related topics, 👍 good biodiversity title ideas, ❓ biodiversity research topics.
- Biodiversity loss.
- Global biodiversity conservation.
- The Amazon rainforest.
- Animal ecology research.
- Sub Saharan Africa.
- Marine biodiversity.
- Threats to ecosystems.
- Plant ecology.
- Importance of environmental conservation.
- Evolution of animal species.
- Biodiversity Hotspots: The Philippines The International Conservation has classified the Philippines as one of the biodiversity hotspots in the world. Additionally, the country is said to be one of the areas that are endangered in the world.
- Biodiversity Benefits for Ecology This variation of species in the ecosystem is a very important concept and factor that indeed is the basis for sustaining life on our planet. Moreover, the most important supporter of life, which is soil […]
- Aspects, Importance and Issues of Biodiversity Genetic diversity is a term used to refer to the dissimilitude of organisms of the same species. Species diversity is used to refer to dissimilitude of organisms in a given region.
- Loss of Biodiversity and Extinctions It is estimated that the number of species that have become extinct is greater than the number of species that are currently found on earth.
- Climate Change’s Negative Impact on Biodiversity This essay’s primary objective is to trace and evaluate the impact of climate change on biological diversity through the lens of transformations in the marine and forest ecosystems and evaluation of the agricultural sector both […]
- Habitat Destruction and Biodiversity Extinctions The instance of extinction is by and large regarded as the demise of the very last character of the genus. Habitat obliteration has played a major part in wiping out of species, and it is […]
- Biodiversity Conservation: Tropical Rainforest The forest is not a threat to many species and that, therefore, helps in showing that conserving this forest will be of great benefit to many species. The disadvantage of conserving the Mangrove Forest is […]
- When Human Diet Costs Too Much: Biodiversity as the Ultimate Answer to the Global Problems Because of the unreasonable use of the natural resources, environmental pollution and inadequate protection, people have led a number of species to extinction; moreover, due to the increasing rates of consumerist approach towards the food […]
- Biology Lab Report: Biodiversity Study of Lichens As a consequence of these results, the variety of foods found in forest flora that include lichens may be linked to varying optimum conditions for establishment and development.
- The Importance of Biodiversity in Ecosystem The most urgent problem right now is to maintain the level of biodiversity in this world but it has to begin with a more in-depth understanding of how different species of flora and fauna can […]
- How Human Health Depends on Biodiversity The disturbance of the ecosystem has some effects on the dynamics of vectors and infectious diseases. Change of climate is a contributing factor in the emergence of new species and infectious diseases.
- Biodiversity: Aspects Within the Sphere of Biology Finally, living objects consist of cells, which are the basic units of their function and structure. The viruses’ structure depends on which nucleic acid is included, which denotes that there are DNA and RNA viruses.
- Coral Reef and Biodiversity in Ecosystems Coral reefs are formed only in the tropical zone of the ocean; the temperature limits their life – are from +18 to +29oS, and at the slightest deviation from the boundaries of the coral die.
- Biodiversity and the Health of Ecosystems Various opinions are revealed concerning biodiversity, including the human impact, reversal of biodiversity loss, the impact of overpopulation, the future of biodiversity, and the rate of extinction.
- Wild Crops and Biodiversity Threats However, out of millions of existing types of wild crop cultures, the vast majority have been abandoned and eradicated, as the agricultural companies placed major emphasis on the breeding of domesticated cultures that are easy […]
- Biodiversity, Interdependency: Threatened and Endhangered Species In the above table, humans rely on bees to facilitate pollination among food crops and use their honey as food. Concurrently, lichens break down rocks to provide nutrient-rich soil in the relationship.
- Invasive Processes’ Impact on Ecosystem’s Biodiversity If the invasive ones prove to be more adaptive, this will bring about the oppression of the native species and radical changes in the ecosystem.
- Conserving Biodiversity: The Loggerhead Turtle The loggerhead sea turtle is the species of oceanic turtle which is spread all over the world and belongs to the Cheloniidae family.
- Biodiversity and Dynamics of Mountainous Area Near the House It should be emphasized that the term ecosystem used in this paper is considered a natural community characterized by a constant cycle of energy and resources, the presence of consumers, producers, and decomposers, as well […]
- National Biodiversity Strategy By this decision, the UN seeks to draw the attention of the world community and the leaders of all countries to the protection and rational use of natural resources.
- Rewilding Our Cities: Beauty, Biodiversity and the Biophilic Cities Movement What is the source of your news item? The Guardian.
- Biodiversity and Food Production This paper will analyze the importance of biodiversity in food production and the implications for human existence. Edible organisms are few as compared to the total number of organisms in the ecosystem.
- Restoring the Everglades Wetlands: Biodiversity The Act lays out the functions and roles of the Department of Environmental Protection and the South Florida Water Management District in restoration of the Everglades.
- Biodiversity: Importance and Benefits This is due to the fact that man is evolving from the tendency of valuing long term benefits to a tendency of valuing short terms benefits.
- A Benchmarking Biodiversity Survey of the Inter-Tidal Zone at Goat Island Bay, Leigh Marine Laboratory Within each quadrant, the common species were counted or, in the case of seaweed and moss, proliferation estimated as a percentage of the quadrant occupied.
- Plant Interactions and Biodiversity: Ecological Insights The author is an ecologist whose main area of interest is in the field of biodiversity and composition of the ecosystem.
- Biodiversity: Population Versus Ecosystem Diversity by David Tilman How is the variability of the plant species year to year in the community biomass? What is the rate of the plant productivity in the ecosystem?
- Biodiversity Hotspots and Environmental Ethics The magnitude of the problem of losing biodiversity hotspots is too great, to the extend of extinction of various species from the face of the earth.
- Natural Selection and Biodiversity These are featured by the ways in which the inhabiting organisms adapt to them and it is the existence of these organisms on which the ecosystems depend and therefore it is evident that this diversity […]
- Scientific Taxonomy and Earth’s Biodiversity A duck is a domestic bird that is reared for food in most parts of the world. It is associated with food in the household and is smaller than a bee.
- Global Warming: Causes and Impact on Health, Environment and the Biodiversity Global warming is defined in simple terms as the increase in the average temperature of the Earth’s surface including the air and oceans in recent decades and if the causes of global warming are not […]
- Loss of Biodiversity in the Amazon Ecosystem The growth of the human population and the expansion of global economies have contributed to the significant loss of biodiversity despite the initial belief that the increase of resources can halt the adverse consequences of […]
- California’s Coastal Biodiversity Initiative The considered threat to California biodiversity is a relevant topic in the face of climate change. To prevent this outcome, it is necessary to involve the competent authorities and plan a possible mode of operation […]
- Biodiversity: American Museum of Natural History While staying at the museum, I took a chance to visit the Milstein Family Hall of Ocean Life and the Hall of Reptiles and Amphibians.
- Biodiversity and Animal Population in Micronesia This means that in the future, the people living in Micronesia will have to move to other parts of the world when their homes get submerged in the water.
- Urban Plants’ Role in Insects’ Biodiversity The plants provide food, shelter and promote the defensive mechanisms of the insects. The observation was also an instrumental method that was used to assess the behavior and the existence of insects in relation to […]
- Biodiversity Markets and Bolsa Floresta Program Environmentalists and scholars of the time led by Lord Monboddo put forward the significance of nature conservation which was followed by implementation of conservation policies in the British Indian forests.
- Brazilian Amazonia: Biodiversity and Deforestation Secondly, the mayor persuaded the people to stop deforestation to save the Amazon. Additionally, deforestation leads to displacement of indigenous people living in the Amazonia.
- Defining and Measuring Biodiversity Biodiversity is measured in terms of attributes that explore the quality of nature; richness and evenness of the living organisms within an ecological niche.
- Biodiversity, Its Importance and Benefits Apart from that, the paper is going to speculate on the most and least diverse species in the local area. The biodiversity can be measured in terms of the number of different species in the […]
- Biodiversity, Its Evolutionary and Genetic Reasons The occurrence of natural selection is hinged on the hypothesis that offspring inherit their characteristics from their parents in the form of genes and that members of any particular population must have some inconsiderable disparity […]
- Biodiversity Hotspots: Evaluation and Analysis The region also boasts with the endangered freshwater turtle species, which are under a threat of extinction due to over-harvesting and destroyed habitat.
- Marine Biodiversity Conservation and Impure Public Goods The fact that the issue concerning the global marine biodiversity and the effects that impure public goods may possibly have on these rates can lead to the development of a range of externalities that should […]
- Natural Sciences: Biodiversity and Human Civilisation The author in conjunction with a team of other researchers used a modelling study to illustrate the fact approximately 2 percent of global energy is currently being deployed in the generation of wind and solar […]
- How Biodiversity Is Threatened by Human Activity Most of the marine biodiversity is found in the tropics, especially coral reefs that support the growth of organisms. Overexploitation in the oceans is caused by overfishing and fishing practices that cause destruction of biodiversity.
- Biodiversity and Business Risk In conclusion, biodiversity risk affects businesses since the loss of biodiversity leads to: coastal flooding, desertification and food insecurity, all of which have impacts on business organizations.
- Measurement of Biodiversity It is the “sum total of all biotic variation from the level of genes to ecosystems” according to Andy Purvus and Andy Hector in their article entitled “Getting the Measure of Diversity” which appeared in […]
- Introduced Species and Biodiversity Rhymer and Simberloff explain that the seriousness of the phenomenon may not be very evident from direct observation of the morphological traits of the species.
- Ecosystems: Biodiversity and Habitat Loss The review of the topic shows that the relationship between urban developmental patterns and the dynamics of ecosystem are concepts that are still not clearly understood in the scholarly world as well as in general.
- The Impact of Burmese Pythons on Florida’s Native Biodiversity Scientists from the South Florida Natural Resource Center, the Smithsonian institute and the University of Florida have undertaken studies to assess the predation behavior of the Burmese pythons on birds in the area.
- Essentials of Biodiversity At the same time, the knowledge and a more informed understanding of the whole concept of biodiversity gives us the power to intervene in the event that we are faced by the loss of biodiversity, […]
- Threat to Biodiversity Is Just as Important as Climate Change This paper shall articulate the truth of this statement by demonstrating that threats to biodiversity pose significant threat to the sustainability of human life on earth and are therefore the protection of biodiversity is as […]
- Cold Water Coral Ecosystems and Their Biodiversity: A Review of Their Economic and Social Value
- Benchmarking DNA Metabarcoding for Biodiversity-Based Monitoring and Assessment
- Prospects for Integrating Disturbances, Biodiversity and Ecosystem Functioning Using Microbial Systems
- Enterprising Nature: Economics, Markets, and Finance in Global Biodiversity Politics
- Institutional Economics and the Behaviour of Conservation Organizations: Implications for Biodiversity Conservation
- Fisheries, Fish Pollution and Biodiversity: Choice Experiments With Fishermen, Traders and Consumers
- Last Stand: Protected Areas and the Defense of Tropical Biodiversity
- Hardwiring Green: How Banks Account For Biodiversity Risks and Opportunities
- Governance Criteria for Effective Transboundary Biodiversity Conservation
- Marine Important Bird and Biodiversity Areas for Penguins in Antarctica: Targets for Conservation Action
- Ecological and Economic Assessment of Forests Biodiversity: Formation of Theoretical and Methodological Instruments
- Environment and Biodiversity Impacts of Organic and Conventional Agriculture
- Food From the Water: How the Fish Production Revolution Affects Aquatic Biodiversity and Food Security
- Biodiversity and World Food Security: Nourishing the Planet and Its People
- Climate Change and Energy Economics: Key Indicators and Approaches to Measuring Biodiversity
- Conflicts Between Biodiversity and Carbon Sequestration Programs: Economic and Legal Implications
- Models for Sample Selection Bias in Contingent Valuation: Application to Forest Biodiversity
- Optimal Land Conversion and Growth With Uncertain Biodiversity Costs
- Internalizing Global Externalities From Biodiversity: Protected Areas and Multilateral Mechanisms of Transfer
- Combining Internal and External Motivations in Multi-Actor Governance Arrangements for Biodiversity and Ecosystem Services
- Balancing State and Volunteer Investment in Biodiversity Monitoring for the Implementation of CBD Indicators
- Differences and Similarities Between Ecological and Economic Models for Biodiversity Conservation
- Globalization and the Connection of Remote Communities: Household Effects and Their Biodiversity Implications
- Shaded Coffee and Cocoa – Double Dividend for Biodiversity and Small-Scale Farmers
- Spatial Priorities for Marine Biodiversity Conservation in the Coral Triangle
- One World, One Experiment: Addressing the Biodiversity and Economics Conflict
- Alternative Targets and Economic Efficiency of Selecting Protected Areas for Biodiversity Conservation in Boreal Forest
- Analysing Multi Level Water and Biodiversity Governance in Their Context
- Agricultural Biotechnology: Productivity, Biodiversity, and Intellectual Property Rights
- Renewable Energy and Biodiversity: Implications for Transitioning to a Green Economy
- Agricultural Biodiversity and Ecosystem Services of Major Farming Systems
- Integrated Land Use Modelling of Agri-Environmental Measures to Maintain Biodiversity at Landscape Level
- Changing Business Perceptions Regarding Biodiversity: From Impact Mitigation Towards New Strategies and Practices
- Forest Biodiversity and Timber Extraction: An Analysis of the Interaction of Market and Non-market Mechanisms
- Poverty and Biodiversity: Measuring the Overlap of Human Poverty and the Biodiversity Hotspots
- Protecting Agro-Biodiversity by Promoting Rural Livelihoods
- Maintaining Biodiversity and Environmental Sustainability
- Landscape, Legal, and Biodiversity Threats That Windows Pose to Birds: A Review of an Important Conservation Issue
- Variable Mating Behaviors and the Maintenance of Tropical Biodiversity
- Species Preservation and Biodiversity Value: A Real Options Approach
- What Is Being Done to Preserve Biodiversity and Its Hotspots?
- How Are Argentina and Chile Facing Shared Biodiversity Loss?
- Are Diverse Ecosystems More Valuable?
- How Can Biodiversity Loss Be Prevented?
- Can Payments for Watershed Services Help Save Biodiversity?
- How Can Business Reduce Impacts on the World’s Biodiversity?
- Are National Biodiversity Strategies Appropriate for Building Responsibilities for Mainstreaming Biodiversity Across Policy Sectors?
- How Does Agriculture Effect Biodiversity?
- Are There Income Effects on Global Willingness to Pay For Biodiversity Conservation?
- How Does the Economic Risk Aversion Affect Biodiversity?
- What Are the Threats of Biodiversity?
- How Has the Increased Usage of Synthetic Pesticides Impacted Biodiversity?
- What Does Drive Biodiversity Conservation Effort in the Developing World?
- How Does the Plantation Affect Biodiversity?
- What Does Drive Long-Run Biodiversity Change?
- How Does the United Nations Deal With Biodiversity?
- What Factors Affect Biodiversity?
- How Are Timber Harvesting and Biodiversity Managed in Uneven-Aged Forests?
- When Should Biodiversity Tenders Contract on Outcomes?
- Who Cares About Biodiversity?
- Why Can Financial Incentives Destroy Economically Valuable Biodiversity in Ethiopia?
- What Factors Affect an Area’s Biodiversity?
- In What Ways Is Biodiversity Economically Valuable?
- Which Human Activities Threaten Biodiversity?
- How Can Biodiversity Be Protected?
- In What Ways Is Biodiversity Ecologically Value?
- In Which Countries Is Biodiversity Economically Valuable?
- Does Species Diversity Follow Any Patterns?
- How Is Biodiversity Measured?
- What Is a Biodiversity Hotspot?
- Ecosystem Essay Topics
- Climate Change Titles
- Environment Research Topics
- Disaster Essay Titles
- Evolution Topics
- Glaciers Topics
- Hunting Questions
- Global Warming Essay Titles
- Chicago (A-D)
- Chicago (N-B)
IvyPanda. (2024, February 22). 133 Biodiversity Topics & Examples. https://ivypanda.com/essays/topic/biodiversity-essay-topics/
"133 Biodiversity Topics & Examples." IvyPanda , 22 Feb. 2024, ivypanda.com/essays/topic/biodiversity-essay-topics/.
IvyPanda . (2024) '133 Biodiversity Topics & Examples'. 22 February.
IvyPanda . 2024. "133 Biodiversity Topics & Examples." February 22, 2024. https://ivypanda.com/essays/topic/biodiversity-essay-topics/.
1. IvyPanda . "133 Biodiversity Topics & Examples." February 22, 2024. https://ivypanda.com/essays/topic/biodiversity-essay-topics/.
Bibliography
IvyPanda . "133 Biodiversity Topics & Examples." February 22, 2024. https://ivypanda.com/essays/topic/biodiversity-essay-topics/.

Biodiversity Loss, the Motivation Problem, and the Future of Conservation Education in the United States
PDF Version Also Available for Download.
Description
The purpose of this dissertation is to make sense of two sets of reactions. On the one hand, Americans can barely lift a finger to help threatened and endangered species while on the other, they routinely come to the aid of human victims of disaster. I argue that in contrast to cases of human tragedy, for the biodiversity crisis conservationists are faced not only with the familiar yet arduous task of motivating the American public to care for living other-than-humans, but they are also saddled with having to overcome the motivation problem of future ethics. The motivation problem consists in … continued below
Creation Information
Grove-Fanning, William December 2011.
This dissertation is part of the collection entitled: UNT Theses and Dissertations and was provided by the UNT Libraries to the UNT Digital Library , a digital repository hosted by the UNT Libraries . It has been viewed 2083 times, with 17 in the last month. More information about this dissertation can be viewed below.
People and organizations associated with either the creation of this dissertation or its content.
- Grove-Fanning, William
- Callicott, J. Baird Major Professor
Committee Members
- Hargrove, Eugene C., 1944-
- Rozzi, Ricardo
- University of North Texas Publisher Info: www.unt.edu Place of Publication: Denton, Texas
Rights Holder
For guidance see Citations, Rights, Re-Use .
Provided By
Unt libraries.
The UNT Libraries serve the university and community by providing access to physical and online collections, fostering information literacy, supporting academic research, and much, much more.
Descriptive information to help identify this dissertation. Follow the links below to find similar items on the Digital Library.
Degree Information
- Department: Department of Philosophy and Religion Studies
- Discipline: Philosophy
- Level: Doctoral
- Name: Doctor of Philosophy
- PublicationType: Doctoral Dissertation
- Grantor: University of North Texas
The purpose of this dissertation is to make sense of two sets of reactions. On the one hand, Americans can barely lift a finger to help threatened and endangered species while on the other, they routinely come to the aid of human victims of disaster. I argue that in contrast to cases of human tragedy, for the biodiversity crisis conservationists are faced not only with the familiar yet arduous task of motivating the American public to care for living other-than-humans, but they are also saddled with having to overcome the motivation problem of future ethics. The motivation problem consists in eliminating or bridging a motivational gap that lies between knowledge of the effects of our actions on future generations and action taken based upon such knowledge. The gap exists because motives that typically move people to action are either ineffective or unavailable. What is more, the gap influences not only our ability to care for future humans, but it affects our ability to care for future other-than-humans as well. Biodiversity loss is in fact a subset of the problem of future generations, an identification hitherto little appreciated. I argue that conservationists can overcome the motivational gap not by appealing directly to the value of species or biodiversity, both of which are temporally distant, abstract and general moral patients, but indirectly, by focusing on the concrete and particular lives of extant and near future moral patients. By applying techniques that have been developed to overcome the motivation problem as it pertains to distant future human generations, conservationists have additional resources to draw upon in their efforts to motivate American citizens to preserve biodiversity. This dissertation’s contribution to the fields of environmental philosophy and conservation biology is both theoretical and practical. It is theoretically significant to elucidate the nature of moral failure for biodiversity conservation. In terms of broader impacts, identifying the basis of moral failure for biodiversity conservation allows me to assess educational campaigns and environmental policy, and to suggest solutions for bridging the motivational gap.
- Future generations
- biodiversity
- environmental education
- moral motivation
- Thesis or Dissertation
Unique identifying numbers for this dissertation in the Digital Library or other systems.
- OCLC : 815395645
- Archival Resource Key : ark:/67531/metadc103321
Collections
This dissertation is part of the following collection of related materials.
UNT Theses and Dissertations
Theses and dissertations represent a wealth of scholarly and artistic content created by masters and doctoral students in the degree-seeking process. Some ETDs in this collection are restricted to use by the UNT community .
What responsibilities do I have when using this dissertation?
Digital Files
- 104 image files available in multiple sizes
- 1 file (.pdf)
- Metadata API: descriptive and downloadable metadata available in other formats
Dates and time periods associated with this dissertation.
Creation Date
- December 2011
Added to The UNT Digital Library
- Oct. 2, 2012, 4:18 p.m.
Description Last Updated
- Aug. 10, 2020, 12:59 p.m.
Usage Statistics
When was this dissertation last used?
Interact With This Dissertation
Here are some suggestions for what to do next.
Search Inside
- or search this site for other thesis or dissertations
Start Reading
- All Formats
Citations, Rights, Re-Use
- Citing this Dissertation
- Responsibilities of Use
- Licensing and Permissions
- Linking and Embedding
- Copies and Reproductions
International Image Interoperability Framework

We support the IIIF Presentation API
Print / Share
Links for robots.
Helpful links in machine-readable formats.
Archival Resource Key (ARK)
- ERC Record: /ark:/67531/metadc103321/?
- Persistence Statement: /ark:/67531/metadc103321/??
International Image Interoperability Framework (IIIF)
- IIIF Manifest: /ark:/67531/metadc103321/manifest/
Metadata Formats
- UNTL Format: /ark:/67531/metadc103321/metadata.untl.xml
- DC RDF: /ark:/67531/metadc103321/metadata.dc.rdf
- DC XML: /ark:/67531/metadc103321/metadata.dc.xml
- OAI_DC : /oai/?verb=GetRecord&metadataPrefix=oai_dc&identifier=info:ark/67531/metadc103321
- METS : /ark:/67531/metadc103321/metadata.mets.xml
- OpenSearch Document: /ark:/67531/metadc103321/opensearch.xml
- Thumbnail: /ark:/67531/metadc103321/thumbnail/
- Small Image: /ark:/67531/metadc103321/small/
- In-text: /ark:/67531/metadc103321/urls.txt
- Usage Stats: /stats/stats.json?ark=ark:/67531/metadc103321
Grove-Fanning, William. Biodiversity Loss, the Motivation Problem, and the Future of Conservation Education in the United States , dissertation , December 2011; Denton, Texas . ( https://digital.library.unt.edu/ark:/67531/metadc103321/ : accessed June 1, 2024 ), University of North Texas Libraries, UNT Digital Library, https://digital.library.unt.edu ; .
Advertisement
School Students’ Conceptions about Biodiversity Loss: Definitions, Reasons, Results and Solutions
- Published: 25 April 2013
- Volume 43 , pages 2277–2307, ( 2013 )
Cite this article
- Ahmet Kilinc 1 ,
- Namik Kemal Yeşiltaş 2 ,
- Tezcan Kartal 3 ,
- Ümit Demiral 3 &
- Baris Eroğlu 4
3111 Accesses
24 Citations
Explore all metrics
Environmental degradation stemming from anthropocentric causes threatens the biodiversity more than ever before, leading scholars to warn governments about the impending consequences of biodiversity loss (BL). At this point, it is of great importance to study the public’s conceptions of BL in order to identify significant educational implications. However, a review of the literature reveals a relatively small body of research about the public understanding of BL. In this qualitative study, we thus strived to elicit Turkish school students’ conceptions about BL using a written questionnaire including open-ended questions with respect to the definition of biodiversity as well as reasons for, results of and solutions to BL. The sample consisted of 245 school students in a relatively small city. A two-staged content analysis was run on the responses. The results showed that school students most commonly preferred species-focused definitions of biodiversity and understood BL through such various conceptual patterns as, ‘balance of nature’, ‘forest’, ‘global warming’, ‘hunting’ and ‘indirect conservation’. At the end of the paper, the possible educational implications and future perspectives were discussed.
This is a preview of subscription content, log in via an institution to check access.
Access this article
Price includes VAT (Russian Federation)
Instant access to the full article PDF.
Rent this article via DeepDyve
Institutional subscriptions
Similar content being viewed by others
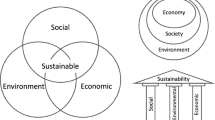
Three pillars of sustainability: in search of conceptual origins
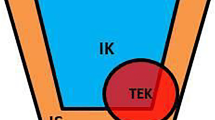
A Multi-Perspective Reflection on How Indigenous Knowledge and Related Ideas Can Improve Science Education for Sustainability
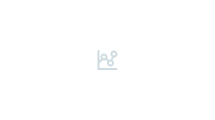
Anthropocentrism: More than Just a Misunderstood Problem
Ashworth, S., Boyes, E., Paton, R., & Stanisstreet, M. (1995). Conservation of endangered species; what do children think? International Journal of Environmental Education and Information, 14 , 229–244.
Google Scholar
Barman, C. R., Griffiths, A. K., & Okebukola, P. A. O. (1995). An analysis of high school students’ concepts regarding food chains and food webs. The American Biology Teacher, 56 , 160–163.
Article Google Scholar
Basca, B.B., Grotzer, T.A., Donis, K., & Shaw, S. (2000, April). Using domino and relational causality to analyse ecosystems: realizing what goes around comes around. Paper presented at the National Association of Research in Science Teaching, New Orleans, NO.
Bloom, J.W. (2001, March). Chaos, complexity, and metapatterns in discourse and learning: A perspective on developing complex understandings. Paper presented at the annual meeting of the National Association for Research in Science Teaching, St. Louis.
Bostrom, A., Morgan, M. G., Fischhoff, B., & Read, D. (1994). What do people know about global climate change? 1 Mental models. Risk Analysis, 14 (6), 959–970.
Boyes, E., & Stanisstreet, M. (1997). Children's models of understanding of two major global environmental issues (ozone layer and greenhouse effect). Research in Science and Technological Education, 15 (1), 19–28.
Carey, S. (1999). Sources of conceptual change. In E. K. Scholnick, K. Nelson, & P. Miller (Eds.), Conceptual development: Piaget’s legacy (pp. 293–326). Mahwah, NJ: Lawrence Erlbaum Associates.
Collins, M. A. J. (1976). Students’ attitudes towards animals. The American Biology Teacher, 38 (8), 491–493.
Cresswell, J.W. (2008) Educational research: planning, conducting, and evaluating quantitative and qualitative research (third edition) New Jersey: Pearson Education Ltd.
Dawe, G., Jucker, R., & Martin, S. (2005). Sustainable development in higher education: Current practices and future developments. Retrieved on January 3, 2010, from http:// www.heacademy.ac.uk/assets/York
Dawson, V. (2007). An exploration of high school students understanding of and attitudes towards biotechnology processes. Research in Science Education, 37 , 59–73.
Demirsoy, A. (2000). Doğal hayatın korunması ve sürüdürülebilir yönetimi (Protection and sustainable management of the natural life). Paper presented at 4th Çevre Şurası, Çevre Bakanlığı, İzmir.
Dervişoglu, S. (2007). Learning pre-conditions for protecting the biodiversity. (Unpublished doctoral dissertation) Hacettepe University, Ankara.
Driver, R., Guesne, E., & Tiberghien, A. (1985). Children’s ideas and the learning of science. In. R., Driver, E., Guesne, & A. Tiberghien (Eds), Children’s ideas in science. Milton Keynes, Philedelphia: Open Univrsity press.
diSessa, A. A., & Sherin, B. L. (1998). What changes in conceptual change. International Journal of Science Education, 20 (10), 1155–1191.
Driscoll, M.P. (2000). Psychology of learning for instruction. Massachusetts: Pearson Education Ltd.
Duit, R., & Treagust, D. F. (2003). Conceptual change: a powerful framework for improving science teaching and learning. International Journal of Science Education, 25 (6), 671–688.
Erdoğan, M., Erentay, N., Barss, M., & Nachita, A. (2008). Students’ awareness of endangered species and threatened environments: a comparative case study. International Journal of Hands-on Science, 1 (2), 46–53.
Eroglu B., Kilinc, A., Boyes, E., & Stanisstreet, M. (2010, September). Education to encourage actions to reduce global warming: which biological organisms might be used as motivators? Paper presented at 9th Turkish National Science and Mathematics Education Congress, Izmir, Turkey.
Eurobarometer (2009). Europeans’ attitudes towards climate change. Special Eurobarometer 313.
Eurobarometer (2007). Attitudes of Europeans towards the issues of biodiversity. Flash Eurobarometer Series No: 219. The Gallup Organization.
Fraenkel, J. R., & Wallen, N. E. (2003). How to design and evaluate research in education . New York: McGraw-Hill.
Francis, C., Boyes, E., Qualter, A., & Stanisstreet, M. (1993). Ideas of elementary students about reducing the ‘Greenhouse Effect’. Science Education, 77 , 375–392.
Gebhard, U., Nevers, P., & Billmann-Mahecha, E. (2003). Moralizing trees: anthropomorphism and identity in children relationships to nature. In S. Clayton & S. Opotow (Eds.), Identity and the natural environment: the psychological significance of nature, identity and the natural environment (pp. 91–112). Cambridge: MIT press.
Grace, M. (2009). Developing high quality decision making discussion about biological conservation in a normal classroom setting. International Journal of Science Education, 31 (4), 551–570.
Grace, M. M., & Ratcliffe, M. (2002). The science and values that the young people draw upon to make decisions about biological conservation issues. International Journal of Science Education, 24 (11), 1157–1169.
Greaves, E., Stanisstreet, M., Boyes, E., & Williams, T. (1993). Children’s ideas about rainforests. Journal of Biological Education, 27 (3), 189–194.
Grotzer, T., & Basca, B. B. (2003). How does grasping the underlying causal structures of ecosystems impact students’ understanding. Journal of Biological Education, 38 (1), 16–29.
Hellden, G., & Hellden, S. (2008). Students’ early experiences of biodiversity and education for a sustainable future. Nordina, 4 (2), 123–131.
Hunter, L. M., & Brehm, J. (2003). Brief comment: qualitative insight into public knowledge of, and concern with biodiversity. Human Ecology, 31 (2), 309–320.
Hunter, L. M., & Rinner, L. (2004). The association between environmental perspective and knowledge and concern with species diversity. Society and Natural Resources, 17 , 517–532.
Inglehart, R., & Welzel, C. (2005). Modernization, Cultural change, and Democracy. Retrieved on August 11, 2009, from http:// www.worldvaluesurvey.org .
IPPC (Intergovernmental Panel on Climate Change). (2001). Climate change 2001: The scientific basis . New York: Cambridge University Press.
Jeffries, H., Stanisstreet, M., & Boyes, E. (2001). Knowledge about the ‘Greenhouse Effect’: have college students improved? Research in Science & Technological Education, 19 (2), 205–221.
Jimenez, N.J. (2008). Children’s knowledge, perception and appreciation of nature and non-native plant and animal species in Valle Fertil, Argentina. (Unpublished master dissertation). Universitat Zurich, Zurich.
Kals, E., & Ittner, H. (2003). Children’s environmental identity: indicators and behavioral impacts. In S. Clayton & S. Opotow (Eds.), Identity and the natural environment: the psychological significance of nature (pp. 135–158). Cambridge: MIT press.
Kassas, M. (2002). Environmental education: biodiversity. Environmentalist, 22 , 345–351.
Kellert, S. R. (1985). Attitudes toward animals: age-related development among children. The Journal of Environmental Education, 16 , 29–39.
Kellert, S. R. (1996). The Value of life . Washington: Island Press.
Kilinc, A., Stanisstreet, M., & Boyes, E. (2008). Turkish students’ ideas about global warming. International Journal of Environmental & Science Education, 3 (2), 89–98.
Knight, A. J. (2008). ‘Baths, snakes, and spiders’ Oh my! How aesthetic and negativistic attitudes and other concepts predict support for species protection. Journal of Environmental Psychology, 28 , 94–103.
Kostova, A., & Atasoy, E. (2008). Comparative evaluation of the environmental culture of 8th grade students in Bulgaria and Turkey. Bulgarian Journal of Science and Education Policy, 2 , 25–47.
Kurumlu, M.S. (2008). Biyoçeşitliliğimizi koruyabiliyor muyuz: Önemi ve koruma stratejileri üzerine Biyoloji öğretmenlerinin yeterliliklerinin araştırılması (Are we able to conserve our biodiversity? A research on the proficiency of biology teachers over the importance and conservation strategies). (Unpublished master dissertation). Gazi University, Ankara.
Ladle, R. J., & Gillson, L. (2009). The imbalance of nature: a public perception time-lag? Public Understanding of Science, 18 , 229–242.
Lamanouskas, V., & Petkeviciene, R. M. (2008). Lithuainian university students’ knowledge of biotechnology and their attitudes to the taught subject. Eurasian Journal of Mathematics, Science and Technology Education, 4 (3), 269–277.
Leeds National Curriculum Science Support Project (LNCSSP) (1992). Children’s ideas about ecosystems. Research report.
Leiserowitz, A.A. (2003). Global warming in the American mind: the roles of affect, imagery, and worldviews in risk perception, policy preferences and behaviour. (Unpublished doctoral dissertation). University of Oregon, Eugene.
Lindemann-Matthies, P. (2005). ‘Loveable’ mammals and ‘lifeless’ plants: how children’s interest in common local organism can be enhanced through observation of nature. International Journal of Science Education, 27 (6), 655–677.
Lindemann-Matthies, P., & Bose, E. (2008). How many species are there? Public understanding and awareness of biodiversity in Switzerland. Human Ecology, 38 , 731–742.
Mankin, P. C., Warner, R. E., & Anderson, W. L. (1999). Wildlife and the Illinois public: a benchmark study of attitudes and perceptions. Wildlife Society Bulletin, 27 (2), 465–472.
McCloskey, M. (1983). Naive theories of motion. In D. Gentner & A. L. Stevens (Eds.), Mental models (pp. 299–324). Mahwah: Lawrence Erlbaum Associates.
Menzel, S., & Bögeholz, S. (2009). The loss of biodiversity as a challenge for sustainable development: how do pupils in Chile and Germany perceive resource dilemmas? Research in Science Education, 39 , 429–447.
Millennium Ecosystem Assesment. (2005). Ecosystems and human well-being: current state and trends . London: Izland Press.
Munson, B. (1994). Ecological misconceptions. The Journal of Environmental Education, 25 , 30–34.
Natarajan, C., Sugra, C., Apte, S., & Rehaodes, J. (2002). Lessons for teaching botany: what do middle school students know about plants? Paper presented at 10th International Organization for Science and Technology Education Symposium . Brazil: Parana.
Novacek, M. J. (2008). Engaging the public in biodiversity issues. Proceedings of the National Academy of Sciences of the United States of America, 105 , 11571–11578.
Palmer, D. H. (1997). Students’ application of the concept of interdependence to the issue of preservation of species: observations on the ability to generalize. Journal of Research in Science Teaching, 34 (8), 837–850.
Perkins, D. N., & Grotzer, T. A. (2005). Dimensions of causal understanding: the role of complex causal models in students’ understanding of science. Studies in Science Education, 41 , 117–166.
Pintrich, P. R., Marx, R. W., & Boyle, R. A. (1993). Beyond cold conceptual change: the role of motivational beliefs and classroom contextual factors in the process of conceptual change. Review of Educational Research, 63 (2), 167–199.
Prokop, P., Kubiatko, M., & Fancovicova, J. (2009). Slovakian pupils’ knowledge of and attitudes toward birds. Anthrozoös, 21 (3), 221–235.
Sjöberg, L. (2000a). Perceived risk and tampering with nature. Journal of Risk Research, 3 (4), 353–367.
Sjöberg, L. (2000b). Specifying factors in radiation risk perception. Scandinavian Journal of Psychology, 41 , 169–174.
Sjöberg, L., & Torell, G. (1993). The development of risk acceptance and moral valuation. Scandinavian Journal of Psychology 34:223–236.
Snaddon, J. L., Turner, E. D., & Foster, A. W. (2008). Childrens perceptions of rainforest biodiversity: which animals have the Lion`s share of environmental awareness. PLoS One, 3 (7), e2579.
Stamp, N. (2004). Misconceptions about plant–herbivore interactions, especially plant defenses. Bulletin of the Ecological Society of America, October, 201–205.
Stamp, N., Armstrong, M., & Biger, J. (2006). Ecological misconceptions, Survey III: The challenge of identifying sophisticated understanding. Bulletin of the Ecological Society of America, April, 168–175.
Stanisstreet, M., Spofford, N., & Williams, T. R. (1993). Attitudes of children to the uses of animals. International Journal of Science Education, 15 , 411–425.
Strike, K.A., & Posner, G.J. (1992). A revisionist theory of conceptual change. In R.A. Duschl & R.J. Hamilton (Eds.), Philosophy of science, cognitive psychology, and educational theory and practice (pp. 147–176). New York: State University of New York Press.
Türkiye Bilimler Akademisi (TÜBA) (2006). Dünya’da ve Türkiye’de biyolojik çeşitliliği koruma (Conserving biodiversity in Turkey and worldwide). Türkiye Bilimler Akademisi Raporları Sayı: 13, Ankara: Şenol Matbaacılık.
United Nations Environment Programme (UNEP). (1993). Guidelines for country studies on biodiversity . Nairobi: UNEP.
Uşak, M., Erdogan, M., Prokop, P., & Özel, M. (2009). High school and university students knowledge and attitudes regarding biotechnology. Biochemistry and Molecular Biology Education, 37 (2), 123–130.
Uzun, N., Özsoy, S., & Keleş, Ö. (2010). Öğretmen Adaylarının Biyolojik Çeşitlilik Kavramına Yönelik Görüşleri (The ideas of preservice teachers about the conception of biodiversity). Biyoloji Bilimleri Araştırma Dergisi, 3 (1), 93–99.
World Bank. (2000). Turkey Biodiversity and Natural Resource Management Project. Project Document: World Bank Publication.
Yılmaz, A. (2000, November). Akdeniz kıyılarında karasal kaynaklı kirlenmenin boyutu ve canlılara etkisi (The degree and the effect of pollution at the Mediterranean coastlines). Paper presented at 4th Çevre Şurası, Çevre Bakanlığı, İzmir.
Yörek, N., Aydın, H., Ugulu, I., & Doğan, Y. (2008). An investigation on students’ perceptions of biodiversity. Natura Montenerina, Podgonica, 7 (3), 175–184.
Zimmerman, C., & Cuddington, K. (2007). Ambiguous, circular and polysemous: students’ definitions of the “balance of nature” metaphor. Public Understanding of Science, 16 , 393–406.
Zoller, U. (1990). Students’ misunderstandings and alternative conceptions in college freshman chemistry (General and Organic). Journal of Research in Science Teaching, 27 (10), 1053–1065.
Download references
Author information
Authors and affiliations.
Science Education Department, Abant Izzet Baysal University School of Education, Bolu, Turkey
Ahmet Kilinc
Gazi University, Ankara, Turkey
Namik Kemal Yeşiltaş
Ahi Evran University, Kirşehir, Turkey
Tezcan Kartal & Ümit Demiral
Aksaray University, Aksaray, Turkey
Baris Eroğlu
You can also search for this author in PubMed Google Scholar
Corresponding author
Correspondence to Ahmet Kilinc .
Rights and permissions
Reprints and permissions
About this article
Kilinc, A., Yeşiltaş, N.K., Kartal, T. et al. School Students’ Conceptions about Biodiversity Loss: Definitions, Reasons, Results and Solutions. Res Sci Educ 43 , 2277–2307 (2013). https://doi.org/10.1007/s11165-013-9355-0
Download citation
Published : 25 April 2013
Issue Date : December 2013
DOI : https://doi.org/10.1007/s11165-013-9355-0
Share this article
Anyone you share the following link with will be able to read this content:
Sorry, a shareable link is not currently available for this article.
Provided by the Springer Nature SharedIt content-sharing initiative
- Biodiversity
- Biodiversity loss
- Conceptions
- School students
- Find a journal
- Publish with us
- Track your research
Academia.edu no longer supports Internet Explorer.
To browse Academia.edu and the wider internet faster and more securely, please take a few seconds to upgrade your browser .
Enter the email address you signed up with and we'll email you a reset link.
- We're Hiring!
- Help Center
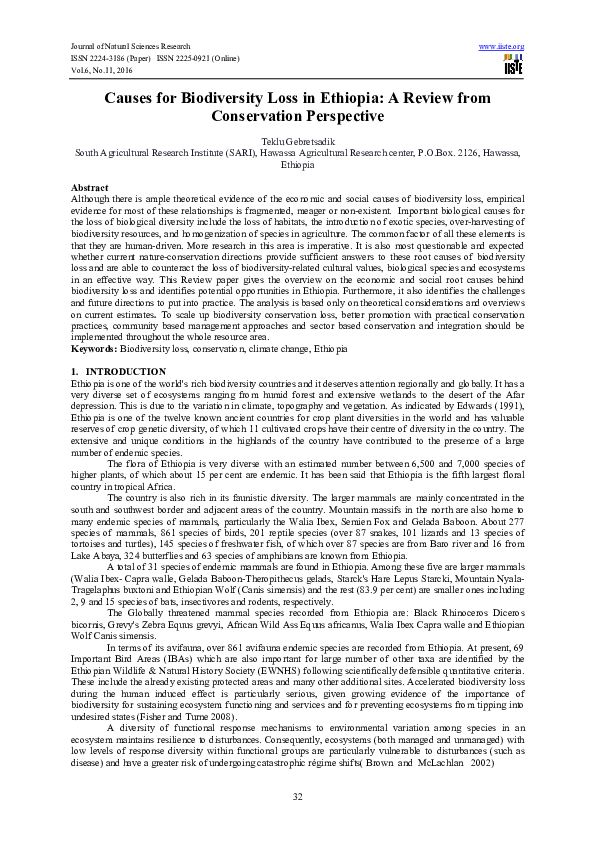

Causes for Biodiversity Loss in Ethiopia: A Review from Conservation Perspective

2016, Journal of Natural Sciences Research
Although there is ample theoretical evidence of the economic and social causes of biodiversity loss, empirical evidence for most of these relationships is fragmented, meager or non-existent. Important biological causes for the loss of biological diversity include the loss of habitats, the introduction of exotic species, over-harvesting of biodiversity resources, and homogenization of species in agriculture. The common factor of all these elements is that they are human-driven. More research in this area is imperative. It is also most questionable and expected whether current nature-conservation directions provide sufficient answers to these root causes of biodiversity loss and are able to counteract the loss of biodiversity-related cultural values, biological species and ecosystems in an effective way. This Review paper gives the overview on the economic and social root causes behind biodiversity loss and identifies potential opportunities in Ethiopia. Furthermore, it also identifi...
Related Papers
Biodiversity Journal
Abdulbasit Hussein
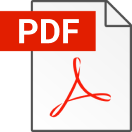
International Journal of Biodiversity and Conservation
Amare gibru mekonnen
Aisa Manlosa
Ensuring food security and halting biodiversity decline are two of the most urgent, interconnected challenges facing humanity in the 21st century. Drawing on both the natural and social sciences, we implemented an interdisciplinary research agenda to address these challenges.<br> This book summarises the findings of five years of research in southwestern Ethiopia. It covers diverse topics, from biodiversity conservation to ecosystem services, livelihoods, social equity, population growth and governance. It concludes with an outlook of what the future may hold for southwestern Ethiopia. Each chapter in the book is based on peer-reviewed empirical science, begins with a summary of key messages, and concludes with a series of recommendations for policy and practice. The book seeks to inspire and inform policymakers, scientists and other stakeholders in Ethiopia and elsewhere, aiming to provide scientifically grounded, tangible insights that can help to harmonise biodiversity cons...
Mellese Damtie (PhD)
This thesis is conducted on the premise that the existing legal, policy and governance frameworks are insufficient to protect biodiversity from the alarming loss it is facing now. It argues that these frameworks are crafted to conform to the dominant paradigm of anthropocentrism; a paradigm which believes that humans are the pinnacle of creation and everything on Earth, including the Earth itself destined to satisfy only the interests of humanity without having their own purpose. By showing how anthropocentric worldview conceived, developed and spread, and how this worldview managed to influence societal collective consciousness to govern the relationship established between humans and the nonhuman nature, the thesis argues that loss of biodiversity not a problem in itself. Rather it is a symptom of the underlying problem rooted in human thinking, guided by anthropocentric worldview. Anthropocentrism has become a powerful paradigm that succeeded in permeating into dominant religions...
Professor Hassen Mamo
Biodiversity and the risk of Infectious Diseases: A review Hassen Mamo (PhD) Department of Microbial, Cellular and Molecular Biology; College of Natural Sciences, Addis Ababa University, PO Box 1176, Addis Ababa, Ethiopia. E-mail: [email protected] Possible correlation between biodiversity and transmission of infectious diseases was proposed over a century ago. Recently, there has been renewed interest in the potential impacts of diversity and disease risk. The effects of diversity on disease transmission have been described for a number of diseases and the underlying specific or general mechanisms have been put forward. It is suggested that increased species diversity could reduce disease risk by regulating the abundance of an important host species thereby pointing to utilitarian functions of biodiversity in the fields of human, animal and plant diseases. Empirical, observational and modeling data are being cited to demonstrate the link of pathogen transmission, including emerging ones, to biodiversity. The author briefly reviewed the literature in the area and touches upon some of the voices of critics. Keywords: biodiversity, infectious diseases, vector-borne
sintayehu eshetu
Mass flowering and death of bamboo: a potential threat to
Desalegn Wana
Landscape Ecology
Frédéric Baudron
International Journal of Natural Resource Ecology and Management
Shemsu Ahmed
Εφημεδία ΜΑΚΕΔΟΝΙΑ
Lina (Triantafyllia) Papadopoulou / Λίνα Παπαδοπούλου
Με το νομοσχέδιο για τα ομόφυλα ζευγάρια αίρεται η δυσμενής διάκριση βάσει φύλου στον γάμο και καταρχήν αναγνωρίζεται η ομογονεϊκότητα, και για αυτό το λόγο κινείται προς τη θετική κατεύθυνση, επισημαίνει στη «ΜτΚ» η καθηγήτρια συνταγματικού δικαίου στο ΑΠΘ Λίνα Παπαδοπούλου.
RELATED PAPERS
Felipe Ribeiro
Biochemistry
Oleg Antzutkin
European Journal of Molecular & Clinical Medicine
NOOR BINTI ROSMAN
如何购买sfu毕业证 西蒙菲莎大学毕业证研究生文凭证书托业成绩单原版一模一样
Current Opinion in Organ Transplantation
Matthias Biebl
Mediterranean Historical Review
Sharon Gerstel
International Review of Administrative Sciences
Journal of Theoretical Biology
Noreen Akhtar
Rocco Liberti
Revista GEARTE
Gustavo Torrezan
Miklós Takács
Ralph HEYNDELS
Gary Harper
Revista Pueblos y fronteras digital
Julieta Martinez Cuero
Desenvolvimento em Debate
Argemiro Brum
Margarita Išoraitė
Marion Brun
Advances in Materials Science and Engineering
Winya Dungkaew
Tamaro Green
Karina Junqueira
RELATED TOPICS
- We're Hiring!
- Help Center
- Find new research papers in:
- Health Sciences
- Earth Sciences
- Cognitive Science
- Mathematics
- Computer Science
- Academia ©2024
Thank you for visiting nature.com. You are using a browser version with limited support for CSS. To obtain the best experience, we recommend you use a more up to date browser (or turn off compatibility mode in Internet Explorer). In the meantime, to ensure continued support, we are displaying the site without styles and JavaScript.
- View all journals
- My Account Login
- Explore content
- About the journal
- Publish with us
- Sign up for alerts
- Open access
- Published: 22 May 2024
Biodiversity loss reduces global terrestrial carbon storage
- Sarah R. Weiskopf ORCID: orcid.org/0000-0002-5933-8191 1 , 2 ,
- Forest Isbell ORCID: orcid.org/0000-0001-9689-769X 3 ,
- Maria Isabel Arce-Plata 4 ,
- Moreno Di Marco ORCID: orcid.org/0000-0002-8902-4193 5 ,
- Mike Harfoot 6 ,
- Justin Johnson ORCID: orcid.org/0000-0001-9903-1787 7 ,
- Susannah B. Lerman 8 ,
- Brian W. Miller ORCID: orcid.org/0000-0003-1716-1161 9 ,
- Toni Lyn Morelli ORCID: orcid.org/0000-0001-5865-5294 2 , 10 ,
- Akira S. Mori ORCID: orcid.org/0000-0002-8422-1198 11 ,
- Ensheng Weng 12 &
- Simon Ferrier 13
Nature Communications volume 15 , Article number: 4354 ( 2024 ) Cite this article
5382 Accesses
39 Altmetric
Metrics details
- Biodiversity
- Climate-change ecology
- Climate-change impacts
- Climate-change mitigation
- Ecosystem services
Natural ecosystems store large amounts of carbon globally, as organisms absorb carbon from the atmosphere to build large, long-lasting, or slow-decaying structures such as tree bark or root systems. An ecosystem’s carbon sequestration potential is tightly linked to its biological diversity. Yet when considering future projections, many carbon sequestration models fail to account for the role biodiversity plays in carbon storage. Here, we assess the consequences of plant biodiversity loss for carbon storage under multiple climate and land-use change scenarios. We link a macroecological model projecting changes in vascular plant richness under different scenarios with empirical data on relationships between biodiversity and biomass. We find that biodiversity declines from climate and land use change could lead to a global loss of between 7.44-103.14 PgC (global sustainability scenario) and 10.87-145.95 PgC (fossil-fueled development scenario). This indicates a self-reinforcing feedback loop, where higher levels of climate change lead to greater biodiversity loss, which in turn leads to greater carbon emissions and ultimately more climate change. Conversely, biodiversity conservation and restoration can help achieve climate change mitigation goals.
Similar content being viewed by others
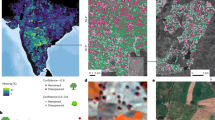
Severe decline in large farmland trees in India over the past decade
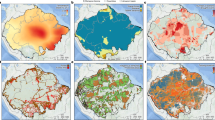
Critical transitions in the Amazon forest system

Plant height as an indicator for alpine carbon sequestration and ecosystem response to warming
Introduction.
Climate change and biodiversity loss have been increasingly recognized as related crises that are most effectively addressed together 1 , 2 , 3 , 4 , 5 . Hundreds of experimental studies have consistently found that within a place, more diverse assemblages, and in particular more diverse plant assemblages, have higher standing biomass production and carbon sequestration 6 , 7 , 8 , 9 . There are several possible mechanisms for this phenomenon. Species with different traits and resource requirements may be able to utilize more resources in an ecosystem through reduced competition, increased facilitation, or both, which leads to overall more efficient resource use 10 , 11 , 12 . At the same time, more diverse assemblages are more likely to contain the most productive species, which can increase overall functioning 13 , 14 . Indeed, biodiversity loss can be one of the major drivers of productivity loss within ecosystems, on par with elevated carbon dioxide or effects of drought 15 . Thus, while climate change can affect biodiversity, biodiversity loss can also affect climate change by altering carbon sequestration and storage 4 , 16 . Despite the contribution that biodiversity itself makes to carbon sequestration, high-level nature-based solution initiatives often focus on increasing the spatial extent of natural ecosystems, particularly forests, and not on their diversity or composition 17 .
Similarly, ecosystem service models do not always account for the effects of biodiversity. Models projecting changes in biodiversity, ecosystem functioning, and ecosystem services typically operate independently and do not account for interactions or feedbacks 18 , 19 . For example, Earth System Models (ESMs) typically model terrestrial ecosystems using a small number of plant functional types and do not include biodiversity-productivity mechanisms 20 , 21 . Not accounting for biodiversity may lead to inaccurate projections of ecosystem function and ecosystem services. For instance, these estimates assume that remnant habitat patches will provide the same level of function even in the face of significant losses of species diversity 22 . Incorporating biodiversity-ecosystem function relationships could improve model accuracy, especially over long timescales as biodiversity effects become stronger over time 23 . For example, an Australian ecosystem modeling exercise found that including species turnover in marine ecosystem models led to very different outcomes for marine fisheries under different climate change scenarios 24 .
Multiple pathways to integrate biodiversity and ecosystem function models have been proposed 25 . One approach that can be applied at the global scale is to connect biodiversity to ecosystem function and ecosystem service models using empirical, observational, or experimental biodiversity-ecosystem function data. Because of the extensive experimental data on the increase in biomass associated with increasing plant species richness 9 , 26 , assessing how loss of plant diversity will affect carbon storage offers a feasible and useful case study to demonstrate the utility of this modeling approach. Moreover, assessments of plant species diversity and carbon storage are relevant for monitoring biodiversity and climate change mitigation goals. Early analyses have been conducted that illustrate this method 4 , 22 . For example, Isbell et al. 22 linked regional estimates of species loss (using species-area relationships) with biodiversity-ecosystem function relationships derived from local-scale experiments. Yet, that work did not consider how climate change or land-use change might affect spatial patterns of species compositional turnover. Species turnover and regional species richness likely have important effects on functioning and stability 27 , 28 , 29 . We build on this previous analysis by accounting for compositional turnover while estimating regional diversity loss.
We use the Biogeographic Infrastructure for Large‐scaled Biodiversity Indicators (BILBI) model to project changes in plant species richness 30 , going beyond the species-area 22 or endemics-area 4 relationships considered in previous studies by also accounting for variation in the species composition of communities (beta diversity) at fine spatial scales. This allows BILBI to be used to assess species persistence/loss over the long term under different scenarios of land-use and climate change 30 , 31 . We link our species-loss estimates with empirical biodiversity-biomass stock relationships 9 to assess the biomass loss, and ultimately carbon storage loss, associated with loss of vascular plant diversity. Although carbon stocks are affected by many global change drivers (e.g., climate, land use), this approach allows us to estimate carbon loss driven specifically by biodiversity loss. Like previous analyses 22 , we use data from local-scale biodiversity experiments to estimate biodiversity-biomass relationships at regional scales. The advantage of using the local experimental data is that by strictly controlling for species richness, composition, and other confounding factors, local experiments can disentangle the causal effects of species richness on biomass production. This assumes that (1) local loss of species diversity is similar to regional scale biodiversity loss, and (2) species loss occurring at the regional scale has consequences for ecosystem functioning. Although the first assumption may not always be met, there is considerable evidence for the second assumption 28 . BILBI produces estimates of plant species loss, based on the local-scale effect of land-use change on species persistence and the regional-scale effect of climate change on species composition. Therefore, we followed previous analyses 22 and used estimates of plant species loss at the ecoregion scale. Our study explores how projected future climate and land-use change scenarios will affect biodiversity loss. We estimate the additional long-term loss of carbon storage resulting indirectly from biodiversity loss that is expected in addition to the direct emissions from land-use change or other climate change impacts on carbon stocks (Fig. 1 ).
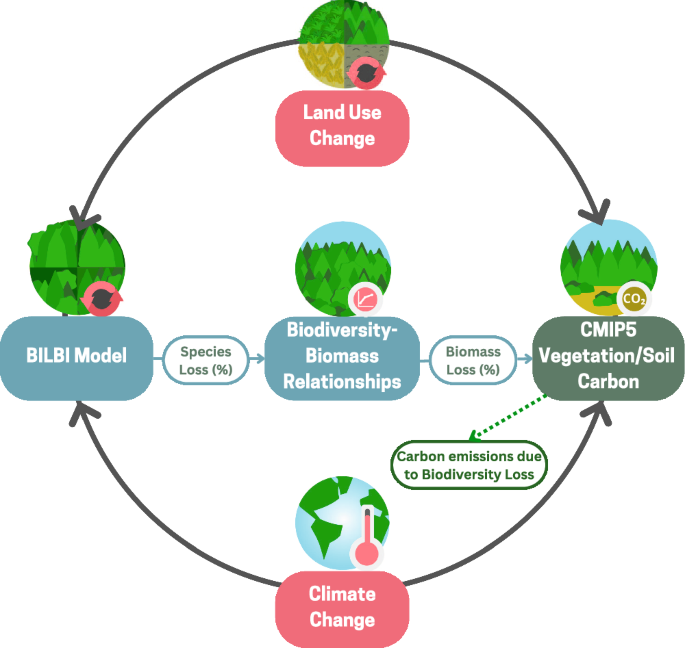
Coupled Model Intercomparison Project (CMIP) and Biogeographic Infrastructure for Large‐scaled Biodiversity Indicators (BILBI) models were used to estimate the effects of land-use and climate change (red boxes) on vegetation/soil carbon and biodiversity, respectively. We used BILBI model output of proportional species loss and empirical biodiversity-biomass relationships to estimate the proportional biomass loss from biodiversity loss (blue boxes). We then applied this proportional biomass loss to soil and vegetation carbon estimates from CMIP5 (green box) using similar climate change and land-use change scenarios as the BILBI model to estimate carbon emissions due to biodiversity loss (green-dashed line).
We used BILBI model projections of the proportion of vascular-plant species expected to persist under “global sustainability” and “fossil-fueled development” scenarios that were produced for a recent model intercomparison project 32 . The BILBI model uses generalized dissimilarity models fitted with more than 52 million records from over 254,000 plant species to map beta diversity at ~1 km 2 scale globally (see refs. 30 , 31 for complete model fitting information). Following Weiskopf et al. 25 (pathway A), we combined beta-diversity estimates with species-area relationships to assess plant species losses in each ecoregion globally, and then used empirical estimates of biodiversity-biomass stock relationships from O’Connor et al. 9 to assess proportional changes in plant biomass. Finally, we used projected terrestrial carbon stock maps (which do not include biodiversity losses) from the Coupled Model Intercomparison Project Phase 5 (CMIP5) IPSL-CM5A-MR model to estimate aboveground plant and soil carbon storage losses associated with projected biodiversity loss in each ecoregion 33 .
Under the global sustainability scenario, the 818 ecoregions lost an average of 16.0% of plant species using a z -value (species-area relationship) of 0.25, ranging from −14.6% to 45.9% for individual ecoregions (Fig. 2 ; see Supplementary Fig. 2 for full range of z -values). This led to an average biomass loss of 4.4% using a b -value (the power relationship between a change in species richness and biomass) of 0.26, ranging from −3.6% to 14.8% (Supplementary Fig. 1 , see Supplementary Fig. 4 for full range of z and b values). This biomass loss was from within remaining vegetation as a result of biodiversity loss, over and above any biomass loss resulting from the direct impact of land-use change under a given scenario. Biodiversity and biomass losses were higher under the fossil-fueled development scenario, with ecoregions losing an average of 20.8% of plant species (ranging from −36.9% to 46.2% across individual ecoregions; Fig. 2 , see Supplementary Fig. 3 for full range of z -values), leading to an average biomass loss of 5.9% (ranging from −8.5% to 14.9%; Supplementary Fig. 1 , see Supplementary Fig. 4 full range of z and b values). In both scenarios, plant species loss, and therefore proportional biomass loss driven by plant species loss were especially high in the tropics. Southern Australia, eastern Europe, and some regions of South America also had high losses.

Plant species loss by ecoregion projected by the Biogeographic Infrastructure for Large‐scaled Biodiversity Indicators (BILBI) model under a global sustainability (SSP1/RCP2.6, A ) and fossil-fueled development (SSP5/RCP8.5, B ) scenario using a species-area relationship of z = 0.25. Darker areas indicate greater plant species loss. Species-loss estimates are what is expected over the long term, when ecosystems approach their new equilibrium states, based on climate and land-use changes projected for 2050.
When we combined the biomass loss values with projected carbon storage maps, we found that overall vegetation carbon loss was greatest in the tropical regions of South America, central Africa, and Southeast Asia, which was driven by which regions store the greatest amount of vegetation carbon and by the level of biodiversity loss (Fig. 3 ). For example, biodiversity loss projections and vegetation carbon were both high in the Amazon, making this a hotspot of biodiversity-driven carbon loss. In contrast, biodiversity loss projections were also high in southern Australia, but because this region has lower vegetation carbon, it was not an area with high biodiversity-driven carbon loss.
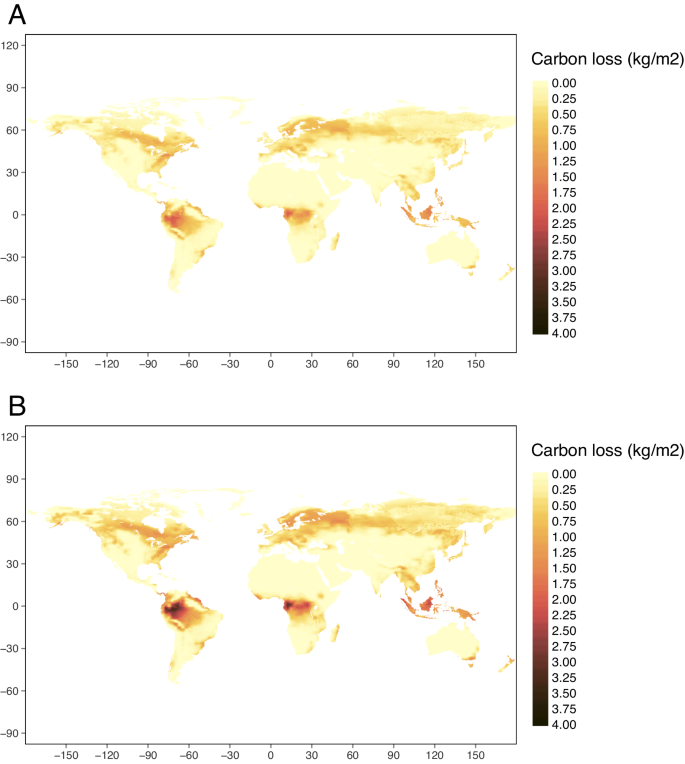
Carbon loss (kg/m 2 ) driven by long-term loss of plant biodiversity by ecoregion under a global sustainability (SSP1/RCP2.6, A ) and fossil-fueled development (SSP5/RCP8.5, B ) scenario using the mean biodiversity-ecosystem functioning slope b = 0.26 and a species-area relationship z = 0.25. Darker areas indicate greater carbon loss. This carbon loss is from within remaining vegetation as a result of biodiversity loss, over and above any carbon loss resulting from the direct impact of land-use change (e.g., deforestation) under a given scenario. Carbon-loss estimates are what is expected over the long term, when ecosystems approach their new equilibrium states, based on climate and land-use changes projected for 2050.
When summed across all terrestrial ecoregions, biodiversity declines led to loss of 7.40–102.68 (29.55 using b = 0.26 and z = 0.25) PgC of vegetation carbon in the long term under global sustainability and 10.83–145.32 (42.89 using b = 0.26 and z = 0.25) PgC under fossil-fueled development. Again, this refers to losses within remaining habitat, above those resulting from direct impacts of land-use change on vegetation extent. The range of carbon-loss values was estimated from the full range of species-area relationships and biodiversity-biomass stock estimates, thus capturing a large range of uncertainty in the strengths of these relationships within and among sites (Supplementary Figs. 5 and 6 ). These carbon losses per ecoregion depended not only on how much plant diversity was lost from the ecoregion, but also the remaining area of the ecoregion, given that they are summed across all remaining habitat (Fig. 4 ). For example, under the global sustainability scenario, the overall loss of carbon was higher from the ecoregions that have lost 10–20% of plant species diversity compared to ecoregions that lost >20% of their diversity because the former cover larger areas (Fig. 4 ).
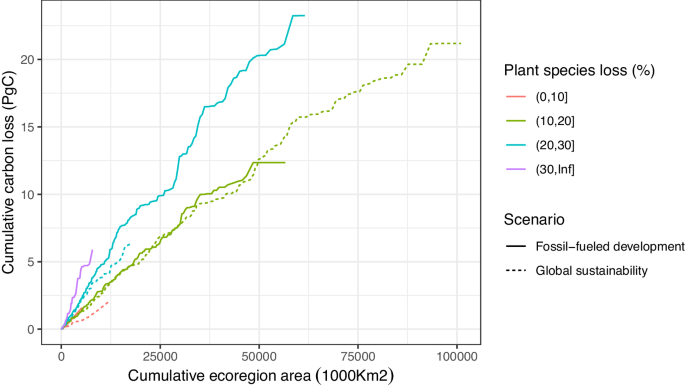
Cumulative carbon loss by cumulative ecoregion area (added from smallest to largest ecoregion size) grouped by proportion of plant species diversity lost in the ecoregion (depicted as different colors) for a global sustainability scenario (SSP1/RCP2.6, dashed line) and fossil-fueled development scenario (SSP5/RCP8.5, solid line). Because carbon losses are summed across all remaining habitat, places with moderate biodiversity loss, collectively, can contribute more to overall carbon loss than areas of high biodiversity loss. For the global sustainability scenario, carbon loss from ecoregions that lost more than 30% of plant species is <0.015 PgC and thus does not show up on the graph. Carbon loss estimates are what is expected over the long term, when ecosystems approach their new equilibrium states, based on climate and land-use changes projected for 2050. Source data are provided as a Source Data file.
Although our uncertainty range was high, projected carbon emissions from biodiversity loss have the potential to rival emissions expected from other sources such as land-use change or melting permafrost (Supplementary Table 1 ). Our models predicted long-term vegetation carbon emissions from long-term biodiversity loss (i.e., over the coming decades as the system moves toward a new equilibrium state) driven by climate and land-use change projections for 2050. These long-term biodiversity-driven carbon emissions were equivalent to about 12–169% of the total emissions expected from land-use change by 2100 for the global sustainability scenario, and about 20–271% for the fossil-fueled development scenario (Supplementary Table 1 ) using the full range of uncertainty from our analysis.
We used a macroecological model to predict changes in ecoregion-level plant species richness and linked it with empirical estimates of the plant biodiversity-biomass stock relationship based on experimental data. We found that biodiversity loss can reduce global carbon storage potential, and although our uncertainty range was large, it could lead to high loss of vegetation carbon. Substantially greater loss is projected under the more intense climate change and land-use change scenario, but even a sustainability scenario (compliant with the Paris target of 2 °C) carries high risks, similar to findings for mammals and wilderness areas 34 , 35 . This engenders a positive feedback wherein higher levels of climate change leads to greater biodiversity loss, which in turn leads to even greater carbon emissions.
Our study builds on a previous analysis that found that land-use change to date could result in the gradual loss of 2–21 PgC as plant species are lost from remaining habitats 22 . We found that projected emissions under future climate and land-use change have the potential to be much higher. In our analysis, we modeled aboveground carbon loss resulting from plant biodiversity loss. Soil carbon may also strongly depend on plant diversity 36 , 37 , 38 , 39 . Carbon loss could increase dramatically if the relationship is on a similar scale to aboveground biomass (18.71–259.72 PgC under the global sustainability scenario and 26.25–353.47 PgC under the fossil-fueled development scenario, Supplementary Figs. 7 and 8 ). A recent analysis using a different approach—linking species distribution models and other ecological modeling approaches with biodiversity-productivity relationships—found that mitigation activities that maintain tree diversity could avoid a 9–39% loss of productivity across terrestrial biomes 4 . Similarly, our estimated carbon loss from biodiversity loss was about 30% lower in the global sustainability scenario compared to the fossil-fueled development scenario. Our analyses used different biodiversity models and climate models, and Mori et al. 4 used estimates of local species loss rather than ecoregion losses as we did here. Together, these findings indicate that biodiversity loss can be a strong driver of carbon emissions.
Priority areas for biodiversity conservation and climate change mitigation could change by accounting for the role of biodiversity in promoting carbon storage. For example, Soto-Navarro et al. 2 identified few areas in central Africa that overlapped as being in the top 20% for both biodiversity and carbon importance. However, we found that carbon losses due to biodiversity loss were high in this area (Fig. 3 ), and therefore that biodiversity protection and restoration here could be highly valuable for climate change mitigation 40 . Several other factors that could contribute to this difference are that we used projected changes in biodiversity and carbon compared to current maps used by Soto-Navarro et al. 2 , and also that we considered only plant diversity while Soto-Navarro et al. 2 looked at mammals, birds, and amphibians. Projected biodiversity loss and associated proportional biomass loss were higher in the Amazon and central Africa under the fossil-fueled development scenario compared to the global sustainability scenario. Interactions between biodiversity loss and ecosystem-level carbon storage led to consistently high losses of carbon in the tropics under both scenarios, specifically in the Amazon, central Africa, and Southeast Asia, and moderately high losses in boreal forests. In other words, because these places store large amounts of carbon, even smaller biodiversity losses under the global sustainability scenario can lead to significant overall loss of carbon. These places thus represent potential hotspots in terms of biodiversity and carbon storage loss.
Earth system models generally project increasing terrestrial carbon accumulation in high latitudes and decreasing accumulation in the tropics 41 . In our analysis, we found that northern latitudes may also experience carbon losses due to biodiversity loss. This could happen in part because our biodiversity model provides a more conservative estimate of potential species gains in these areas (discussed more below). Similar to Mori et al. 4 , we found that total carbon loss from biodiversity loss was also greatest in the tropics (driven by the interaction between biodiversity loss and the location of high carbon stores), which may represent additional losses not captured in current models. Moreover, when proportional loss is considered, other areas such as southern Australia and the European Alps become hotspots of biodiversity and carbon loss (Supplementary Fig. 1 ). A recent intercomparison of ecosystem function models, including dynamic global vegetation models, found similar patterns of carbon loss across South America and central Africa 42 . These models also found high losses in northern Africa, but northern Africa did not come out from our models as a hotspot of biodiversity-driven carbon loss. Interestingly, the model intercomparison found little difference in total ecosystem carbon between global sustainability and fossil-fueled development scenarios, likely due to CO 2 fertilization with higher levels of climate change 42 . Dynamic global vegetation models represent global plant diversity as a small set of plant functional types and simulate their distribution and biogeochemical cycles across the world under different climate and land-use change scenarios. Thus, these models are not accounting for how changes in species diversity within an area will affect biomass. Incorporating biodiversity-biomass relationships could be a useful way to improve such models in the future.
The IPCC estimates that the remaining carbon budgets—the amount of carbon that can be emitted by human activities while still limiting global warming to specified levels—is 140 PgC for limiting warming to 1.5 °C, and 310 PgC for limiting warming to 2 °C, although there is substantial uncertainty around these estimates 41 . While the uncertainty range for biodiversity-driven carbon loss is large, our high-end estimates for carbon loss from biodiversity loss constitute a large proportion of these limits (102.68 PgC under the global sustainability scenario and 145.32 PgC under the fossil-fueled development scenario). Not considering biodiversity loss in emissions scenarios could lead to severe overestimates of terrestrial carbon stocks and remaining carbon budgets.
Overall, our analysis points to the important role that maintaining and/or enhancing the diversity of plant species within areas of natural vegetation, in addition to increasing the extent of these areas, can play in addressing climate change. Alongside increasing the global extent of conservation areas (to prevent rapid carbon loss from ecosystem degradation), increasing plant species diversity in degraded ecosystems can increase carbon storage potential 3 . However, existing international initiatives like the Bonn Challenge and the Paris Agreement focus on forest extent rather than forest quality and composition for protection, afforestation, and reforestation 17 , 43 . Further, initiatives that include biodiversity goals do not always provide clear definitions of what constitutes a biodiverse restoration 44 . This can lead to planting monocultures with non-native species, which could be detrimental to biodiversity and carbon storage over the long-term 17 . Higher biodiversity, with the right species in the right places 45 , could even help reduce the impacts of climate change on biodiversity, and therefore indirectly help maintain carbon storage potential of ecosystems 46 .
Although informative, there are a number of uncertainties and limitations in our analysis that should be refined in future assessments. First, our empirical relationship between biodiversity and biomass stock comes from a meta-analysis of hundreds of experiments conducted at the local scale 9 . Experiments can disentangle the causal effects of species richness on biomass production. However, experiments generally take place over small spatial and temporal scales and may miss important processes like dispersal, evolution, and natural patterns of species assembly and loss 47 . This makes results more difficult to generalize to natural ecosystems, and additional work is needed to do so. See the Pathway A assumptions and challenges section in 25 for more discussion on this limitation. Moreover, the local scale of experimental data does not directly match the ecoregion scale of the BILBI model analysis. As discussed above, this assumes that (1) local loss of species diversity is similar to regional scale biodiversity loss, and (2) species loss occurring at the regional scale has consequences for ecosystem functioning of a similar magnitude to those for species loss at a local scale. There are several theoretical reasons why we expect biodiversity-ecosystem functioning relationships observed at a local level to be equally strong, and perhaps even stronger, across larger spatial extents. Larger spatial and temporal extents will encompass a greater range of environmental conditions. This provides greater opportunity for niche partitioning, and thus positive biodiversity-ecosystem functioning relationships 28 , 48 . Additionally, whole landscapes require more species to maintain ecosystem functioning than do individual locations, with more diversity needed at broader spatial and temporal scales 49 . We presented estimated carbon losses over a large range of potential biodiversity-ecosystem functioning relationship values to capture some of the uncertainties introduced by these assumptions.
Second, the BILBI model assumes that if changes result in non-analog climatic conditions, species will not persist (and thus does not allow for adaptation or tolerance of conditions not experienced at present) and it also does not consider the possibility of increasing species richness in some ecoregions if species are able to exploit new habitat conditions as the climate becomes more suitable. Thus, the model presents a somewhat pessimistic estimate of biodiversity loss from climate change, a common issue with many species distribution model approaches 50 , 51 , 52 . However, native species assemblages have greater complementarity than exotic species assemblages due to longer histories of interactions. Thus, increasing species richness by adding species not previously present in the ecosystem may have a relatively small effect on productivity and may even decrease productivity or decrease the effects of biodiversity on productivity 53 , 54 , 55 .
Third, it is important to correctly interpret the findings from our analysis. The BILBI model uses the species-area relationship to assess plant species persistence, meaning that it projects plant species losses expected in the long term due to habitat conditions in a given year (e.g., poor conditions in 2050 might generate losses beyond 2050). Because the BILBI model does not predict exactly how long it will take for species to disappear once environmental conditions have changed, we do not have an exact date for the projected changes in plant persistence. Therefore, our carbon storage loss estimates are also what is expected over the long term, when ecosystems approach their new equilibrium states, based on climate and land-use changes projected for 2050, whereas land-use and permafrost emissions were estimated from climate and land-use changes from present conditions up to 2100. Although long term is not easily defined, the way that species loss scales with area becomes larger over longer time frames 56 . That is, some species will disappear right away when they lose all suitable habitat, whereas others may disappear over time as remaining habitats are not able to sustain viable populations. Similarly, the effects of biodiversity grow stronger (and less saturating) over time 23 . Thus, estimates produced using smaller species-area ( z -values) and biodiversity-biomass production estimates are more likely over shorter timescales, while larger losses become increasingly likely as more time elapses. By using a range of species-area relationship values, we attempted to capture the range of future biodiversity-loss-driven emissions that might be seen over different time scales.
Finally, our estimates of total carbon loss are based on projected carbon maps from a single general circulation model from CMIP5 (IPSL-CM5A-MR). Our goal was to compare scenarios with each other and provide a range of reasonable carbon loss estimates rather than absolute losses. Scenarios (including emissions and land-use) are a major source of uncertainty compared to global climate models when modeling persistence probability, and the selection of biodiversity modeling approach is also a major source of uncertainty 57 , 58 . We used projected carbon maps from the IPSL-CM5A-MR model to be consistent with our biodiversity model input parameters, but terrestrial carbon uptake estimates vary across CMIP5 and CMIP6 models 59 . Among CMIP5 models assessed, IPSL-CM5A-MR correctly reproduced the global land sink in comparison with historical data, but was not the best performing model for the cVeg variable that we used in this analysis 60 . Recent analysis found that IPSL-CM5A-MR produced estimates of near-present plant carbon within the range of observation-based estimates in the non-circumpolar region, but overestimated the circumpolar regions 21 . Thus, our carbon loss estimates from biodiversity loss may also be overestimated in these regions.
Biological carbon sequestration and biodiversity are tightly linked (Fig. 5 ). Biodiversity-mediated carbon loss has the potential to rival emissions from other sources, so achieving Sustainable Development Goal 15 (Life on Land) can contribute to achieving Goal 13 (Climate Action) 61 . While meeting the Paris Agreement would prevent a large amount of carbon loss compared to a fossil-fueled economic development strategy, this scenario is still associated with potentially high carbon loss via biodiversity loss. Therefore, additional mitigation measures may be needed to meet Paris Agreement expectations even if current emission reduction targets are met. Improving our understanding of how biodiversity will adapt to climate change will be key to improving climate impact predictions. Carbon sequestration by naturally functioning ecosystems is an important element to offset the residual emissions that would occur even with maximum effort toward carbon neutrality.
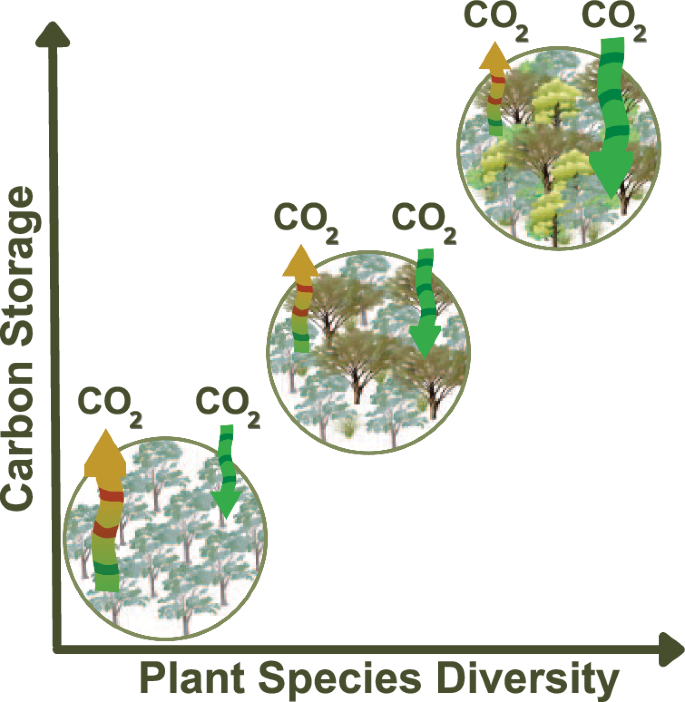
Conceptual graphic representing the role biodiversity plays in biological carbon sequestration. Increasing plant species diversity increases biomass stock. This is depicted as an increasing ratio between carbon sequestration (green arrows) and carbon emissions (yellow arrows). Illustrations from the Integration and Application Network, with no changes made. Images include: “Acer pensylvanicum”, originally published by Joanna Woerner. Integration and Application Network (2010); released under a Creative Commons Attribution-ShareAlike 4.0 International (CC BY-SA 4.0, Acer pensylvanicum (Striped Maple) | Media Library | Integration and Application Network (umces.edu) ). “Eucalyptus spp.”, originally published by Lana Heydon. QLD Department of Environment and Resource Management (2008); released under a Creative Commons Attribution-ShareAlike 4.0 International (CC BY-SA 4.0; Eucalyptus spp. (Eucalypt) 1 | Media Library | Integration and Application Network (umces.edu) ). Acer pensylvanicum (Striped Maple) | Media Library | Integration and Application Network (umces.edu) ). “Acacia spp.”, originally published by Kim Kraeer and Lucy Van Essen-Fishman. Integration and Application Network (2008); released under a Creative Commons Attribution-ShareAlike 4.0 International (CC BY-SA 4.0; Acacia spp. (Acacia) | Media Library | Integration and Application Network (umces.edu) ). “Process; primary production”, originally published by Tracey Saxby. Integration and Application Network (2003); released under a Creative Commons Attribution-ShareAlike 4.0 International (CC BY-SA 4.0; Process; primary production | Media Library | Integration and Application Network (umces.edu) ). “Process; organic carbon release”, originally published by Tracey Saxby. Integration and Application Network (2003); released under a Creative Commons Attribution-ShareAlike 4.0 International (CC BY-SA 4.0; Process; organic carbon release | Media Library | Integration and Application Network (umces.edu) ).
Addressing climate change and biodiversity loss together will more effectively address these crises. Although policymakers are starting to think about climate change mitigation initiatives that have co-benefits for biodiversity, the role of biodiversity itself in promoting carbon storage is often overlooked, with much focus simply on biomass or ecosystem extent. On one hand, this may mean that the scientific community is underestimating future carbon emissions by not accounting for biodiversity-driven carbon losses, thus increasing the urgency for mitigating climate and land-use impacts. On the other hand, this highlights the important role that ecosystem restoration, focusing on the composition of these ecosystems, can play in climate change mitigation. In other words, there is potential to link the restoration target (T2) of the Convention on Biological Diversity (CBD) Kunming-Montreal Global Biodiversity Framework with that for climate-change mitigation (T8) and enhancing nature’s contributions to people (T11), emphasizing a need to reconsider the functional value of biodiversity rather than focusing only on area-based measures for conservation (e.g., so-called 30 by 30; T3) 62 . At a national and local level, this could mean that a focus on maintaining and restoring diverse ecosystems can increase the return-on-investment for carbon storage over the same land area. This may be particularly important for those ecoregions that are projected to have high levels of biodiversity-driven carbon loss.
Our understanding of how biodiversity underpins ecosystem functions and services such as carbon storage has been increasing, but incorporating this knowledge into global projections and conservation policy lags behind 25 , 28 , 63 , 64 , 65 . Our modeling effort provides an important example of how we can effectively link biodiversity, ecosystem functions, and ecosystem services models. As our understanding of biodiversity and ecosystem function relationships improves, our analysis can be updated to reduce the uncertainty in the estimates. Building on and improving the modeling approach used in this study, including by filling the gaps identified in Table 1 , can benefit ESM development and also help identify areas for conservation and restoration and thereby contribute to ongoing processes such as national biodiversity strategy and action plans under the CBD, nationally determined contributions for emissions reduction under the Paris Agreement, and payment for ecosystem services programs.
Step 1—Use BILBI model to estimate proportion of plant species expected to persist in each ecoregion under different climate and land-use scenarios
To assess how land-use change and climate change affect biodiversity, the BILBI model uses land-use data and projections to create a map of habitat condition, which is expressed in units of the proportion of native species expected to remain in each grid-cell, given the land-use type of that cell (Table S1 in ref. 31 ). The model is also able to project climate-driven change in beta-diversity patterns, expressed in terms of the predicted dissimilarity (or conversely similarity) in species composition between any specified pair of grid-cells over both space and time. These projections are coupled with a modified form of species-area analysis to estimate the proportion of species expected to persist (i.e., avoid extinction) under a given scenario of land-use and climate change, within any given region. The use of a species-area relationship through generalized dissimilarity modeling (GDM) approach has been shown to be able to predict trends in biodiversity 31 , 66 , 67 , 68 , 69 , 70 . GDMs perform well as predictors of species composition 68 , 70 . The BILBI model relies on how species richness responds to land-use change locally, and this was tested (fit and validation) as part of the PREDICTS project 66 . Although more work is needed to understand the speed of extinctions following land-use change, species-area-based approaches have been found to be fairly good predictors of the number of threatened or extinct species 67 , 71 . See Supplementary Methods and refs. 30 , 31 for full model and model validation details, but briefly, this is achieved by:
Calculating the total area of similar ecological environments relative to a given cell, by summing the predicted compositional similarity with all other cells under the present climate, and hypothetically assuming the habitat of all cells is in perfect condition.
Calculating the potential area of similar ecological environments under a given future scenario, accounting for both the projected change in climate and the expected condition of habitat under that scenario.
Expressing the effective area of habitat, across similar ecological environments, expected under a given scenario (from step 2 above), as a proportion of the total area of similar environments prior to climate and land-use change (from step 1 above, data available at 72 ), and then using the species-area relationship to translate this proportion into the predicted proportion of species expected to persist over the long term. A species-area exponent of z = 0.25 was used in these calculations, as widely employed in other studies predicting the proportion of species expected to persist in fragmented habitats. However, intact habitats also experience species relaxation (i.e., long-term loss of species as the community approaches equilibrium species richness 73 ), commonly estimated at z = 0.15. To estimate the additional loss of species due to climate and land-use change, we subtracted these two estimates of z to obtain a lower bound of z = 0.1 22 , 74 . We used a range of z values between 0.1 and 0.65, similar to Isbell et al. 22 , to capture some of the uncertainty around the magnitude of species extinction debts.
Our scenario analysis followed the protocols laid out in Kim et al. 32 . We used two scenarios: SSP1/RCP 2.6 (“global sustainability”), a low land-use change and low climate change scenario which is compliant with the Paris target of keeping global warming to below 2 °C by the end of the century compared to pre-industrial times, and SSP5/RCP8.5 (“fossil-fueled development”), a high climate change and intermediate land-use change scenario 75 , 76 . Note that the global sustainability scenario still entails a significant amount of land- use change due to bioenergy production and increased food demand 77 . We chose these scenarios to represent the extreme low- and high-end outcomes to provide a full range of uncertainty estimates. We used land use data from the 0.25° Land Use Harmonization dataset version 2 (LUH2) 78 and climate data from the 1 km WorldClim dataset 79 . For current conditions, we used the LUH2 data for 2015 and the WorldClim data for 1960–1990, and for the future conditions, we used LUH2 data for 2050 and WorldClim data for 2040–2060 31 .
To obtain estimates of the proportion of species expected to persist at the ecoregion level ( \({{{p}}}_{{{{{{\rm{region}}}}}}}\) ), we used a weighted geometric mean of all cells in the ecoregion. The weight applied to each cell is inversely proportional to the total effective area covered by cells with a similar environment to the cell of interest. This means that cells within less extensive environments have a higher weight, since these areas are likely to support more unique species and thus are expected to contribute more to regional species persistence.
Step 2: Use empirical relationships to link changes in species richness to changes in biomass
We use empirical biodiversity-biomass relationships from a recent meta-analysis based on 374 experiments (>500 entries from primary producers, dominated by terrestrial plant studies). They found general support for using a power function to describe how changes in species richness lead to changes in biomass for primary producers as follows 9 :
where a is a constant representing the average biomass of a monoculture for the ecosystem, and b describes the power relationship between a change in richness and biomass. As species richness increases, the biomass of the system will increase compared to the monoculture baseline, but the amount of increase per species decelerates as more species are added. This equation can be converted to proportion of remaining biomass ( \({{{p}}}_{{{{{{\rm{biomass}}}}}}}\) ) based on proportional change in species richness per ecoregion as follows:
We apply this transformation to the BILBI model output to assess the proportion of remaining biomass from the proportion of remaining plant species richness, using the mean b = 0.26, as well as the 95% CI to provide uncertainty estimates around our results. O’Connor et al. 9 found that for primary producers, b = 0.26 (with a 95% CI of 0.16–0.37) was valid for most assemblages and was robust to differences in experimental design and the range of species richness levels considered. While they did not find an effect of study duration on b values, previous studies have found that biodiversity-productivity relationships grow stronger over time 23 . Although b values can vary spatially 4 , 80 , there is still uncertainty in how biodiversity-ecosystem functioning relationships differ across space and in how factors like climate, environmental conditions, and species trait compositions might systematically affect the observed relationship 9 . If the places where habitat destruction is highest are also the places that tend to have the highest or lowest biodiversity-ecosystem functioning relationships, then using a narrower range of spatially explicit values could systematically over or underestimate the carbon storage loss associated with this biodiversity loss. To address this concern, we estimated productivity losses associated with the full confidence interval range from O’Connor et al. 9 . Thus, rather than considering a single slope for the biodiversity-ecosystem functioning relationship, we consider a range of relationships that reflects variation in both composition and site-to-site differences found among previous biodiversity experiments. For example, the range of relationship values is wider than spatially explicit values estimated from in-situ forest re-measurement data globally (range = 0.198–0.299, mean = 0.26) 80 . Therefore, our range of b values provides a conservative range of estimates of productivity loss associated with biodiversity loss. In addition, although estimates come from historical data, we do not currently have estimates of how relationships may change in the future. Experimental evidence suggests, however, that positive biodiversity-productivity relationships are robust to droughts and changes in nutrient availability 81 .
Step 3: Estimate total changes in carbon storage and compare to other global change drivers
The previous step provided spatially explicit estimates of proportional change in biomass associated with loss of biodiversity for each scenario. To convert biomass change to carbon storage change, we multiplied the gridded estimates of proportional change in biomass from the BILBI model by a global map of terrestrial carbon stock from CMIP5. The CMIP model estimates changes in carbon storage from climate change and land use change, but does not account for changes in species richness. By using these model projections as our baseline carbon estimate, we can assess the effects of biodiversity loss on carbon storage that are expected on top of the direct changes from climate change and land use change that have already been incorporated in initial carbon storage projections.
We used terrestrial carbon storage maps that considered only vegetation carbon, as well as maps considering vegetation and soil carbon. We calculated the average cVeg and cSoil value over a 12-month period in 2050 (the end year for the BILBI model output). Model inputs were not exactly the same, making it difficult to be consistent with the climate and land use input data used to estimate biodiversity loss and carbon storage. We downloaded the total carbon in vegetation (cVeg) and total carbon in soil (cSoil) layer from the CMIP5 IPSL-CM5A-MR model 33 . The biodiversity and ecosystem services models using harmonized scenarios (BES-SIM) used climate data from either the lower resolution IPSL-CM5A-LR or 1 km WorldClim data downscaled from the IPSL-CM5A-LR depending on biodiversity model requirements 32 . We chose to use the mid-resolution 1.25° × 2.5° CMIP5 IPSL-CM5A-MR model to obtain higher resolution carbon maps. We obtained cVeg and cSoil for both of our scenarios—global sustainability (SSP1/RCP 2.6) and fossil-fueled development (SSP5/RCP8.5) from the Earth System Grid Federation (ESGF; https://esgf-node.llnl.gov/search/cmip5/ ). The BILBI model used land use data from the Land Use Harmonization dataset version 2 78 , while the CMIP5 IPSL-CM5A-MR model used land use data from the Land Use Harmonization dataset version 1 82 . Version 2 provides higher resolution data over a longer time frame with more detailed land-use categories 78 . Although the input data are slightly different, we do not believe this invalidates the approach, as we are not comparing carbon storage changes between the models but instead using the CMIP5 IPSL-CM5A-MR model to estimate potential carbon storage under the global sustainability and fossil-fueled development scenarios, which we then use to estimate the possible magnitude of carbon storage loss driven by biodiversity loss under the same scenarios.
Soil type, climate, and land use are important drivers of soil carbon. Plant diversity can also increase soil carbon, and these relationships grow stronger over time 37 , 38 , 39 . If soil carbon depends strongly on plant diversity, then it is important to consider the possible magnitude of plant loss on soil carbon, even if the strength of these relationships is not fully established. We assessed how large soil carbon losses could be if plant biodiversity-soil carbon relationships are on a similar magnitude to aboveground biomass. We thus consider the additional impact that plant loss may have on soil carbon on top of the local environmental conditions considered in the CMIP models. We excluded soil types that are more likely to be impacted by drying and warming than by changes in plant diversity, including wetland (Gleysols), peatland (Histosols), and permafrost (Cryosols) soils 22 . We note that these soil types represent major global carbon stores. If biodiversity does in fact drive carbon loss in these ecosystems, we could be missing additional losses. Specifically, we resampled the 250 m predicted World Reference Base 2006 subgroup soil classification (ISRIC, https://data.isric.org/geonetwork/srv/eng/catalog.search#/metadata/5c301e97-9662-4f77-aa2d-48facd3c9e14 83 ); to the same resolution as the cSoil raster layer using the nearest neighbor method in the R software program terra package 84 , and then masked out these soil types from the cSoil raster.
We did not account for potential changes in litter carbon. Increasing biodiversity increases the rate of litter decomposition (i.e., less litter mass storage), which could add to increasing decomposition from warming, and thus we would expect biodiversity loss to increase litter carbon storage. While the strength of the biodiversity-carbon relationships for soil and litter are not fully established, the effects on litter carbon are likely weaker than those on plant biomass or soil carbon 6 , 85 , 86 . For example, decomposition was 34.7% higher in mixed species forests compared to monocultures, while soil carbon storage was 178% higher in mixed grasslands than in monocultures 39 , 86 . Moreover, the estimated effects of diversity on plant biomass and soil carbon were driven by short-term studies, and these relationships grow stronger over time in long-term experiments 23 , 36 , 39 .
To obtain cVeg and cSoil values on the same scale as the biodiversity data, we resampled by ecoregion using bilinear interpolation. Then, we multiplied our raster layers (proportion of remaining plant biomass and 2050 carbon maps), to obtain changes in carbon storage in 2050 in kg/m 2 ( ΔC ), such that:
To convert this to total C storage in PgC ( \({{{C}}}_{{{{{{\rm{total}}}}}}}\) ), we used the cellSize function in the terra package 84 to calculate the total area in m 2 of each ecoregion ( A ). We then multiplied this by the carbon storage layer to obtain total carbon storage lost per ecoregion, which we summed to obtain global C storage loss values:
where k = a given ecoregion and n = total number of ecoregions.
We conducted all analyses in R version 4.1.1 87 , and produced all graphics using either the tmap or ggplot2 packages 88 , 89 (Supplementary Software 1 – 6 ).
Data availability
BILBI model data are available at https://doi.org/10.6084/m9.figshare.25188650 . CMIP data are available from the Earth System Grid Federation (ESGF; https://esgf-node.llnl.gov/search/cmip5/ ). World Reference Base 2006 subgroup soil classification data are available from ISRIC, https://data.isric.org/geonetwork/srv/eng/catalog.search#/metadata/5c301e97-9662-4f77-aa2d-48facd3c9e14 83 . Raster data for the maps generated in this study have been deposited in ScienceBase at https://doi.org/10.5066/P13WUFMU 90 . Source data are provided with this paper.
Code availability
R scripts are included as Supplementary files.
Di Marco, M. et al. Synergies and trade-offs in achieving global biodiversity targets. Conserv. Biol. 30 , 189–195 (2016).
Article PubMed Google Scholar
Soto-Navarro, C. et al. Mapping co-benefits for carbon storage and biodiversity to inform conservation policy and action. Philos. Trans. R. Soc. B Biol. Sci. 375 , 20190128 (2020).
Strassburg, B. B. N. et al. Global priority areas for ecosystem restoration. Nature 586 , 724–729 (2020).
Article ADS CAS PubMed Google Scholar
Mori, A. S. et al. Biodiversity–productivity relationships are key to nature-based climate solutions. Nat. Clim. Change 11 , 1–8 (2021).
Pörtner, H. O. et al. IPBES-IPCC Co-Sponsored Workshop Report on Biodiversity and Climate Change . www.ipbes.net ; https://doi.org/10.5281/zenodo.4782538 (2021).
Cardinale, B. J. et al. The functional role of producer diversity in ecosystems. Am. J. Bot. 98 , 572–592 (2011).
Cardinale, B. J. et al. Biodiversity loss and its impact on humanity. Nature 489 , 326–326 (2012).
Article ADS CAS Google Scholar
Duffy, E. J., Godwin, C. M. & Cardinale, B. J. Biodiversity effects in the wild are common and as strong as key drivers of productivity. Nature 549 , 261–264 (2017).
O’Connor, M. I. et al. A general biodiversity–function relationship is mediated by trophic level. Oikos 126 , 18–31 (2017).
Article ADS Google Scholar
Hooper, D. U. et al. Effects of biodiversity on ecosystem functioning: a consensus of current knowledge. Ecol. Monogr. 75 , 3–35 (2005).
Article Google Scholar
Loreau, M. & Hector, A. Partitioning selection and complementarity in biodiversity experiments. Nature 412 , 72–76 (2001).
Tilman, D., Lehman, C. L. & Thomson, K. T. Plant diversity and ecosystem productivity: theoretical considerations. Proc. Natl Acad. Sci. USA 94 , 1857–1861 (1997).
Article ADS CAS PubMed PubMed Central Google Scholar
Aarssen, L. W. High productivity in grassland ecosystems: effected by species diversity or productive species? Oikos 80 , 183 (1997).
Hooper, D. U. The role of complementarity and competition in ecosystem responses to variation in plant diversity. Ecology 79 , 704–719 (1998).
Hooper, D. U. et al. A global synthesis reveals biodiversity loss as a major driver of ecosystem change. Nature 486 , 105–108 (2012).
Weiskopf, S. R. et al. Climate change effects on biodiversity, ecosystems, ecosystem services, and natural resource management in the United States. Sci. Total Environ. 733 , 137782 (2020).
Seddon, N., Turner, B., Berry, P., Chausson, A. & Girardin, C. A. J. Grounding nature-based climate solutions in sound biodiversity science. Nat. Clim. Change 9 , 84–87 (2019).
Ferrier, S., Ninan, K. N., Leadley, P. & Alkemade, R. The Methodological Assessment Report on Scenarios and Models of Biodiversity and Ecosystem Services 32 (IPBES, 2016).
O’Connor, M. I. et al. Grand challenges in biodiversity–ecosystem functioning research in the era of science–policy platforms require explicit consideration of feedbacks. Proc. R. Soc. B Biol. Sci. 288 , 20210783 (2021).
Zhou, J. et al. A traceability analysis system for model evaluation on land carbon dynamics: design and applications. Ecol. Process. 10 , 12 (2021).
Wei, N. et al. Evolution of uncertainty in terrestrial carbon storage in Earth system models from CMIP5 to CMIP6. J. Clim. 35 , 5483–5499 (2022).
Isbell, F., Tilman, D., Polasky, S. & Loreau, M. The biodiversity-dependent ecosystem service debt. Ecol. Lett. 18 , 119–134 (2015).
Reich, P. B. et al. Impacts of biodiversity loss escalate through time as redundancy fades. Science 336 , 589–592 (2012).
Fulton, E. A. & Gorton, R. Adaptive Futures for SE Australian Fisheries & Aquaculture: Climate Adaptation Simulations (CSIRO, 2014).
Weiskopf, S. R. et al. A conceptual framework to integrate biodiversity, ecosystem function, and ecosystem service models. BioScience. 72 , 1–12 (2022).
O’Connor, M. I., Bernhardt, J. R., Stark, K., Usinowicz, J. & Whalen, M. A. In The Ecological and Societal Consequences of Biodiversity Loss (eds Loreau, M., Hector, A. & Isbell, F.) 97–118 (Wiley, 2022).
Wang, S. & Loreau, M. Biodiversity and ecosystem stability across scales in metacommunities. Ecol. Lett. 19 , 510–518 (2016).
Article PubMed PubMed Central Google Scholar
Isbell, F. et al. Linking the influence and dependence of people on biodiversity across scales. Nature 546 , 65–72 (2017).
Mori, A. S., Isbell, F. & Seidl, R. β-Diversity, community assembly, and ecosystem functioning. Trends Ecol. Evol. 33 , 549–564 (2018).
Hoskins, A. J. et al. BILBI: supporting global biodiversity assessment through high-resolution macroecological modelling. Environ. Model. Softw. 132 , 104806 (2020).
Di Marco, M. et al. Projecting impacts of global climate and land-use scenarios on plant biodiversity using compositional-turnover modelling. Glob. Change Biol. 25 , 2763–2778 (2019).
Kim, H. et al. A protocol for an intercomparison of biodiversity and ecosystem services models using harmonized land-use and climate scenarios. Geosci. Model Dev. 11 , 4537–4562 (2018).
Dufresne, J. L. et al. Climate change projections using the IPSL-CM5 Earth system model: from CMIP3 to CMIP5. Clim. Dyn. 40 , 2123–2165 (2013).
Asamoah, E. F. et al. Land-use and climate risk assessment for Earth’s remaining wilderness. Curr. Biol. 32 , 4890–4899.e4 (2022).
Article CAS PubMed Google Scholar
Mendez Angarita, V. Y., Maiorano, L., Dragonetti, C. & Di Marco, M. Implications of exceeding the Paris Agreement for mammalian biodiversity. Conserv. Sci. Pract. 5 , e12889 (2023).
Yang, Y. et al. Restoring abandoned farmland to mitigate climate change on a full Earth. One Earth 3 , 176–186 (2020).
Chen, X. et al. Effects of plant diversity on soil carbon in diverse ecosystems: a global meta-analysis. Biol. Rev. 95 , 167–183 (2020).
Chen, X. et al. Tree diversity increases decadal forest soil carbon and nitrogen accrual. Nature 618 , 94–101 (2023).
Yang, Y., Tilman, D., Furey, G. & Lehman, C. Soil carbon sequestration accelerated by restoration of grassland biodiversity. Nat. Commun. 10 , 1–7 (2019).
Google Scholar
Cimatti, M., Chaplin-Kramer, R. & Marco, M. D. Regions of High Biodiversity Value Preserve Nature’s Contributions to People under Climate Change . https://www.researchsquare.com/article/rs-2013582/v1 ; https://doi.org/10.21203/rs.3.rs-2013582/v1 (2022).
Canadell, J. G. et al. Global carbon and other biogeochemical cycles and feedbacks. In Climate Change 2021: The Physical Science Basis . Contribution of Working Group I to the Sixth Assessment Report of the Intergovernmental Panel on Climate Change (eds Masson-Delmotte, V. et al.) 673–816 (Cambridge University Press, 2021).
Pereira, H. M. et al. Global trends in biodiversity and ecosystem services from 1900 to 2050. bioRxiv 1 , 1–5 (2020).
Mori, A. S. Advancing nature-based approaches to address the biodiversity and climate emergency. Ecol. Lett. 23 , 1729–1732 (2020).
Andres, S. E. et al. Defining biodiverse reforestation: why it matters for climate change mitigation and biodiversity. Plants People Planet 1–12 https://doi.org/10.1002/ppp3.10329 (2022).
van der Plas, F., Hennecke, J., Chase, J. M., van Ruijven, J. & Barry, K. E. Universal beta-diversity–functioning relationships are neither observed nor expected. Trends Ecol. Evol. S0169534723000125 https://doi.org/10.1016/j.tree.2023.01.008 (2023).
Hisano, M., Searle, E. B. & Chen, H. Y. H. Biodiversity as a solution to mitigate climate change impacts on the functioning of forest ecosystems. Biol. Rev. 93 , 439–456 (2018).
Winfree, R., Fox, J. W., Williams, N. M., Reilly, J. R. & Cariveau, D. P. Abundance of common species, not species richness, drives delivery of a real-world ecosystem service. Ecol. Lett. 18 , 626–635 (2015).
Gonzalez, A. et al. Scaling-up biodiversity-ecosystem functioning research. Ecol. Lett. 23 , 757–776 (2020).
Thompson, P. L. et al. Scaling up biodiversity-ecosystem functioning relationships: the role of environmental heterogeneity in space and time. Proc. R. Soc. B Biol. Sci. 288 , 20202779 (2021).
Benito Garzón, M., Robson, T. M. & Hampe, A. ΔTraitSDMs: species distribution models that account for local adaptation and phenotypic plasticity. N. Phytologist 222 , 1757–1765 (2019).
Sinclair, S. J., White, M. D. & Newell, G. R. How useful are species distribution models for managing biodiversity under future climates? Ecol. Soc. 15 , 8 (2010).
Bush, A. et al. Incorporating evolutionary adaptation in species distribution modelling reduces projected vulnerability to climate change. Ecol. Lett. 19 , 1468–1478 (2016).
Wilsey, B. J., Teaschner, T. B., Daneshgar, P. P., Isbell, F. I. & Polley, H. W. Biodiversity maintenance mechanisms differ between native and novel exotic-dominated communities. Ecol. Lett. 12 , 432–442 (2009).
Dee, L. E. et al. Clarifying the effect of biodiversity on productivity in natural ecosystems with longitudinal data and methods for causal inference. Nat. Commun. 14 , 2607 (2023).
Isbell, F. I. & Wilsey, B. J. Increasing native, but not exotic, biodiversity increases aboveground productivity in ungrazed and intensely grazed grasslands. Oecologia 165 , 771–781 (2011).
Article ADS PubMed Google Scholar
Rosenzweig, M. L. Heeding the warning in biodiversity’s basic law. Science 284 , 276–277 (1999).
Article CAS Google Scholar
Thuiller, W., Guéguen, M., Renaud, J., Karger, D. N. & Zimmermann, N. E. Uncertainty in ensembles of global biodiversity scenarios. Nat. Commun. 10 , 1446 (2019).
Article ADS PubMed PubMed Central Google Scholar
Leclère, D. et al. Bending the curve of terrestrial biodiversity needs an integrated strategy. Nature 585 , 551–556 (2020).
Arora, V. K. et al. Carbon-concentration and carbon-climate feedbacks in CMIP6 models and their comparison to CMIP5 models. Biogeosciences 17 , 4173–4222 (2020).
Anav, A. et al. Evaluating the land and ocean components of the global carbon cycle in the CMIP5 Earth system models. J. Clim. 26 , 6801–6843 (2013).
United Nations. Transforming Our World: The 2030 Agenda for Sustainable Development (2015).
United Nations Convention on Biological Diversity. Kunming-Montreal Global Biodiversity Framework . (2022).
Rosa, I. M. D. et al. Multiscale scenarios for nature futures. Nat. Ecol. Evol. 1 , 1416–1419 (2017).
Rosa, I. M. D. et al. Challenges in producing policy-relevant global scenarios of biodiversity and ecosystem services. Glob. Ecol. Conserv. 22 , e00886 (2020).
van der Plas, F. Biodiversity and ecosystem functioning in naturally assembled communities. Biol. Rev. https://doi.org/10.1111/brv.12499 (2019).
Newbold, T. et al. Global effects of land use on local terrestrial biodiversity. Nature 520 , 45–50 (2015).
Brooks, T. M. et al. Habitat loss and extinction in the hotspots of biodiversity. Conserv. Biol. 16 , 909–923 (2002).
Elith, J. et al. Novel methods improve prediction of species’ distributions from occurrence data. Ecography 29 , 129–151 (2006).
Blois, J. L., Williams, J. W., Fitzpatrick, M. C., Jackson, S. T. & Ferrier, S. Space can substitute for time in predicting climate-change effects on biodiversity. Proc. Natl Acad. Sci. 110 , 9374–9379 (2013).
Ware, C. et al. Improving biodiversity surrogates for conservation assessment: a test of methods and the value of targeted biological surveys. Divers. Distrib. 24 , 1333–1346 (2018).
Pimm, S. L., Jenkins, C. N. & Li, B. V. How to protect half of earth to ensure it protects sufficient biodiversity. Sci. Adv. 4 , 1–9 (2018).
Di Marco, M., Hoskins, A. J., Harwood, T. D., Ware, C. & Ferrier, S. BILBI model data for SSP1/RCP2.6 and SSP5/RCP8.5. figshare https://doi.org/10.6084/m9.figshare.25188650 (2024).
Diamond, J. M. Biogeographic kinetics: estimation of relaxation times for Avifaunas of Southwest Pacific Islands. Proc. Natl Acad. Sci. 69 , 3199–3203 (1972).
Gonzalez, A. In Encyclopedia of Life Sciences https://doi.org/10.1002/9780470015902.a0021230 (Wiley, 2009).
Kriegler, E. et al. Fossil-fueled development (SSP5): an energy and resource intensive scenario for the 21st century. Glob. Environ. Change 42 , 297–315 (2017).
van Vuuren, D. P. et al. Energy, land-use and greenhouse gas emissions trajectories under a green growth paradigm. Glob. Environ. Change 42 , 237–250 (2017).
Ciais, P. et al. Carbon and other biogeochemical cycles. In Climate Change 2013: The Physical Science Basis . Contribution of Working Group I to the Fifth Assessment Report of the iItergovernmental Panel on Climate Change (eds Stocker, T. F. et al.) 465–570 (Cambridge University Press, 2013).
Hurtt, G. C. et al. Harmonization of global land use change and management for the period 850-2100 (LUH2) for CMIP6. Geosci. Model Dev. 13 , 5425–5464 (2020).
Hijmans, R. J., Cameron, S. E., Parra, J. L., Jones, P. G. & Jarvis, A. WorldClim Global Climate Data Version 1 . http://worldclim.org/version1 (2017).
Liang, J. et al. Positive biodiversity-productivity relationship predominant in global forests. Science 354 , aaf8957 (2016).
Craven, D. et al. Plant diversity effects on grassland productivity are robust to both nutrient enrichment and drought. Philos. Trans. R. Soc. B 371 , 20150277 (2016).
Hurtt, G. C. et al. Harmonization of land-use scenarios for the period 1500–2100: 600 years of global gridded annual land-use transitions, wood harvest, and resulting secondary lands. Clim. Change 109 , 117 (2011).
Hengl, T. et al. SoilGrids250m: global gridded soil information based on machine learning. PLoS ONE 12 , e0169748 (2017).
Hijmans, R. J. terra: Spatial Data Analysis. R package version 1.7-39. (2023).
Balvanera, P. et al. Quantifying the evidence for biodiversity effects on ecosystem functioning and services. Ecol. Lett. 9 , 1146–1156 (2006).
Mori, A. S., Cornelissen, J. H. C., Fujii, S., Okada, K. & Isbell, F. A meta-analysis on decomposition quantifies afterlife effects of plant diversity as a global change driver. Nat. Commun. 11 , 1–9 (2020).
R Core Team. R: A Language and Environment for Statistical Computing (R Foundation for Statistical Computing, 2021).
Wickham, H. Ggplot2: Elegant Graphics for Data Analysis (Springer-Verlag, 2016).
Tennekes, M. tmap: Thematic Maps in R. J. Stat. Softw. 84 , 1–39 (2018).
Weiskopf, S. R. et al. Biodiversity Loss Reduces Global Terrestrial Carbon Storage—Data . U.S. Geological Survey data release (2024).
Download references
Acknowledgements
This work was supported by the National Socio-Environmental Synthesis Center under funding received from the National Science Foundation (grant no. DBI-1639145). A portion of this research was supported by the US Geological Survey National and North Central Climate Adaptation Science Centers. MDM received support from the European Union–NextGenerationEU as part of the National Biodiversity Future Center, Italian National Recovery and Resilience Plan (NRRP) Mission 4 Component 2 Investment 1.4 (CUP: B83C22002950007). We thank the Beth Fulton, the Diversity and Eco-Function working group, and the Morelli lab group for their feedback. We thank Alexey Shiklomanov for R coding assistance. Any use of trade, firm, or product names is for descriptive purposes only and does not imply endorsement by the US Government.
Author information
Authors and affiliations.
U.S. Geological Survey National Climate Adaptation Science Center, Reston, VA, USA
Sarah R. Weiskopf
Department of Environmental Conservation, University of Massachusetts, Amherst, MA, USA
Sarah R. Weiskopf & Toni Lyn Morelli
Department of Ecology, Evolution and Behavior, University of Minnesota, Saint Paul, MN, USA
Forest Isbell
Département de Sciences Biologiques, Université de Montréal, Montréal, QC, H3T 1J4, Canada
Maria Isabel Arce-Plata
Department of Biology and Biotechnologies, Sapienza University of Rome, Rome, Italy
Moreno Di Marco
Vizzuality, 123 Calle de Fuencarral, 28010, Madrid, Spain
Mike Harfoot
Department of Applied Economics, University of Minnesota, 1994 Buford Ave, Saint Paul, MN, 55105, USA
Justin Johnson
USDA Forest Service Northern Research Station, Amherst, MA, USA
Susannah B. Lerman
U.S. Geological Survey North Central Climate Adaptation Science Center, Boulder, CO, USA
Brian W. Miller
U.S. Geological Survey Northeast Climate Adaptation Science Center, Amherst, MA, USA
Toni Lyn Morelli
Research Center for Advanced Science and Technology, the University of Tokyo, 4-6-1 Komaba, Meguro, Tokyo, 153-8904, Japan
Akira S. Mori
Columbia University/NASA Goddard Institute for Space Studies, 2880 Broadway, New York, NY, 10025, USA
Ensheng Weng
CSIRO Environment, Canberra, ACT, 2601, Australia
Simon Ferrier
You can also search for this author in PubMed Google Scholar
Contributions
S.R.W., F.I., and S.F. conceived the project. S.R.W., F.I., S.F., M.I.A.P., M.D.M., M.H., J.J., A.S.M., and E.W. contributed to the design of the analysis. S.R.W. led the analyses and wrote the initial paper draft. All authors, including B.W.M., S.B.L., and T.L.M., contributed substantively to revisions.
Corresponding author
Correspondence to Sarah R. Weiskopf .
Ethics declarations
Competing interests.
The authors declare no competing interests.
Peer review
Peer review information.
Nature Communications thanks Rob Alkemade, Eduardo Gomes and Aafke Schipper for their contribution to the peer review of this work. A peer review file is available.
Additional information
Publisher’s note Springer Nature remains neutral with regard to jurisdictional claims in published maps and institutional affiliations.
Supplementary information
Peer review file, description of additional supplementary files, supplementary software 1, supplementary software 2, supplementary software 3, supplementary software 4, supplementary software 5, supplementary software 6, source data, source data, rights and permissions.
Open Access This article is licensed under a Creative Commons Attribution 4.0 International License, which permits use, sharing, adaptation, distribution and reproduction in any medium or format, as long as you give appropriate credit to the original author(s) and the source, provide a link to the Creative Commons licence, and indicate if changes were made. The images or other third party material in this article are included in the article’s Creative Commons licence, unless indicated otherwise in a credit line to the material. If material is not included in the article’s Creative Commons licence and your intended use is not permitted by statutory regulation or exceeds the permitted use, you will need to obtain permission directly from the copyright holder. To view a copy of this licence, visit http://creativecommons.org/licenses/by/4.0/ .
Reprints and permissions
About this article
Cite this article.
Weiskopf, S.R., Isbell, F., Arce-Plata, M.I. et al. Biodiversity loss reduces global terrestrial carbon storage. Nat Commun 15 , 4354 (2024). https://doi.org/10.1038/s41467-024-47872-7
Download citation
Received : 04 August 2023
Accepted : 11 April 2024
Published : 22 May 2024
DOI : https://doi.org/10.1038/s41467-024-47872-7
Share this article
Anyone you share the following link with will be able to read this content:
Sorry, a shareable link is not currently available for this article.
Provided by the Springer Nature SharedIt content-sharing initiative
By submitting a comment you agree to abide by our Terms and Community Guidelines . If you find something abusive or that does not comply with our terms or guidelines please flag it as inappropriate.
Quick links
- Explore articles by subject
- Guide to authors
- Editorial policies
Sign up for the Nature Briefing newsletter — what matters in science, free to your inbox daily.

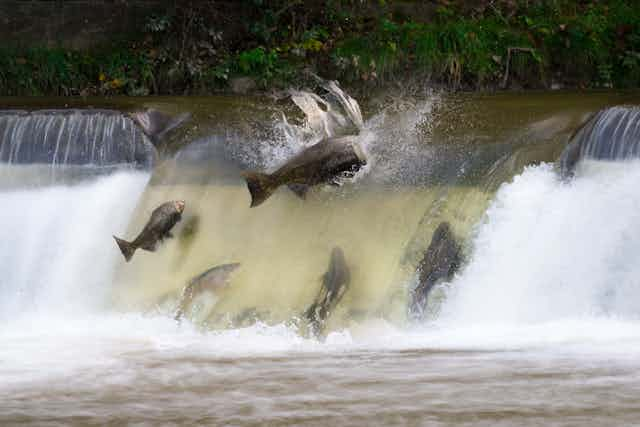
The surge in hydroelectric dams is driving massive biodiversity loss
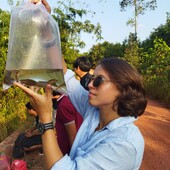
Assistant Prof. Ecology, University of Leeds
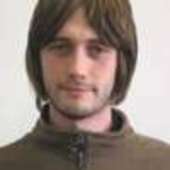
Professor of Aquatic Science, University of Leeds
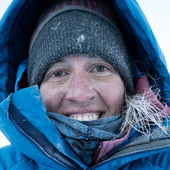
Senior independent research fellow, University of Leeds
Disclosure statement
Lee Brown receives funding from Yorkshire Water for research that aims to improve knowledge of how environmental flows from reservoirs influence downstream river ecosystems
Taylor Maavara receives funding from the Natural Environment Research Council (NERC) in the United Kingdom.
Josie South does not work for, consult, own shares in or receive funding from any company or organisation that would benefit from this article, and has disclosed no relevant affiliations beyond their academic appointment.
University of Leeds provides funding as a founding partner of The Conversation UK.
View all partners
Around the world, free-flowing natural rivers are being fragmented by dams, weirs and other barriers. It’s one of the biggest, yet least acknowledged, causes of biodiversity loss worldwide.
River fragmentation is a key reason migratory fish populations have declined by 81% since 1970, according to a new report by the Living Planet Index – a rate of wildlife loss six times that of animals that live on land or in the sea.
These migratory fish need to move between different parts of rivers, estuaries and oceans at different stages in their lives. For instance salmon go from the sea to rivers or lakes to reproduce, while eels do the opposite.
Some species migrate within large river systems, like the Clanwilliam sandfish which moves from lowland rivers into small headwaters of South Africa’s Cederberg Mountains to spawn. The dorado or Goliath catfish migrates more than 11,000 km from spawning habitats in the high Andes to nursery areas in the Amazon River mouth.
An 80% decline in five decades:
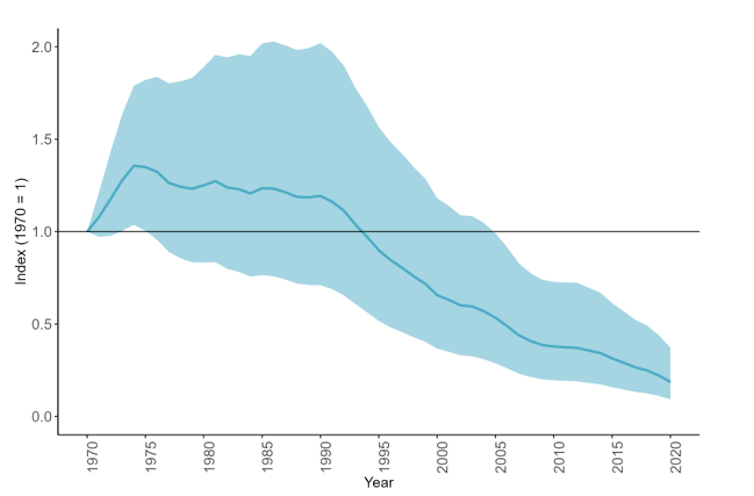
That’s why it’s crucial to maintain as many free-flowing rivers as possible to allow species and nutrients to pass from upstream headwaters to the floodplains, deltas and estuaries downstream. Yet growing demand for renewable energy means there are at least 3,500 hydropower dams under different stages of development around the world, which, when complete, will nearly double the number of large dams on Earth.
There are already over a million river barriers in Europe alone, and at least 16 million worldwide. Only around a third of the world’s longest rivers remain free flowing and less than a quarter flow uninterrupted into the open ocean. Once the new hydroelectric dams are all completed, 93% of the rivers on Earth are expected to be classified as moderately to severely fragmented.
Unintended consequences
With barriers now blocking their natural routes, no wonder there has been a catastrophic decrease in these migratory freshwater fish. According to the new report, the most alarming losses are in Latin America and the Caribbean, where populations are less than a tenth of what they were in 1970.
This region has a high reliance on hydropower electricity and is threatened by increasing drought risk, but millions of people directly rely on these fish for food, livelihoods and traditional culture. The global thirst for renewable, “clean” electricity might therefore lead to unintended consequences.
While a single dam can cause serious disruptions, barriers act in tandem with other major stressors to cause even more devastating effects. Barriers increase water temperatures, compounding trends driven by climate warming. Warmer water holds less oxygen and puts fish under stress. And then those same barriers make it harder for fish to leave uninhabitable areas for cooler water upstream.
Species which are adapted to fast-flowing, highly oxygenated cool water are overwhelmingly adversely affected and rapidly disappear from rivers impacted by barriers. Dams also trap sediments, which then alters habitats used by fish , as well as making it easier for non-native invasive species to establish.
Down with dams
One river in the US is showing a possible way forward. In an unprecedented operation to rectify the ecological damage caused by damming, six dams along 400 miles of the Klamath River in Oregon are being removed .
The Klamath River will run free, with sections having water flow after being dry for almost 100 years. Migratory salmon and steelhead trout will once again swim freely throughout the river basin, Indigenous communities will have access to traditional fisheries, and water quality will be restored.
There are options short of full dam-removal. In many parts of the world, dam operations are also being modified in attempts to make the water flow more naturally .
It may be possible to remediate some of the catastrophic impact river fragmentation has had on migratory fish species. However, this will depend on major and urgent engineering interventions, that effectively balance the need for water storage in reservoirs with human needs for freshwater biodiversity .
- Freshwater biology
- Hydroelectric dam
- Give me perspective

Data Manager

Research Support Officer

Director, Social Policy

Head, School of Psychology

Senior Research Fellow - Women's Health Services
- Essay Database
- world trade center
- Greek Food and Culture
- The Future Portrayed I
- Intercultural Communications
- In Heart of Darkness,
- Things Fall Apart by C
- In J.M. Coetzee's Wait
- The Criminals Of Profe
- Socialization of Children
- The Poet of Nature, Wi
- Leonhard Euler
- Articles of Confederat
- About all Sharks
- Vietnam Poetry
Biodiversity
What is paper-research.
- Custom Writing Service
- Terms of Service
- Privacy Policy
- Biographies

IMAGES
VIDEO
COMMENTS
Species diversity is used to refer to dissimilitude of organisms in a given region. Loss of Biodiversity and Extinctions. It is estimated that the number of species that have become extinct is greater than the number of species that are currently found on earth. Climate Change's Negative Impact on Biodiversity.
Biodiversity is the variety of life, including variation among genes, species and functional traits. It is often measured as: richness is a measure of the number of unique life forms; evenness is ...
Loss of biodiversity appears to influence ecosystems as much as climate change, pollution and other major forms of environmental stress. A healthy and balanced ecosystem is maintained by a diverse ...
Biodiversity is the variety of life, including variation among genes, species and functional traits. It is often measured as: richness is a. measure of the number of unique life forms; evenness is ...
Climate change ultimately drives terrestrial biodiversity loss and affects ecosystem carbon storage both directly and indirectly via land use change, i.e. climate change-driven cropland expansion. Molotoks et al. use a modelling approach to explore uncertainties in projections of biodiversity and carbon loss and find that, in spite of large ...
Paul R. Ehrlich* and Robert M. Pringle. Department of Biological Sciences, Stanford University, Stanford, CA 94305. The threats to the future of biodiversity are many and well known. They include habitat conversion, environmental toxification, cli-mate change, and direct exploitation of wildlife, among others.
The present study investigates the impact of climate change on biodiversity loss using global data consisting of 115 countries. In this study, we measure biodiversity loss using data on the total number of threatened species of amphibians, birds, fishes, mammals, mollusks, plants, and reptiles.
Honors Thesis Appalachian State University Submitted to the Department of Government and Justice Studies ... However, biodiversity loss is an international environmental issue that is increasingly threatening the countless ecosystem services and stabilizing abilities of biologically diverse ecosystems. The drivers of biodiversity loss are ...
Halting global biodiversity loss is central to the Convention on Biological Diversity and United Nations Sustainable Development Goals 1,2, but success to date has been very limited 3,4,5.A ...
Loss of one or a few top predator species can reduce plant biomass by at least as much52 as does the transformation of a diverse plant assemblage into a species monoculture12. Loss of consumers ...
Gaucherand, Spiegelberger, Gouraud, & Kerbiriou, 2018). All dimensions of biodiversity; genetic, species, ecosystem and functional diversity are under pressure (Hugé et al., 2017). One of the major effects of biodiversity erosion has been found to be natural habitat loss and destruction as
Biodiversity loss and the risk to development gains. It is because of biodiversity's importance in maintaining (and enhancing) stable, productive and resilient natural ecosystems — and the resources and services they provide — that biodiversity loss is an important development issue. Biodiversity is not just about the.
Abstract. This bachelor thesis focuses on the biodiversity loss problematics in Southeast Asia, since it is one of the most species rich places on Earth, coupled with the highest rate of loss of species. Four biodiversity hotspots encompasses Southeast Asia which implies areas of high endemism coupled with high rates habitat loss.
The purpose of this dissertation is to make sense of two sets of reactions. On the one hand, Americans can barely lift a finger to help threatened and endangered species while on the other, they routinely come to the aid of human victims of disaster. I argue that in contrast to cases of human tragedy, for the biodiversity crisis conservationists are faced not only with the familiar yet arduous ...
Recent global reports (Díaz et al. 2019; IPBES Secretariat 2019; CBD 2020) have rigorously synthesized the large scientific literature on biodiversity and have identified major knowledge gaps.These gaps include large uncertainties in how many species are threatened with extinction (Díaz et al. 2019; CBD 2020; IUCN 2020), a lack of estimates for the impacts of global biodiversity loss on ...
UK biodiversity policy is currently based on a 'Post-2010 Biodiversity Framework' designed to achieve the global Aichi Biodiversity Targets, agreed in 2010 by 196 countries to halt the loss of biodiversity globally by 2020, at a UK level. The framework is supported by the Joint Nature Conservation Committee.
Environmental degradation stemming from anthropocentric causes threatens the biodiversity more than ever before, leading scholars to warn governments about the impending consequences of biodiversity loss (BL). At this point, it is of great importance to study the public's conceptions of BL in order to identify significant educational implications. However, a review of the literature reveals ...
Although there is ample theoretical evidence of the economic and social causes of biodiversity loss, empirical evidence for most of these relationships is fragmented, meager or non-existent. ... the thesis argues that loss of biodiversity not a problem in itself. Rather it is a symptom of the underlying problem rooted in human thinking, guided ...
Habitat loss and fragmentation is considered as one of the main causes of biodiversity loss worldwide (Rands et al. 2010; Barnosky et al. 2011;Hilty et al. 2020), threatening with extinction over ...
Loss of Biodiversity Biodiversity Loss and Mitigation This essay will discuss the environmental citizenship concept and the different theoretical debates in the context of loss of biodiversity as well as its mitigation: ... Thesis Statement: Present a clear thesis that outlines your perspective on global warming, its impacts, and potential ...
Full size image. When summed across all terrestrial ecoregions, biodiversity declines led to loss of 7.40-102.68 (29.55 using b = 0.26 and z = 0.25) PgC of vegetation carbon in the long term ...
1. Introduction. The United Nation's Sustainable Development Goals (SDGs), alongside the Convention for Biological Diversity's 2020 Strategic Plan, lay out an ambitious conservation agenda [1,2].Achieving these goals (i.e. SDG 14, 15; Aichi targets) will require coordination among multiple stakeholders, including conservation scientists, industry and cross-sectoral decision-makers, to ...
Disclosure statement. ... causes of biodiversity loss worldwide. River fragmentation is a key reason migratory fish populations have declined by 81% since 1970, ...
Carbon sequestration and biodiversity are tightly linked, but many models projecting carbon storage change do not account for the role biodiversity plays in the sequestration capacity of terrestrial ecosystems. Here, we link a macroecological model projecting changes in vascular plant richness with empirical biodiversity-biomass stock relationships, to assess the consequences of plant biodiversity
Biodiversity. Biodiversity in an ecosystem refers to all member species in an environment. Each member has an important role to play within the ecosystem and the lack of any member can have negative consequences for the whole. It also includes all the different habitats, biological communities, and ecological processes within the ecosystem.