No item found for the identifier
Handle: 10919/9291/browse.
Take me to the home page

Home > GRADSCHOOL > GRADSCHOOL_THESES > 5655

LSU Master's Theses
Using 2d hec-ras modeling with vertical feature extraction to inform ecological design in the lower atchafalaya river basin, louisiana.
Colin Anderson , Louisiana State University and Agricultural and Mechanical College Follow
Semester of Graduation
Master of Science (MS)
Civil and Environmental Engineering
Document Type
A 2D HEC-RAS model was created to demonstrate applications of hydraulic modeling for informing ecological design. This was done by comparing the hydrologic conditions of a baseline year (2010) to that of the environmental flow prescription from Kozak et al. (2016). The inundation results are relevant to hydraulic connectivity and cypress regeneration.
The hydraulic model was created by assimilating numerous data sources, refining the model with vertical feature (VF) extraction, and calibrating/validating the model. Several topo-bathymetric sources were combined to create the digital elevation model (DEM). Most importantly, this included bathymetry of the backswamp. Polylines of significant VFs in the DEM, such as natural levees, were provided by Dr. Gao. Cell faces of the HEC-RAS mesh (where flow is calculated) were aligned with the VFs. Calibration of a low flow and high flow period was conducted so the model could replicate the hydrodynamics at all times of the year. The model was validated using the 2010 hydrograph.
Different VF and cell mesh sizes were simulated to explore the influence on this model’s performance. The VF and cell mesh sizes were 30 m and 9 m and located in a refinement region. No significant difference in model accuracy or inundation extent was found between the different VF and mesh sizes.
The hydrologic results of 2010 were compared to the prescribed flow results. The area that was inundated in the spring and then experienced 100 or more consecutive dry days in the growing season is assumed to be the most suitable for cypress regeneration. In 2010 that area was 31.0 km 2 , or 6.7% of the study area. The prescribed flow for an average year increased this to 100.8 km 2 (21.9%). If an extreme low flow event were to occur, that would increase the area to 283.8 km 2 .
Simulation results for 2010 that are similar to Kozak et al. (2016) flow-ecology targets (flows that produce expected ecological services) were extracted. It was found that overbank flooding of the Gulf Intracoastal Waterway occurred during the Overbank Flooding and Backswamp Flooding flow-ecology targets. Widespread overbank flooding did not occur along the Atchafalaya River main channel.
Recommended Citation
Anderson, Colin, "Using 2D HEC-RAS Modeling with Vertical Feature Extraction to Inform Ecological Design in the Lower Atchafalaya River Basin, Louisiana" (2022). LSU Master's Theses . 5655. https://repository.lsu.edu/gradschool_theses/5655
Committee Chair
Willson, Clinton
10.31390/gradschool_theses.5655
Since September 13, 2022
Included in
Environmental Engineering Commons , Hydraulic Engineering Commons , Other Civil and Environmental Engineering Commons
Advanced Search
- Notify me via email or RSS
- Collections
- Disciplines
Author Corner
- Submit Thesis
SPONSORED BY
- LSU Libraries
- LSU Office of Research and Economic Development
Home | About | FAQ | My Account | Accessibility Statement
Privacy Copyright
- Bibliography
- More Referencing guides Blog Automated transliteration Relevant bibliographies by topics
- Automated transliteration
- Relevant bibliographies by topics
- Referencing guides
Dissertations / Theses on the topic 'HEC-RAS modeling'
Create a spot-on reference in apa, mla, chicago, harvard, and other styles.
Consult the top 36 dissertations / theses for your research on the topic 'HEC-RAS modeling.'
Next to every source in the list of references, there is an 'Add to bibliography' button. Press on it, and we will generate automatically the bibliographic reference to the chosen work in the citation style you need: APA, MLA, Harvard, Chicago, Vancouver, etc.
You can also download the full text of the academic publication as pdf and read online its abstract whenever available in the metadata.
Browse dissertations / theses on a wide variety of disciplines and organise your bibliography correctly.
Ma, Yan M. Eng Massachusetts Institute of Technology. "Uganda Manafwa River early flood warning system development hydrologic watershed modeling using HEC-HMS, HEC-RAS, ArcGIS." Thesis, Massachusetts Institute of Technology, 2013. http://hdl.handle.net/1721.1/82819.
Alzahrani, Abdulaziz S. "Application of Two-Dimensional Hydraulic Modeling in Riverine Systems Using HEC-RAS." University of Dayton / OhioLINK, 2017. http://rave.ohiolink.edu/etdc/view?acc_num=dayton1493135117254329.
Mowinckel, Erland Kragh. "Flood Capacity Improvement of San Jose Creek Channel Using HEC-RAS." DigitalCommons@CalPoly, 2011. https://digitalcommons.calpoly.edu/theses/583.
Cronander, Joel. "Återskapande av naturliga trösklar i mindre vattendrag och våtmarker : Modellutveckling för simulering av förändradeflödesregimer." Thesis, Uppsala universitet, Institutionen för geovetenskaper, 2017. http://urn.kb.se/resolve?urn=urn:nbn:se:uu:diva-334275.
Silva, Thiago Alves da. "Modeling of Hydraulic Canal River Granjeiro, Crato / Ec, using the Computer Program HEC-RAS." Universidade Federal do CearÃ, 2014. http://www.teses.ufc.br/tde_busca/arquivo.php?codArquivo=11664.
Fang, Yanhui. "Flood Forecasting via a Combination of Stochastic ARIMA Approach and Deterministic HEC-RAS Modeling." Ohio University / OhioLINK, 2015. http://rave.ohiolink.edu/etdc/view?acc_num=ohiou1449142353.
Decina, Thiago Galvão Tiradentes. "Análise de medidas de controle de inundações a partir da avaliação de cenários de uso e ocupação do solo na bacia hidrográfica do Córrego do Gregório, São Carlos - SP." Universidade de São Paulo, 2012. http://www.teses.usp.br/teses/disponiveis/18/18138/tde-30082012-111216/.
Evanoff, Elizabeth Nicole. "Assessment of causal mechanisms on flood conveyance on the Tisza River, Hungary using one-dimensional retro- and scenario-modeling." OpenSIUC, 2010. https://opensiuc.lib.siu.edu/theses/263.
Bayareddy, Venkata Subbarao. "Determination of Ineffective Flow Areas in Bridge Modeling Using HEC-RAS by Locating Ineffective Flow Stations." University of Dayton / OhioLINK, 2016. http://rave.ohiolink.edu/etdc/view?acc_num=dayton1462356833.
Åberg, Isabelle. "Flood Mapping: Assessing the uncertainty associated with flood inundation modelling. A case study of the Mora River, Sweden." Thesis, Uppsala universitet, Institutionen för geovetenskaper, 2017. http://urn.kb.se/resolve?urn=urn:nbn:se:uu:diva-315687.
Castro, Bolinaga Celso Francisco. "Hydraulic Modeling of a River Network for Predicting Flood Inundation using HEC-RAS and GIS Models - A Case Study in Southern Virginia." Thesis, Virginia Tech, 2012. http://hdl.handle.net/10919/49555.
Miranda, Olga Lopes. "Comparação dos modelos hidráulicos HEC-RAS e LISFLOOD-FP na avaliação do risco de inundação num troço do rio Lis." Master's thesis, Universidade de Évora, 2011. http://hdl.handle.net/10174/15037.
Martinaitis, Erikas. "Akmenos upės potvynio bangos, sukeltos griūvant Padvarių užtvankai, modeliavimas Kretingos mieste." Master's thesis, Lithuanian Academic Libraries Network (LABT), 2009. http://vddb.library.lt/obj/LT-eLABa-0001:E.02~2009~D_20090603_100305-21229.
Correia, Evaldo Flávio Gomes. "Modelagem hidrológica da bacia hidrográfica do rio Bengalas, Nova Friburgo, RJ, utilizando o potencial de geotecnologias na definição de áreas de risco de inundação." Universidade do Estado do Rio de Janeiro, 2011. http://www.bdtd.uerj.br/tde_busca/arquivo.php?codArquivo=3736.
Appleby, Christina. "Modeling Historical Meander Bends Reconnection on the lower Long Tom River in Lane Co. and Benton Co., OR." Thesis, University of Oregon, 2016. http://hdl.handle.net/1794/20717.
Alvarez, Montero Gabriela Fiamma, and Cueva Carlos Alvaro Moreno. "Modelamiento hidráulico para el control y prevención de inundaciones mediante el uso de Hec-Ras 2d en la zona urbana del río Piura." Bachelor's thesis, Universidad Peruana de Ciencias Aplicadas (UPC), 2021. http://hdl.handle.net/10757/656880.
Aldred, Jennifer L. "The Effects of Late Holocene Climate Changes on Flood Frequencies and Magnitudes in Central Appalachia." Ohio University / OhioLINK, 2010. http://rave.ohiolink.edu/etdc/view?acc_num=ohiou1282188774.
Gurung, Tshering T. "One-Dimensional Dynamic Modeling of the Lower Mississippi River." ScholarWorks@UNO, 2014. http://scholarworks.uno.edu/td/1804.
Fini, Massimiliano. "Hydraulic modeling of the Panaro river for flood hazard investigation." Master's thesis, Alma Mater Studiorum - Università di Bologna, 2021.
Thurkettle, Sara. "A Paleoflood Assessment of the Greenbrier River in Southeast, West Virginia, U.S.A." Ohio University / OhioLINK, 2019. http://rave.ohiolink.edu/etdc/view?acc_num=ohiou1554904220305813.
Koppel, David W. "Changes in Flooding and Flood Protection Along a Channelized Reach of the Hocking River, Athens, Ohio." Ohio University / OhioLINK, 2011. http://rave.ohiolink.edu/etdc/view?acc_num=ohiou1306859886.
Preston, Olivia. "2D Effects of Geomorphology and Discharge on Hyporheic Exchange—a HEC-RAS Modelling Study." Thesis, KTH, Hållbar utveckling, miljövetenskap och teknik, 2020. http://urn.kb.se/resolve?urn=urn:nbn:se:kth:diva-284150.
Mousavi, Moghaddam Seyedali. "Inundation mapping of urban areas in case of severe rainfall events using HEC-RAS." Master's thesis, Alma Mater Studiorum - Università di Bologna, 2021.
Jonušaitis, Karolis. "Reguliuoto upelio ruožo hidrodinaminis modeliavimas." Master's thesis, Lithuanian Academic Libraries Network (LABT), 2011. http://vddb.laba.lt/obj/LT-eLABa-0001:E.02~2011~D_20110607_115053-48393.
Štěpánová, Markéta. "Vyhodnocení povodňové situace na vybrané části toku." Master's thesis, Vysoké učení technické v Brně. Fakulta stavební, 2017. http://www.nusl.cz/ntk/nusl-265428.
Gällstedt, Frida. "Transport av förorenat sediment i Eskilstunaån." Thesis, Uppsala universitet, Luft-, vatten och landskapslära, 2019. http://urn.kb.se/resolve?urn=urn:nbn:se:uu:diva-392763.
Ahonen, Jani. "Can morphologic restoration of hydropower outlet channels create hydraulically suitable spawning and larvae habitats for grayling? : Modelling the effects of environmental measures with HEC-RAS." Thesis, Umeå universitet, Institutionen för ekologi, miljö och geovetenskap, 2018. http://urn.kb.se/resolve?urn=urn:nbn:se:umu:diva-144188.
Ortiz, Peña Victor Alejandro, and Milos Dinic. "Satellite derived data to support flood modelling : An application to the Po River." Thesis, KTH, Hållbar utveckling, miljövetenskap och teknik, 2018. http://urn.kb.se/resolve?urn=urn:nbn:se:kth:diva-230889.
Negretto, Giacomo. "The impact of spatial representation on flood hazard assessment: a comparison between 1D, quasi-2D and fully 2D hydrodynamic models of Rio Marano (Rimini)." Master's thesis, Alma Mater Studiorum - Università di Bologna, 2019. http://amslaurea.unibo.it/17600/.
Andersson, Nyberg Adrian. "Combining hydrologic modelling and boundary shear stress estimates to evaluate the fate of fine sediments in river Juktån : Impact of ecological flows." Thesis, Umeå universitet, Institutionen för ekologi, miljö och geovetenskap, 2018. http://urn.kb.se/resolve?urn=urn:nbn:se:umu:diva-145948.
Kostelecký, Jiří. "Numerické modelování přepadu přes nouzové přelivy." Master's thesis, Vysoké učení technické v Brně. Fakulta stavební, 2016. http://www.nusl.cz/ntk/nusl-240040.
Patel, Kunal P. "Watershed modeling using HEC-RAS, HEC-HMS, and GIS models a case study of the Wreck Pond Brook Watershed in Monmouth County, New Jersey /." 2009. http://hdl.rutgers.edu/1782.2/rucore10001600001.ETD.000051392.
Hanmaiahgari, P. R., N. R. Gompa, D. Pal, and Jaan H. Pu. "Numerical modeling of the Sakuma Dam reservoir sedimentation." 2018. http://hdl.handle.net/10454/14622.
Winter, Heather. "Analysis and Prediction of Rainfall and Storm Surge Interactions in the Clear Creek Watershed using Unsteady-State HEC-RAS Hydraulic Modeling." Thesis, 2012. http://hdl.handle.net/1911/64693.
Lowrey, Maria Rose Knebl. "Regional scale flood modeling and watershed investigation, using NEXRAD rainfall, GIS, and HEC-HMS/RAS: a case study for the San Antonio River Basin, Texas." Thesis, 2006. http://hdl.handle.net/2152/3415.
Zelíková, Nikol. "Potenciál revitalizačních opatření vodních toků jako nástroje pro retenci vody v říční nivě." Master's thesis, 2019. http://www.nusl.cz/ntk/nusl-411459.
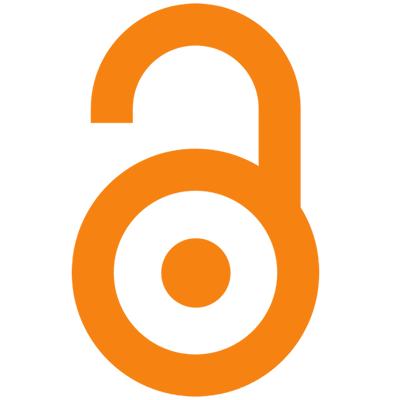
- Previous Article
- Next Article
INTRODUCTION
Methodology, results and discussion, summary and conclusions, acknowledgements, conflict of interest, data availability statement, urban flood risk analysis of buildings using hec-ras 2d in climate change framework.
- Article contents
- Figures & tables
- Supplementary Data
- Open the PDF for in another window
- Guest Access
- Cite Icon Cite
- Permissions
- Search Site
R. Madhuri , Y. S. L. Sarath Raja , K. Srinivasa Raju , Bonagiri Sai Punith , Kondisetti Manoj; Urban flood risk analysis of buildings using HEC-RAS 2D in climate change framework. H2Open Journal 1 January 2021; 4 (1): 262–275. doi: https://doi.org/10.2166/h2oj.2021.111
Download citation file:
- Ris (Zotero)
- Reference Manager
The present study aims to assess flood depth, building risk analysis, and the effectiveness of various flood adaptation strategies to attenuate building risk caused by urban floods in climate change scenarios. A framework is proposed where a hydraulic model, Hydrologic Engineering Center's-River Analysis System 2D (HEC-RAS 2D), is applied for 2-dimensional flood modeling to estimate (a) submerged areas, (b) flood depth, and (c) building risk for extreme events corresponding to two representative concentration pathways (RCPs), 6.0 and 8.5. Greater Hyderabad Municipal Corporation (GHMC), India, is chosen for demonstration. Percentages of buildings in GHMC under high, medium, and low risks for RCP 6.0 are 38.19, 9.91, and 51.9% in the respective order, and these are 40.82, 10.55, and 48.63% for RCP 8.5. Six flood proofing (FP) strategies (S1–S6) are proposed for attenuating building risk along with the required capital cost. The capital investment required for FP to achieve the ideal situation of no risk for all buildings (strategy S6) works out to Rs. 3,740 × 10 7 and Rs. 3,800 × 10 7 for RCPs 6.0 and 8.5. It is observed that the effect of adaptation strategies is significant.
Generation of flood inundation maps using Hydrologic Engineering Center's-River Analysis System 2D (HEC-RAS 2D) for the urban catchment of GHMC under two representative concentration pathways (RCPs).
Building risk analysis and formulation of adaptation strategies under two RCPs.
Over the past 30 years, urban floods occurring in catchments have been the more prominent natural disasters, which account for almost 43% ( EM-DAT, CRED 2015 ). This can be due to low-lying areas, sedimentation in drainages, clogged drains, and imperviousness of surfaces due to increased urbanization. In addition, natural streams deviated from the original flow paths, resulting in excessive urban runoff ( Ahmed et al. 2013 ; Liu et al. 2019a ). Another dimension of urbanization is the enormous increase in the number of buildings that experience different levels of alarming risk during floods. Building risk maps should be developed for different rainfall scenarios, including climate change-related, to assess this complex flood situation. These will help prioritize areas for mitigation measures and serve as a guide map to the policymakers.
The most important parameters for developing building risk maps are elevated terrain levels, contour maps, and corresponding submerged areas. The area under submergence is the extent of inundation in terms of the percentage of area occupied. However, it does not provide information about the water depth above the ground level. Flood depth varies from location to location based on the terrain–rainfall interaction. Risk estimation can be quantified on flood depth ( Rangari et al. 2019 ). The higher the flood depth, the more risk of damage to ecology, roads, buildings, and infrastructure. This makes flood depth analysis a distinct necessity. However, flood depth alone does not serve the purpose while analyzing the infrastructural damage. For example, high flood depth in uninhabited areas does not cause much damage to infrastructure compared to buildings with low to medium flood depth. Hence, flood depth levels around buildings and flood inundation maps can be used to identify locations where buildings require more maintenance than usual.
Accordingly, the present study aims to assess flood depth, building risk analysis, and effectiveness of various flood adaptation strategies to attenuate building risk caused by urban floods in a climate change scenario. For demonstration, we have chosen Greater Hyderabad Municipal Corporation (GHMC), India. GHMC witnessed many flood events in the past, resulting in damage to property. This necessitated studying the response of GHMC toward extreme rainfalls for flood preparedness. Many studies were performed in the past for hydraulic modeling of urban floods for producing flood inundation depth, flood risk maps, and depth damage relationships. Some of the relevant literature reviews are as follows.
Mosquera-Machado & Ahmad (2007) evaluated flood hazard (FH) for Atrato River, Quibdó, northwest Colombia. They employed geographic information systems (GIS), statistical approaches, and Hydrologic Engineering Center's-River Analysis System (HEC-RAS) for evolving three FH maps having different return periods (RPs). Water depths on the left and right banks were 3.7 and 3.1 m for a 50-year RP, which could be used as the basis for designing flood protection structures. Fan et al. (2009) applied Qual2 K with HEC-RAS 1D for assessing the water quality of a tidal river, northern Taiwan, and the models exhibited good agreement. Timbadiya et al. (2011) employed HEC-RAS for the lower Tapi River to simulate the flow for 1998, 2003, and 2006. Simulated flows were closely matched with the observed data. Qasim (2013) simulated free flow over the broad-crested single-step weir using HEC-RAS 1D. Simulated and observed flow profiles were matched with reasonable accuracy. Klimeš et al. (2014) simulated hydrological characteristics of floods due to glacial lake outburst using HEC-RAS 1D along the Chucchun River, Peru, resulting in a simulated peak discharge of 580 m 3 /s. Authors have only considered 1D flow (depth) for flood analysis but have not applied 2D flow (depth and flood extent for submergence areas) using HEC-RAS 2D.
Quirogaa et al. (2016) applied HEC-RAS 2D to simulate the flood events of February 2014 for a case study of Llanos de Moxos, Bolivian Amazonia. They verified the same with available satellite images. Bhandari et al. (2017) used HEC-RAS 2D in a lower region of the Brazo River watershed, Richmond, TX. Maximum water depth and flood velocities were 13.1 m and 16.1 m/s, respectively. An increase in flooding was expected in climate change scenarios. Song et al. (2017) investigated the effect of Manning's roughness coefficient in HEC-RAS 1D for a case study of the German lowland area. They found that the roughness coefficient played a significant role in enhancing the model output's quality. Vojtek et al. (2019) evaluated the sensitivity of the event-based approach for small and ungauged basins using HEC-RAS 1D. They simulated flood area and flood volume and stressed the importance of roughness and cross-section parameters, besides excess rainfall determination for effective mitigation. Shustikova et al. (2019) compared LISFLOOD-FP (Local Inertia Solver for FLOOD across Flood Plain) and HEC-RAS (River Analysis System) for Secchia River, Italy. HEC-RAS performed better than LISFLOOD-FP for all resolutions except for a 25-m resolution. Kumar et al. (2020) employed the Global Flood Monitoring System and HEC-RAS 2D for the confluence of Ganga and Yamuna, Prayagraj, India. They identified critical and most critical areas based on a 100-year RP. The authors used Manning's roughness coefficient to enhance the model output quality in river basin analysis. However, no studies are reported in urban areas.
Rangari et al. (2019) applied HEC-RAS 2D to analyze flood inundation depths for different rainfall scenarios for zone 13 of GHMC. Seventeen percent of the total catchment area was likely to flood, of which 9% was under high risk. Surwase & Manjusree (2019) assessed the Storm Water Management Model (SWMM), HEC-RAS 2D, and Height Above the Nearest Drainage (HAND) for simulation of urban flooding for zone 12 of GHMC. All the models complemented each other. They determined the depth of the stream. None of them used the GHMC area nor the climate change impacts in their study.
Swathi et al. (2020) employed SWMM and HEC-RAS 2D to assess the impacts of land use land cover (LULC) and climate change on runoff in GHMC. Madhuri et al. (2021) applied five machine learning algorithms to a case study of GHMC under four representative concentration pathways (RCPs) for predicting flood risk probabilities, where XGBoost was found superior. The mentioned works did not focus on flood depth, building risk analysis, and prioritized areas under climate-based scenarios.
Dau & Kuntiyawichai (2015) simulated flood risks due to climate change in Central Vietnam. They used the global climate model (GCM), namely, HadCM3, for A2 and B2. A potential increase in runoff and water levels was observed. Shrestha & Lohpaisankrit (2016) assessed the FH potential based on three GCMs in Yang River Basin of Thailand using TOPMODEL and HEC-RAS 1D. The total flooded area was found to increase for RCP 4.5 as compared to the baseline period. Mondal et al. (2018) applied SWAT, HEC-RAS, and Delft3D to three major rivers of Bangladesh under RCP 8.5. An increase in peak flood levels by 25–72 cm was expected by this century's end. Dau & Kuntiyawichai (2020) assessed the climate change impacts on the operations of a reservoir in Huong River Basin, Vietnam, for RCP 8.5. They coupled HEC-HMS and HEC-RAS 2D. Mean rainfall, mean water level, mean annual runoff, and temperature would increase in the future. Hanif et al. (2020) examined flood risks for 2030 and 2050, respectively, under RCPs 4.5 and 8.5 using HEC-HMS and HEC-RAS. The study also provided potential adaptation strategies. Malik & Pal (2021) analyzed the flood susceptibility of the lower Dwarkeswar River for historical and future floods for one GCM using the HEC-RAS rain-on-grid model. They concluded that Weibull's method was the best suited for flood susceptibility mapping. These studies could not address the need for better adaptation strategies required to mitigate the risk of future floods.
Aerts et al. (2018) computed the associated costs of various adaptation measures for coping with sea-level rise in coastal LA County. Adaptation costs range between $4.3 and $6.4 billion. de Ruig et al. (2020) combined cost–benefit analysis (CBA) and developed a flood risk model for Naples and Venice Beach in Long Beach and Los Angeles. An optimal mix of 35–45 and 55–65% of dry-flood proofing (FP) and building elevation measures was proposed. Ventimiglia et al. (2020) developed a methodology for determining an optimal combination of FP measures using CBA for the Mela river, northeastern Sicily. Results supported the possibility of decreasing the damage caused due to floods by implementing FP measures. Han (2021) evaluated building-level adaptation strategies by incorporating the latest information on the sea-level rise through a dynamic programming-based CBA analysis for Bay County, Florida. He concluded that investing in adaptive measures decreased the average annual damage. The studies mentioned did not take into consideration the exclusive FP measures based on building risk analysis.
Building risk analysis based on flood depth
Adaptation measures for buildings at risk
Hence, the objectives chosen for GHMC are (a) to develop flood inundation maps and identify the submerged areas for RCPs 6.0 and 8.5, using HEC-RAS 2D, (b) to compute building risk analysis based on flood depth, and (c) to formulate suitable adaptive measures and assess their effectiveness for attenuation of building risk in the urban catchment.
The following sections discuss the methodology, study area description, data collection and processing, and description of HEC-RAS 2D along with calibration and validation, results & discussion, and summary of the work.
HEC-RAS 2D was calibrated and validated using the National Remote Sensing Centre (NRSC) flood vulnerability maps and flood depth data. Two extreme event rainfalls corresponding to RCPs 6.0 and 8.5 were simulated using calibrated HEC-RAS 2D to generate flood inundation maps. These contain information related to submergence areas and flood depths at all locations of GHMC. Flood depths at the building locations were obtained by overlaying the GHMC building shapefile with the flood inundation map in Arc GIS. The resulting building risk map emphasizes classifying buildings into three risk categories based on corresponding flood depths. In addition, six FP strategies were also defined, and the positive impacts of their implementation were assessed. Investment required and the equivalent annual costs (EAC) for the implementation were also computed for each building category.
Study area, data collection, and processing
The catchment area of GHMC, India, is 625 km 2 . It consists of 16 zones based on a stormwater drainage network, as shown in Figure 1 . Elevations of the area fall between 462 and 635 m from mean sea level. Higher elevations are found in zones 9 and 11, the northern parts of zones 12, 14, and 7. Similarly, lower elevations are found near the Musi river, especially in the southern part of zones 1, 4, 5, 6, 12, 13, and 15. The river Musi flows between the catchment area from west to east. All natural streams drain the water to the Musi river, the main tributary of the Krishna River Basin.

DEM/pictorial representation of zones in GHMC.
Several factors affect the flooding process, which include terrain, slope, LULC, and imperviousness. Variation in these factors leads zones 1, 2, 3, 4, 6, and 7 to more flooding. With more imperviousness percentage area, the water holding capacity of soil decreases, resulting in excess water on the surface, causing inundation. LULC map is shown in Figure 2 (refer to Table 1 for more details). A significant amount of data is necessary to facilitate satisfactory flood risk analysis of buildings. Table 1 presents information about significant data collected/processed, including rainfall (historic and climate-based), digital elevation model (DEM), percentage imperviousness land use, LULC, and cost/m 2 for water proofing (WP).
Information on data collected and their sources

LULC map of GHMC.
Description of HEC-RAS 2D, calibration and validation
In the present study, HEC-RAS 2D, a 2-dimensional hydraulic model, is employed. The model considers hydrodynamics, horizontal and vertical flows, and 2D flow visualization, which are not possible in a 1D model ( Quirogaa et al. 2016 ; Şensoy et al. 2018 ; Dasallas et al. 2019 ). HEC-RAS 2D also efficiently solves shallow water and diffusion wave equations ( HEC-RAS 2016 ; Liu et al. 2019b ).
The model requires rainfall, DEM as a boundary condition, and Manning's roughness coefficient derived from LULC for calibration. ASTER DEM of 30 m × 30 m resolution is processed in Arc GIS. Model is discretized into an equal number of grid cells of 30 m × 30 m, i.e., equal geometry to maintain spatial uniformity. In addition, DEM of the same resolution is adopted, as it is appropriate for hydrological modeling studies ( Buakhao & Kangrang 2016 ; Rocha et al. 2020 ; Abili 2021 ). Simultaneously, other topographical features and infrastructural layers are also made similar to maintain uniformity. This helped in making 2D flow calculations simpler.
DEM is used as an input in the RAS mapper of HEC-RAS 2D to develop Digital Terrain Model (DTM). DTM developed from DEM is also taken into account natural drainage flow. DEM data are added to the New Terrain Layer dialogue of the RAS mapper in HEC-RAS 2D. A new terrain layer is created with terrain files that are used for evaluation. This information is saved in the terrain folder in GeoTiff format. In addition to GeoTiff, two more files are created in .hdf and .vrt formats. The file .hdf is created in the RAS mapper, which contains information on the raster data. The .vrt file helps visualize and display multiple data. For visualizing flood plain, the model geometry can be coupled to DTM. This DTM acts as a basis to create a 2D mesh using the polygon shapefile consisting of 19,717 cells. The DEM and DTM are presented in Figures 1 and 3 that contain submerged area and water depths at each mesh grid. These can be used for computing the water surface elevations to visualize flood plain geometry and flood risk analysis of buildings.
A cumulative rainfall of 165 mm occurred at Qutubullapur on September 21, 2016 for 12 h is used for calibration ( Surwase & Manjusree 2019 ). Manning's value between 0.01 and 0.4 is used as input to the model during calibration ( HEC-RAS 2016 ). It is based on a combination of land-use classes and soil properties ( Dorn et al. 2014 ; HEC-RAS 2016 ).
F -Index computations for zone 12
Calibration is reasonable as understood from the average F -index value of 81% ( Quirogaa et al. 2016 ; Liu et al. 2019b ; Rahimzadeh et al. 2019 ) with Manning's value of 0.015. It is considered representative of the whole area due to uniformity in catchment characteristics in terms of sandy-loam soil.
Validation is carried out at eight locations of zone 12. Historic rainfall of 215.9 mm occurred during September 20–28, 2016 is used for validation. Simulated flood depths vary from 0 to 4 m at Nizampet, Qutubullapur, and Jeedimetla and agree with NRSC maps ( Bhatt & Rao 2018 ).
Results related to flood inundation mapping, building risk analysis based on flood depth, and corresponding adaptation measures are presented for two extreme events of RCPs 6.0 and 8.5 in this section.
Flood inundation mapping and submergence areas
After validating HEC-RAS 2D, the model facilitates flood inundation maps for extreme future rainfall events, i.e., 440.35 and 624.2 mm corresponding to RCPs 6.0 and 8.5 (refer to Table 1 ). Inundation maps are presented in Figure 4(a) and 4(b) . It is observed that areas most vulnerable to flooding are located near the Musi river, its tributaries, and Hussain Sagar (highlighted in circles on the map). GHMC-wise submergence areas for RCPs 6.0 and 8.5 are 334.23 and 357.97 km 2 , respectively, as observed from inundation maps ( Figure 4(a) and 4(b) ). This can be attributed to their higher rainfall magnitudes. Areas most vulnerable to flooding are in the northern sections of zones 1 and 5 and southern sections of zones 13 and 15.

DTM of GHMC.

Flood inundation map of RCPs (a) 6.0, (b) 8.5, (c) submerged area of all zones for RCP 6.0, and (d) submerged area of all zones for RCP 8.5.
In addition, zone-wise submergence areas and catchment areas are shown in Figure 4(c) and 4(d) for RCPs 6.0 and 8.5. Zones 3, 9, 11, and 14 were less submerged due to their high elevation levels in the ranges of 500–564, 561–602, 559–612, and 525–613 m, respectively. This ensures that water does not accumulate. Among these, zones 9 and 11 are relatively less submerged (4.77 and 7.27 km 2 for RCP 6.0; 5.43 and 8.53 km 2 for RCP 8.5).
It is observed that a higher slope leads to more inundation, as evident from Figures 1 and 4(c) and 4(d) . Submerged areas of zone 12 for RCPs 6.0 (69.2 km 2 ) and 8.5 (71.45 km 2 ) are more than those of the other zones. This is due to the higher catchment area (138.12 km 2 ) and terrain characteristics. Zones 1, 2, and 4 were found to have terrains that aid the flooding process, whereas it is vice-versa for zones 8, 9, and 11. Zones 1 (elevation level: 462–539 m) and 2 (elevation level: 492–527 m) are highly submerged (15.69 and 3.68 km 2 for RCP 6.0; 17.72 km 2 and 3.99 km 2 for RCP 8.5).
This situation is challenging for the residents in the vicinity of inundated areas of GHMC. Complimentarily, buildings were 526,545 in number with an area of 84.91 km 2 . Hence, it was felt to analyze building risk associated with flood depths. These were then categorized as low-risk buildings (LRB) with flood depth of <0.5 m, medium-risk buildings (MRB) with flood depths of ≥0.5 and <1 m, and high-risk buildings (HRB) with flood depth of ≥1 m as discussed in the following section.
Building risk analysis
Building risk analysis is carried out for all zones of GHMC. Flood depths were found to vary from zone to zone. High flood depth values near buildings were observed in zones 1, 5, 6, 7, 13, and 15; low flood depth values near buildings exist in zone 9. The percentages of HRB, MRB, and LRB for RCP 6.0 in GHMC-wise are 38.19% (201,110), 9.91% (52,144), and 51.9% (273,291), and these are 40.82% (214,943), 10.55% (55,531), and 48.63% (256,071) for RCP 8.5. Values in parentheses represent the number of buildings in that category. The zone-wise building risk analysis for RCPs 6.0 and 8.5 is depicted in Figure 5(a) and 5(b) .

(a) Zone-wise percentage of buildings under high, medium, and low risks for RCP 6.0. (b) Zone-wise percentage of buildings under high, medium, and low risks for 8.5.
It is observed from Figure 5(a) and 5(b) that for RCP 6.0, the range of zone-wise percentages of HRB, MRB, LRB, respectively, are, 19–48, 9–13, and 41–70%. The highest number of HRB was noticed in zone 1 due to its low elevation levels of 462–539 m. The lowest percentages of HRB and LRB were found in zone 9 due to its high elevation levels of 561–602 m. For RCP 8.5, the ranges of zone-wise percentages of HRB, MRB, and LRB, respectively, are 31–58, 8–12, and 34–64%. This increase in the percentage of HRB is similar to that of RCP 6.0 for zone 1. The most negligible percentage of HRB was found in zones 3 and 6. This can be due to the medium elevation (500–564 m) for zone 3 and a moderate slope of 11.3° for zone 6 where minor flooding is possible. The maximum number of LRB is found in zone 9, which is similar to RCP 6.0. The minimum number of MRB was found in zone 1 for RCPs 6.0 and 8.5.
Accordingly, suitable adaptation measures are necessary for the existing buildings, which are at risk. The adaptation strategy required to reduce damages to the buildings is discussed in the following section.
Adaptation strategies
S2 – 2 m FP for all HRBs + rooftop FP
S3 – 2 m FP for all HRBs + 1 m FP for all MRBs + rooftop FP
S4 – 1.5 m FP for all HRBs + 1 m FP for all MRBs + 0.5 m FP for all LRBs + rooftop FP
S5 – 2 m FP for all HRBs + 1 m FP for all MRBs + 0.5 m FP for all LRBs + rooftop FP
S6 – FP equal to flood depth for all buildings + rooftop FP
FP strategies and their corresponding results are shown in Table 3 .
FP strategies and their corresponding results
Notes : Column No. 11 has been rounded off to the nearest integer. 1 US$ = 74 Rs. as of August 8, 2021.
An apparent comprehension of the calculation of EAC per building can be obtained from the following example.
The total cost incurred for S2, S3, and S4 under RCP 8.5 is estimated to be Rs. 2,264 × 10 7 , Rs. 2,791 × 10 7 , and Rs. 3,152 × 10 7 , respectively. The corresponding EAC required can be estimated using Equation ( 5 ) as Rs. 322.39 × 10 7 , Rs. 397.31 × 10 7 , and Rs. 448.72 × 10 7 , respectively. However, EAC required per building is of greater significance at an individual level than the total EAC. This can be facilitated by Equation ( 6 ).
It should be noted that the denominator in Equation ( 6 ) varies across strategies depending on the number of buildings where FP is implemented. The utilization of capital cost for implementing FP results in the reclassification of buildings from higher to lower risk. Analysis was done across various strategies for RCPs 6.0 and 8.5, as shown in Table 3 , which results in the following.
Capital investment of Rs. 3,740 × 10 7 and Rs. 3,800 × 10 7 are required, respectively, for RCPs 6.0 and 8.5 for achieving the ideal situation of all buildings under no risk (strategy S6). Corresponding EAC/building is Rs. 16,874 and Rs. 16,144. Strategy S5 results in an increase in the number of NRBs by 87.42 and 104.87% for RCPs 6.0 and 8.5, respectively, employing EAC/building of Rs. 14,360 and Rs. 14,298. HRBs decrease from 38.19 to 16.69% and 40.82 to 16.2%, respectively, for RCPs 6.0 and 8.5. RCP 8.5 results in shifting 21,244 from HRB to MRB; 27,912 from HRB to LRB; and 80,471 from HRB to NRB with Rs. 2,264 × 10 7 for strategy S2. The implementation of strategy S3 requires an amount of Rs. 2,791 × 10 7 , which is Rs. 526 × 10 7 more than S2 under RCP 8.5. The implementation of strategy S4 for RCP 8.5 leads to a decrease in the number of HRBs, MRBs, and LRBs by 50.42, 49.73, and 45.36%, respectively, and an increase in NRBs by 86.40% by expecting investment, EAC/building of Rs. 3,152 × 10 7 and Rs. 13,388, respectively.
Overall, the application of FP is an effective measure for flood adaptation, ensuring preparedness toward urban floods. Effective implementation of FP can help decrease the vulnerability of building risk and increase the life span. Additional benefits are the incentives provided in the form of flood insurance. Such schemes will encourage the city dwellers to participate in FP actively. This can also be added to the existing government schemes as a policy, thereby reducing the fear of floods and resultant infrastructural damages.
The problem of urban flooding is a complex one; hence, it is very challenging to model such situations. HEC-RAS 2D was presented for analyzing extreme future rainfall events of RCPs 6.0 and 8.5. Flood inundation maps based on buildings were studied and analyzed. The percentages of HRB, MRB, and LRB in GHMC-wise for RCP 6.0 were 38.19% (201,110), 9.91% (52,144), and 51.9% (273,291). These were 40.82% (214,943), 10.55% (55,531), and 48.63% (256,071) for RCP 8.5. Adaptation measures for the buildings at risk were also analyzed using six FP strategies. The capital investment required for FP has been calculated as Rs. 3,740 × 10 7 and Rs. 3,800 × 10 7 for RCPs 6.0 and 8.5 to achieve the ideal situation of no risk for all buildings, i.e., strategy S6. Information provided in this study will help residents of the catchment in Hyderabad to remain alert and be warned of future calamities, and combat the same in time. Future scenarios of risk maps for buildings and flood inundation maps will help the policymakers before planning any projects. The investments in strategy S6, as suggested, will be comparatively small but are certainly for better efficiencies. The contribution of this study is (a) in emphasizing flood risk of buildings by identifying the flood depths associated with each building in climate change scenarios and (b) in assessing the effectiveness of adaptation strategies for attenuating this risk.
The present work is based on chosen GCM, hydrologic model, RCPs, and costs from various sources. The outcome may vary based on different data/approaches. However, the methodology remains the same, which is the main focus of the present study. All the building conditions were considered the same here, and we wish to study in-depth by considering buildings’ life cycle/age in our future work.
The present work is supported by Information Technology Research Academy (ITRA), Government of India under ITRA-water grant ITRA/15(68)/water/IUFM/01. Acknowledgments go to GHMC officials and other agencies for their help. Special acknowledgments to Prof D. Nagesh Kumar, Dept. of Civil Engineering, IISc., Bangalore, for providing critical suggestions for improving the manuscript and Dr Swathi Vemula for providing necessary data support.
The authors declare that there are no conflicts of interest.
Data cannot be made publicly available; readers should contact the corresponding author for details.
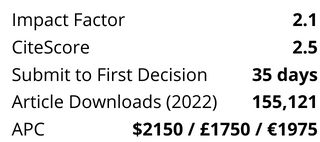
Affiliations
- EISSN 2616-6518
- Open Access
- Collections
- Subscriptions
- Subscribe to Open
- Editorial Services
- Rights and Permissions
- Sign Up for Our Mailing List
- IWA Publishing
- Republic – Export Building, Units 1.04 & 1.05
- 1 Clove Crescent
- London, E14 2BA, UK
- Telephone: +44 208 054 8208
- Fax: +44 207 654 5555
- IWAPublishing.com
- IWA-network.org
- IWA-connect.org
- Cookie Policy
- Terms & Conditions
- Get Adobe Acrobat Reader
- ©Copyright 2024 IWA Publishing
This Feature Is Available To Subscribers Only
Sign In or Create an Account
Advertisement
Advanced floodplain mapping: HEC-RAS and ArcGIS pro application on Swat River
- Original Article
- Open access
- Published: 03 April 2024
Cite this article
You have full access to this open access article
- Muhammad Ihsan Ullah ORCID: orcid.org/0009-0004-9078-9646 1 ,
- Komal Sahab Qureshi 1 ,
- Ateeq ur Rauf 1 &
- Liaqat Ali Shah 2
310 Accesses
Explore all metrics
Floods stand out as one of the most devastating environmental threats worldwide resulting in the tragic loss of human lives and significant damage to our essential infrastructure. This study focuses on creating floodplain maps for the two important reaches of Swat River in the Khyber Pakhtunkhwa Province of Pakistan, namely, Kalam–Khwazakhela and Khwazakhela–Chakdara reaches. The Advanced Land Observing Satellite Phased Array Type L-band Synthetic Aperture Radar (ALOS PALSAR) 12.5 m Digital Elevation Model (DEM) data has been used for this purpose. Furthermore, the sensitivity of the flood model was assessed for the Flood 2022 event, considering simulated flood depth, extent, and velocity in relation to various terrains derived from the 12.5-m ALOS PALSAR data. To estimate extreme flows for different return periods (2, 5, 10, 25, 50, and 100 years), the Log Normal (LN), Log Pearson III (LP3), and Generalized Extreme Value (GEV) distributions were employed for the frequency analysis. The GEV distribution turned out to be the best fit for modeling the Swat River for both the Chakdara and Khwazakhela gauge stations. To predict flood levels for the peak floods identified through frequency analysis and for the specific return periods the Hydrologic Engineering Center's River Analysis System (HEC-RAS) 2D simulations were performed. Subsequently the outcomes of this model were used to create floodplain maps using Geographic Information System Professional (ArcGIS Pro) software customized for various return periods. The analysis revealed a linear increase in flood inundation area with longer return periods. The floodplain maps developed hold significant importance for the governing authorities of the Swat region. These maps serve as essential tools for implementing proactive measures against potential infrastructure damage, thereby protecting against economic losses and enhancing public safety. This research effectively combines hydrological modeling and geospatial technology offering practical solutions for managing flood risks. It serves as a valuable guide for making well-informed decisions and promoting sustainable development in flood-prone areas.
Similar content being viewed by others
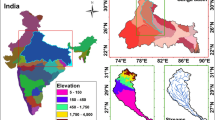
Can geomorphic flood descriptors coupled with machine learning models enhance in quantifying flood risks over data-scarce catchments? Development of a hybrid framework for Ganga basin (India)
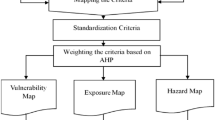
Coastal Flood Induced Salinity Intrusion Risk Assessment Using a Spatial Multi-criteria Approach in the South-Western Bangladesh
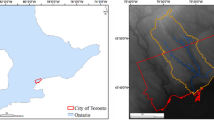

Urbanization impacts on flood risks based on urban growth data and coupled flood models
Avoid common mistakes on your manuscript.
1 Introduction
Floods are one of the most common and seriously damaging natural disasters. They causes substantial worldwide economic damages [ 1 , 2 , 3 , 4 , 5 ]. During July 2010 and July 2022 the sequence of seasonal heavy rainfall in northern Pakistan led to catastrophic floods causing significant loss of life, property and agricultural crisis [ 6 , 7 ]. To reduce flood damage it is crucial to integrate both structural and non-structural flood mitigation measures. Construction of infrastructure for protecting against flooding such as spurs, floodwalls and embankments are included in structural approaches. In contrast non-structural strategies evaluate flood risks and produce maps to make it easier to adopt efficient mitigation strategies. Relying only on structural changes is ineffective in managing reoccurring flood hazards [ 8 ]. In order to protect both people's lives and crucial infrastructure the incorporation of flood hazard mapping becomes essential as a non-structural measure [ 9 , 10 ]. Initially the flood frequency analysis was conducted to estimate extreme flows for different return periods (2, 5, 10, 25, 50, and 100 years), employing the Log Normal (LN), Log Pearson III (LP3) and Generalized Extreme Value (GEV) distribution. The Easy Fit (5.6) software was used to determine the optimal flood distribution model for calculating peak discharges over different annual recurrence intervals (ARI). Secondly an enhanced iteration of the HEC-RAS software was employed for this study. HEC-RAS stands as a preeminent model extensively employed for analyzing channel flow and accurately delineating floodplains. Its extensive use is due to its effectiveness in simulating hydraulic behavior and assisting in the evaluation of floodplains comprehensively [ 11 ]. Finally for the visual representation of the 2D models the advance version of ArcGIS Pro software was used over the ArcGIS Desktop also used previously in number of studies [ 12 , 13 ]. The ArcGIS Pro paid version and free trial is available on the Esri website [ 14 ]. Although the 1D analysis in Hec-Ras yielded successful outcomes for the specified regions within Pakistan [ 15 ] it encountered challenges in hilly areas like Swat in previous studies [ 16 ]. Accurate representation and integration of river features into digital elevation models (DEMs) play a key role in improving the overall accuracy and reliability of 2D flood models [ 17 , 18 ]. Flood hazard assessments are important tools for understanding the extent and severity of potential floods. This study includes a key analysis of two fundamental factors: flood depth, extent, and exposure factors such as land cover and land use. Accurate representation of these factors facilitates effective risk management strategies and contributes to the development of flood-resilient communities. Integrating advanced tools such as 2D flood models and geographic information systems (GIS) will further improve the accuracy and applicability of flood risk assessment techniques [ 19 , 20 , 21 , 22 , 23 ].
2 Methodology
2.1 data collection.
Runoff data were collected for a period of thirty years (1993–2022) from Peshawar Hydro-Irrigation Department. After collection the data were processed using Microsoft Excel. Digital Elevation Model (DEM) data were obtained from the Alaska ALOS PALSAR website DEM 12.5 m (PALSAR). The land cover data used in this study were obtained from the USGS website. Medium Resolution Imaging Spectroradiometer (MODIS) land cover data were downloaded (USGS, 2023). Data analysis and visualization tasks were performed using ArcGIS Pro version 2.8.3. Hydraulic modeling was performed using Hydrologic Engineering Centers-River Analysis System (HEC-RAS) version 6.3.1. Frequency analysis was performed using EasyFit software.
2.2 Study area
The Floodplain maps were developed for the two reaches Kalam to Khwazakhela and Khwazakhela to Chakdara gauge station of Swat River. Figure 1 shows the Swat River is a 240 km long perennial river in northern Pakistan that rises from the Gabral and Ushu rivers in the Kalam region. Its source lies in the Hindu Kush Mountains from which glacial water is supplied. Downstream the Kalam valley flows into the city of Madian and through the plains of the Swat valley the river flows into Chakdara. At the southernmost tip of the Swat Valley the river joins the Panjkora River and finally joins the Kabul River near Charsadda. The Basin of the Swat River lies between latitude 34° 00 and 35° 56/N and longitude 70° 59 and 72° 47/E. It has two headworks the Munda Headwork with an average discharge of 280 cumecs and the Amandala Headwork with an average discharge of 443 cumecs.
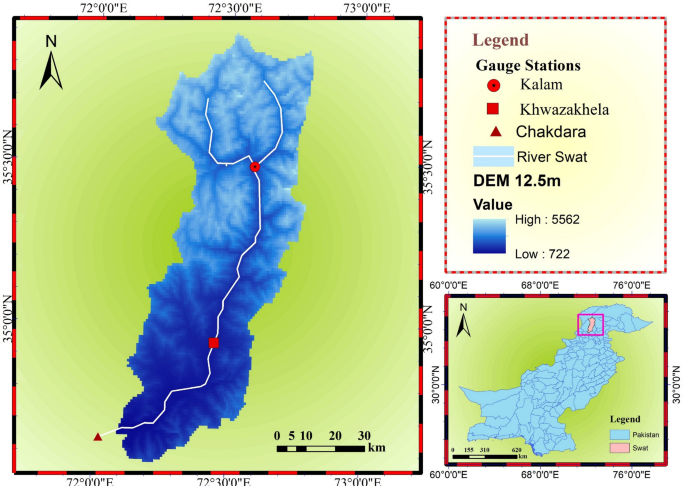
Study area map
2.3 Methodology
The methodology follows the steps of the process shown in Fig. 2 . When designing structures to reduce flooding and managing natural disasters the peak flow calculating is of utmost importance [ 24 , 25 ]. The main focuses of this study basically cover two key phases. The first focuses on frequency analysis while the second is focused on mapping floodplains. In terms of frequency analysis using the EasyFit software helped identify the peak flows associated with different Annual Recurrence Intervals (ARIs). As a result it was easier to decide which distribution analysis technique would be best for modelling and analyzing the data. The two-dimensional models used in the context of floodplain mapping. These created models were then visualized and mapped using ArcGIS Pro producing an extensive representation of the floodplain maps. The floodplains maps were developed in ArcGIS Pro, a simple and advance software tool that has capabilities that are beyond those of ArcGIS Desktop. The following consecutive procedures were carefully followed: A thorough frequency study was conducted in Step 1 of the research process using three different distribution analysis techniques. The most precise fit was determined after a thorough analysis using the EasyFit software. As a result of its availability and suitability for the study region a Digital Elevation Model (DEM) with a resolution of 12.5 m was chosen in Step 2 and obtained from the Alaska PALSAR website. In Step 3 the DEM and peak discharge data for various return periods were loaded into the HEC-RAS. Step 4 involved a thorough examination of the HEC-RAS model in order to produce an advanced 2D model that improved our understanding of flood dynamics [ 26 , 27 ]. The results of the analysis were then imported into ArcGIS Pro (Step 4) then the model was converted into a map. This mapping goes through significant refinement considering the historical context of the 2022 floods enabling an evaluation of the model's accuracy and dependability. These methodological floodplain mapping steps are used using ArcGIS Pro and HEC-RAS.

Methodology flowchart
2.3.1 Frequency analysis
The Generalized Extreme Value (GEV), Log-Pearson 3 (LP3), and Log-Normal (LN) (3-parameter) distributions were examined in the analysis of flood distribution models for the Khwazakhela and Chakdara gauge stations using the EasyFit software. The Kolmogorov–Smirnov (K–S) test, Anderson–Darling test, and Chi-Squared test were used to gauge how well these models matched the data. The Generalized Extreme Value (GEV) distribution was determined to be the best match distribution for the Khwazakhela and Chakdara gauge stations based on the findings of the Kolmogorov–Smirnov (K–S) test, Anderson–Darling test, and Chi-Squared test. This choice implies that the GEV distribution and the observed flood data at both sites are most closely aligned. The peak discharge figures produced from the GEV distribution were subsequently chosen for further use in floodplain mapping [ 28 , 29 , 30 ].
2.3.2 Hec-Ras 2d modelling
To achieve accurate floodplain mapping and hazard assessment several crucial steps are taken when doing 2D hydraulic modelling with HEC-RAS [ 31 , 32 ]. First 12.5 m resolution Digital Elevation Model (DEM) downloaded from the Alaska PALSAR website and a file with the PRJ extension is downloaded to determine the proper projection for Pakistan. The projection adjusted in Ras-Mapper to match the Pakistan coordinates after the obtained data has been imported. The preparation of the geometry which includes drawing the boundaries of the 2D flood region, comes next. For the modelling procedure a mesh with 100 × 100 cell size was used. Using the flood data from 2022 hydrographs are created and then imported into the HEC-RAS model to create the unsteady flow conditions. The unsteady flow analysis is run, producing data on the inundation depth and boundary for the flood peak discharge scenario in 2022. The model's accuracy is then confirmed by contrasting the predictions with the actual flood in July 2022 as seen through MODIS imagery. A thorough evaluation of flood hazards is ensured by the extension of this validation method to various Annual Recurrence Intervals (ARIs) including 5, 10, 25, 50, and 100 years [ 33 ]. The validated model is then used to produce floodplain maps, hazard maps, and velocity maps for the flood event in 2022 as well as the flood during the 100-year return period this offering crucial insights for flood management and disaster preparedness [ 34 ].
3 Results and discussion
3.1 flood frequency analysis.
The Khwazakhela and Chakdara gauge stations peak discharge corresponding to different return periods were the obtain using Extreme Value (GEV), Log-Pearson 3 (LP3), and Log-Normal (LN) (3-parameter) distributions. Table 1 provide Frequency Analysis Results for Chakdara Station, Table 2 provide Frequency Analysis Results for Khwazakhela Station. Table 3 and Table provide Goodness of Fit Test Results for Chakdara Station and Khwazakhela Station.
Through several statistical tests, the EasyFit software was used to perform the goodness of fit test (Table 4 ).
3.2 Advance floodplain mapping
3.2.1 models validation.
The technique used for the calculation was the diffusive wave equation and the 2022 flood event was simulated using the HEC-RAS flood model. By comparing the calculated flood extent with MODIS Imagery captured during the same flood event the model's results were validated, and their accuracy was demonstrated shown in Figs. 3 and 4 . The Swat River's Chakdara and Khwazakhela segments were the site of this validation operation. After the River Swat flood model was carefully calibrated and validated additional simulations were run to assess expected flood scenarios associated to different return periods: 2, 5, 10, 25, 50, and 100 years. The utilization of 12.5m ALOS PALSAR DEM resolution data is crucial for accurately simulating flood extents and depths in hilly and mountainous terrains. This level of detail enhances the precision of hydraulic models enabling more reliable floodplain mapping and improving our ability to predict and manage flood risks. Supported by thorough validation and comparative studies the evidence highlights the importance of choosing the 12.5m ALOS PALSAR Digital Elevation Model (DEM) as a foundational element for efficient and accurate hydraulic modeling in complex terrains emphasizing its accuracy and suitability for such applications.
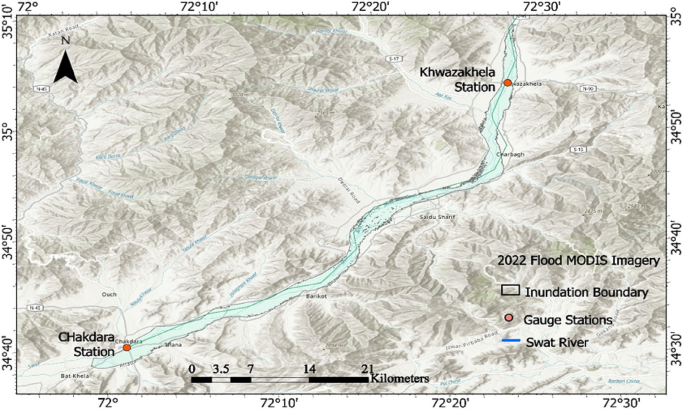
Extent of inundation observed in MODIS Imagery during the 2022 Flood event (from Khwazakhela to Chakdara)
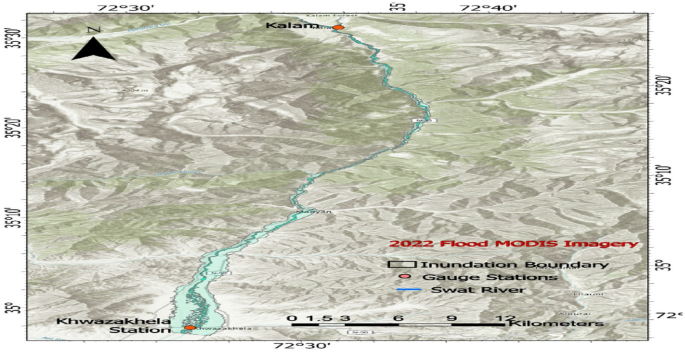
Extent of inundation observed in MODIS Imagery during the 2022 Flood event (Kalam to Khwazakhela)
3.2.2 Floodplain and hazard maps
The HEC-RAS 2D flood model's output includes data on flood inundation depth, flood velocity, and the size of inundated areas. The ArcGIS Pro-created processed maps shown in the Figs. 5 , 6 , 7 , 8 , 9 , 10 and 11 the simulated inundation depths and extents for design events with return periods of 2, 5, 10, 25, 50, and 100 years in the research area.
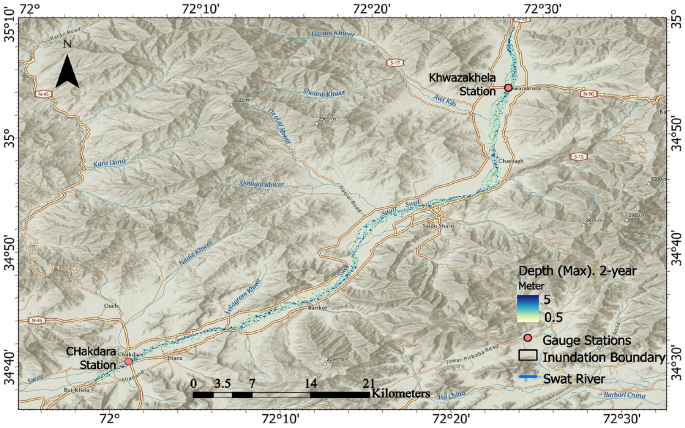
Modeled inundation extent and depth for 2-year return periods. (Chakdara to Khwazakhela)

Modelled inundation extent and depth for 5-year return periods. (Chakdara to Khwazakhela)
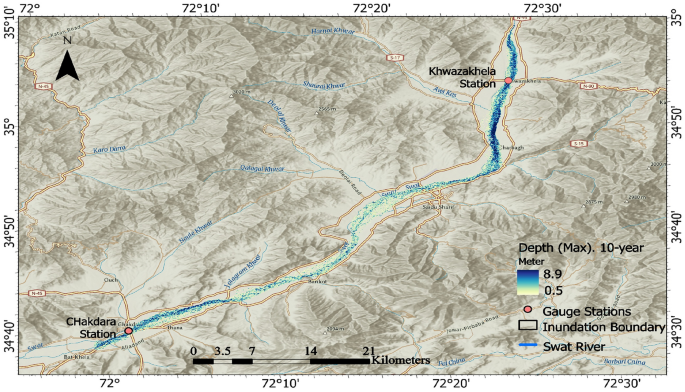
Modelled inundation extent and depth for 10-year return periods. (Chakdara to Khwazakhela)
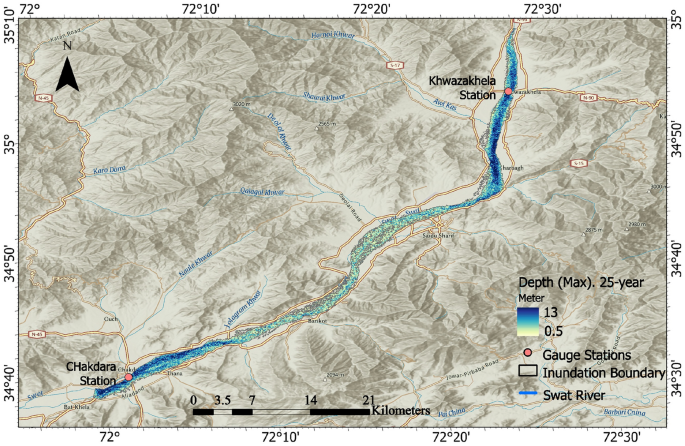
Modelled inundation extent and depth for 25-year return periods. (Chakdara to Khwazakhela)
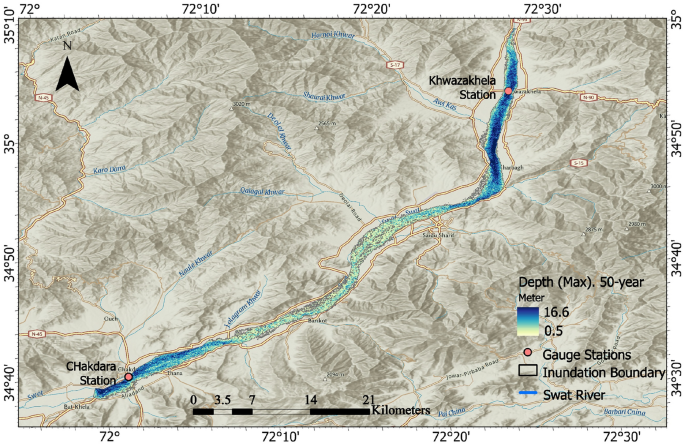
Modelled inundation extent and depth for 50-year return periods. (Chakdara to Khwazakhela)
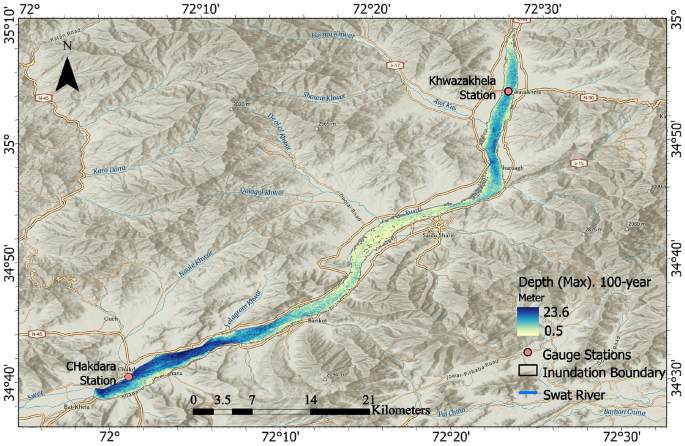
Modelled inundation extent and depth for 100-year return periods. (Chakdara to Khwazakhela)
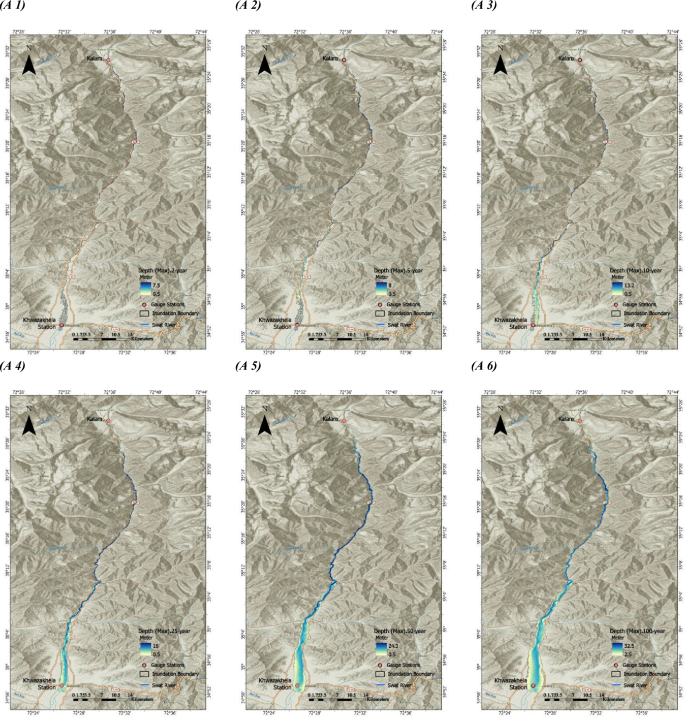
Modelled inundation extent and depth (Kalam to Khwazakhela), A1) 2-year return periods. A2) 5-year return periods. A3) 10-year return periods. A4) 25-year return periods. A5) 50-year return periods A6) 100-year return periods
The Fig. 12 shows the flood hazard layer for the 100-year return period was reclassified in accordance with the instructions supplied by the National Disaster Management Authority (NDMA) of Pakistan given in Table 5 , which led to the identification of three different hazard zones. As part of the reclassification process, places were divided into groups according to how susceptible they were to flood. The information on these hazard zones is provided in the Table 5 that follows and it provides helpful details on the various flood risk levels in the research area. Implementing this classification enables stakeholders and decision-makers to allocate resources and priorities mitigation measures to locations with greater flood danger levels improving overall disaster management and preparedness efforts [ 35 , 36 , 37 ].
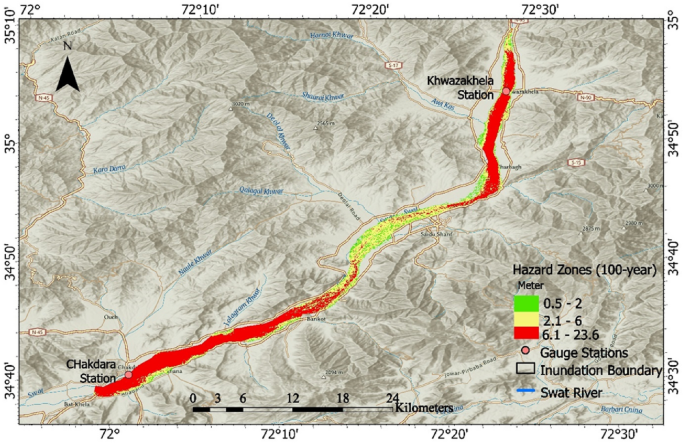
Flood Hazard Zones for 100-years return period (Khwazakhela to Chakdara reach)
A computed velocity pattern during a flood event with a 100-year recurrence interval is shown in Figs. 13 and 14 . A specific stretch of the river in the study region encountered speeds below 4 m/s, while other sections of the river achieved greater velocities of 10-15 m/s according to the modelling of Khwazakhela to Chakdara Reach velocity.
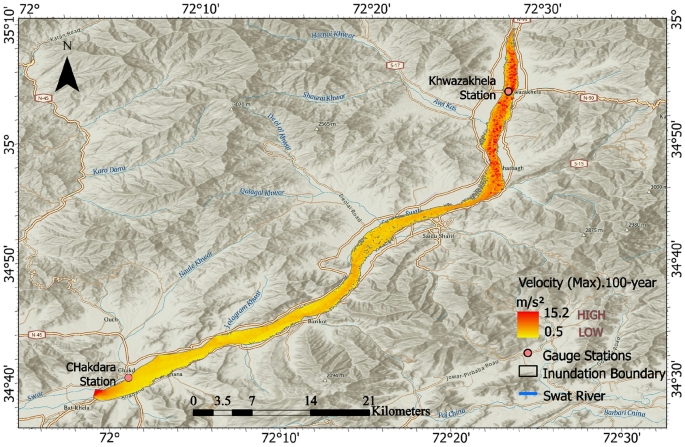
Simulated food velocity for 100-years flood return period (Khwazakhela to Chakdara Reach)
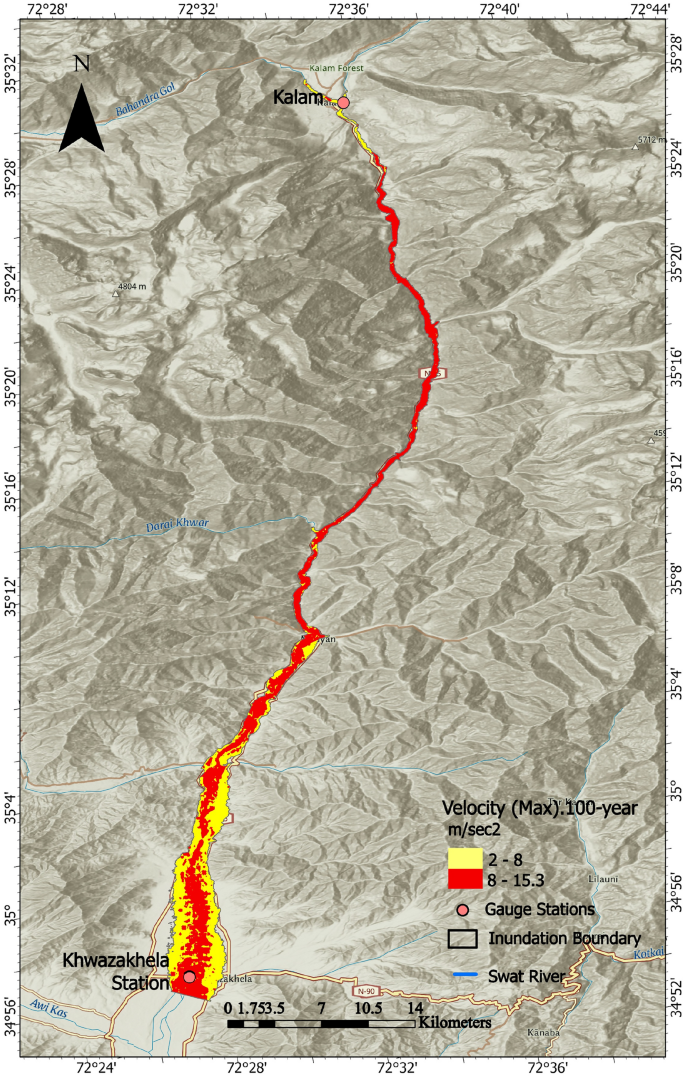
Simulated food velocity for 100-years flood return period (Khwazakhela to Kalam Reach)
The Khwazakhela to Kalam stretch includes a very hilly area where flood occurrences can cause water flow rates of up to 10–17 m/s. The water travels through this location with great power and speed because of the uneven terrain. The risk for severe and quick flooding in these hilly locations highlighted and areas susceptible to high-velocity floods can be identified. The velocity flood maps can be helpful an inclusive flood control strategy. Stakeholders can use different methods to control and lessen the impacts of flooding by using these sources of information to get a better understanding of the flood risk.
Figures 15 and 16 shows the extent of flooding for different return periods 2, 5, 10, 25, 50, and 100 years. These analyses were conducted within ArcGIS Pro, utilizing landcover data sourced from the USGS website. The inundation area increases with increasing return periods. This relationship is linear and it is primarily attributed to the rise in flood discharge.

Khwazakhela to Chakdara reach Inundation Area (km 2 ) vs Return periods
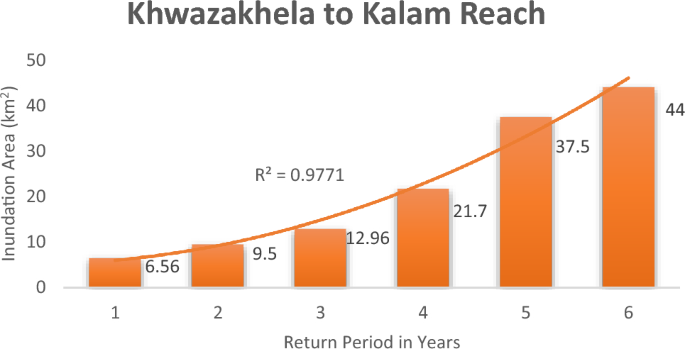
Khwazakhela to Kalam reach Inundation Area (km 2 ) vs Return periods
Using ArcGIS Pro, we conducted an analysis of landcover damages for various return periods, including 2, 5, 10, 25, 50, 100 years, and for the flood event in 2022. Figures 17 and 18 shows our findings, which indicate that croplands suffered the most significant impact, followed by prairies and orchards.

Khwazakhela to Chakdara Reach Damaged Area of various Return Periods
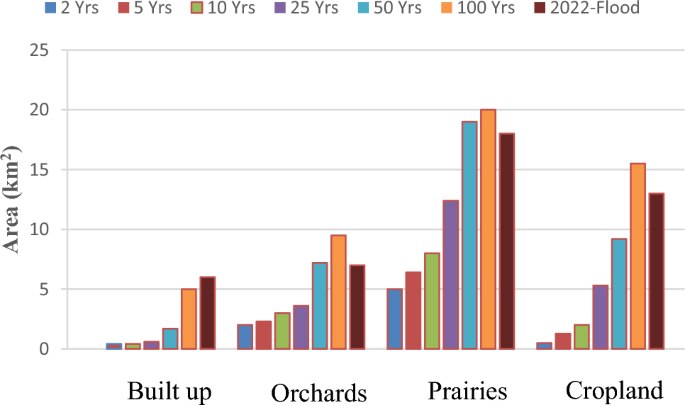
Khwazakhela to Kalam Reach Damaged Area of various Return Periods
4 Conclusion
The research was conducted along a 132-km stretch of the Swat River from Kalam to Khwazakhela Gauge station and Khwazakhela to Chakdara Gauge station. The study focused on modeling the behavior of the River Swat particularly during the Flood2022 event utilizing advanced versions of ArcGIS-Pro and HEC-RAS 2D for flood modeling. Calibration and validation of the flood model were performed with a specific focus on analyzing simulated flood depth, extent, and velocity based on 12.5-meter DEM. Subsequent simulations were conducted for various return periods namely 2, 5, 10, 25, 50, and 100 years, revealing a linear increase in flood inundation area with longer return periods. The study identified croplands and prairies as the primary land cover classes at risk and their exposure proportionally increases with increases in return periods. The flood model and associated hazard maps offer valuable insights for disaster managers to effectively address and mitigate flood vulnerability within the studied region. For future studies it is recommended to utilize higher resolution DEM data with greater than 12.5 meters to get finer and more accurate results.
Data availability
All relevant data are included in the paper.
Yusuf A et al (2023) Modelling flood hazards impacted by ungauged river in urbanised area using HEC-RAS and GIS. Niger J Technol Dev 20(2):83–92. https://doi.org/10.4314/njtd.v20i2.1405
Article Google Scholar
Khan B, Khan A (2021) Floodplain hazard mapping and assessment of river Kabul using HEC-RAS 2D model
Statistica. Economic damage caused by significant foods worldwide up to 2016. 2018 17/08/2023]; Statistic. [online] Statista. https://www.statista.com/statistics/267750/economic-damage-caused-by-food
Khouz A et al (2023) Flood susceptibility assessment through statistical models and HEC-RAS analysis for sustainable management in Essaouira Province, Morocco. Geosciences 13(12):382
Mejia Manrique SA et al (2021) Flood impacts on critical infrastructure in a coastal floodplain in Western Puerto Rico during Hurricane María. Hydrology 8(3):104. https://doi.org/10.3390/hydrology8030104
Nagarajan K et al (2022) Review paper for floodplain mapping with applications of HEC-HMS, HEC-RAS, and ArcGIS softwares—a remote sensing and GIS approach. Int Res J Eng Technol (IRJET) 9(6):812–826
Google Scholar
Ikirri M et al (2022) Flood hazard index application in arid catchments: case of the taguenit wadi watershed, Lakhssas, Morocco. Land 11(8):1178. https://doi.org/10.3390/land11081178
Pradhan D, Sahu RT, Verma MK (2022) Flood inundation mapping using GIS and Hydraulic model (HEC-RAS): a case study of the Burhi Gandak river, Bihar, India, in soft computing: theories and applications: proceedings of SoCTA 2021. Springer, pp 135–145. https://doi.org/10.1007/978-981-19-0707-4_14
Beza M, Fikre A, Moshe A (2023) Dam breach modeling and downstream flood inundation mapping using HEC-RAS model on the proposed Gumara Dam, Ethiopia. Adv Civ Eng 11:78. https://doi.org/10.1155/2023/8864328
Gashu MW et al (2023) Assessment of River Bank overtopping the problem using HEC-RAS (in the case of Sedie River, Abbay Basin, Ethiopia). Water Conserv Sci Eng 8(1):28. https://doi.org/10.1007/s41101-023-00197-w
Hec-Ras. The official public website of the U.S. Army Corps of Engineers Hydrologic Engineering Center (HEC). 2023. https://www.hec.usace.army.mil/software/hec-ras/download.aspx
Akram S (2023) Flood hazard modelling using hydraulic simulation model and satellite images: a case study of Chaj Doab, Punjab, Pakistan. Pak J Sci 75(04):634–644. https://doi.org/10.57041/pjs.v75i04.1027
Kiba LG, Nengzouzam G, Ranjan P (2023) Flood hazard mapping using hydraulic models and GIS: a review. River sediment and hydrological extremes: causes, impacts and management. pp 65–72. https://doi.org/10.1007/978-981-99-4811-6_4
Pro-3.1 A (2023) ArcGIS Pro is a full-featured professional desktop GIS application from Esri. https://pro.arcgis.com/en/pro-app/latest/get-started/get-started.htm .
Khattak MS et al (2015) Floodplain mapping using HEC-RAS and ArcGIS: a case study of Kabul River. Arab J Sci Eng 41(4):1375–1390. https://doi.org/10.1007/s13369-015-1915-3
Article MathSciNet Google Scholar
Bahadar I et al (2015) Flood hazard assessment using hydro-dynamic model and GIS/RS tools: a case study of Babuzai-Kabal tehsil Swat Basin, Pakistan. J Himal Earth Sci 48(2):129
Callow JN, Van Niel KP, Boggs GS (2007) How does modifying a DEM to reflect known hydrology affect subsequent terrain analysis. J Hydrol 332(1):30–39. https://doi.org/10.1016/j.jhydrol.2006.06.020
Djedaiet K, Ghachi A (2023) Simulation of flood’s risk using GIS and Hec-Ras in the lower valley of Wadi El Kebir-Tebessa, Algeria. Ann Univ Bucharest Geogr Ser/Analale Universitatii Bucuresti. Seria Geografie 11:78. https://doi.org/10.5719/aub-g/72.1/2
Zahmatkesh Z, Han S, Coulibaly P (2021) Understanding uncertainty in probabilistic floodplain mapping in the time of climate change. Water 13(9):1248. https://doi.org/10.3390/w13091248
Skakun S et al (2013) Flood hazard and flood risk assessment using a time series of satellite images: a case study in Namibia. Risk Anal Off Publ Soc Risk Anal 34(8):1521–1537. https://doi.org/10.1111/risa.12156
Bessar M et al (2021) Comparative analysis of local and large-scale approaches to floodplain mapping: a case study of the Chaudière River. Can Water Resour J/Revue canadienne des ressources hydriques 46(4):194–206. https://doi.org/10.1080/07011784.2021.1961610
Masood M, Takeuchi K (2012) Assessment of flood hazard, vulnerability and risk of mid-eastern Dhaka using DEM and 1D hydrodynamic model. Nat Hazards 61(2):757–770. https://doi.org/10.1007/s11069-011-0060-x
Solaimani K (2011) Flood forecasting based on GIS and hydraulic model. Int J Fluid Mech Res 38(3):215–224. https://doi.org/10.1615/interjfluidmechres.v38.i3.20
Li Y, Wright DB, Liu Y (2022) Flood-induced geomorphic change of floodplain extent and depth: a case study of hurricane maria in Puerto Rico. J Hydrol Eng 27(10):04022019. https://doi.org/10.1061/(ASCE)HE.1943-5584.0002199
Apel H et al (2006) A probabilistic modelling system for assessing flood risks. Nat Hazards 38:79–100. https://doi.org/10.1007/s11069-005-8603-7
Khan LT, Tasnim K (2023) Flood inundation mapping of Buriganga river floodplain using HEC-RAS 1D/2D coupled model. Eng Res Transcr 4:1–14. https://doi.org/10.55084/grinrey/ERT/978-81-964105-1-3_1
Zia SB, Womera SA, Rahman MA (2023) Hydrological assessment and flood inundation mapping for flood plain of Padma river using HEC-RAS 2D. In: AIP conference proceedings. 2023. AIP Publishing. https://doi.org/10.1063/5.0129938
Collins EL et al (2022) Predicting flood damage probability across the conterminous United States. Environ Res Lett 17(3):034006. https://doi.org/10.1088/1748-9326/ac4f0f
Hussain Z (2011) Application of the regional flood frequency analysis to the upper and lower basins of the Indus River, Pakistan. Water Resour Manag 25:2797–2822. https://doi.org/10.1007/s11269-011-9839-5
Afreen S, Muhammad F (2012) Flood frequency analysis of various dams and barrages in Pakistan. Irrig Drain 61(1):116–128. https://doi.org/10.1002/ird.621
Pandit B, Bhattarai P (2023) Flood risk mapping of Kamla River Basin using HEC-RAS 2D model. Adv Eng Technol Int J 3(1):31–45. https://doi.org/10.3126/aet.v3i1.60620
El-Bagoury H, Gad A (2024) Integrated hydrological modeling for watershed analysis, flood prediction, and mitigation using meteorological and morphometric data, SCS-CN, HEC-HMS/RAS, and QGIS. Water 16(2):356. https://doi.org/10.3390/w16020356
Islam MM, Rahman MA (2023) Development of flood inundation map for 100 years return period using HEC-RAS 2D. In: AIP conference proceedings. 2023. AIP Publishing. https://doi.org/10.1063/5.0129912
Yazdan MMS et al (2022) Estimating flooding at river spree floodplain using HEC-RAS simulation. Journal 5(4):410–426. https://doi.org/10.3390/j5040028
Efendi R et al. (2024) Integration GIS and HEC-RAS to simulate flood damage from river overflow (Study: Bengawan solo river section in Kadokan village). In: AIP conference proceedings. 2024. AIP Publishing. https://doi.org/10.1063/5.0185058
Hino M et al (2023) Growing safely or building risk? Floodplain management in North Carolina. J Am Plann Assoc. https://doi.org/10.1080/01944363.2022.2141821
Tamang S, Bhattarai P (2023) Flood hazard mapping of West Rapti and assessing impact on agricultural production in Dang District using HEC-RAS 2D
Download references
No external funding.
Author information
Authors and affiliations.
Department of Civil Engineering, University of Engineering and Technology Peshawar (Bannu Campus), Peshawar, Khyber Pakhtunkhwa, Pakistan
Muhammad Ihsan Ullah, Komal Sahab Qureshi & Ateeq ur Rauf
Department of Architecture, Building and Civil Engineering, Loughborough University, Loughborough, LE11 3TU, UK
Liaqat Ali Shah
You can also search for this author in PubMed Google Scholar
Contributions
Conceptualization: Muhammad Ihsan Ullah, Komal Sahab Qureshi., and Ateeq ur Rauf; Methodology and software: Muhammad Ihsan Ullah and Komal Sahab Qureshi.; formal analysis, data curation, writing—original draft preparation: Muhammad Ihsan Ullah.; writing—review and editing, Liaqat Ali Shah; supervision: Ateeq ur Rauf.
Corresponding author
Correspondence to Muhammad Ihsan Ullah .
Ethics declarations
Conflict of interest.
The authors affirm that there are no conflicts of interest regarding the publication of this manuscript. Furthermore, the ethical issues, data fabrication, double publication or submission to any other journal have been absolutely put under consideration by the authors.
Ethical approval
The submitted work is original and has not been published elsewhere in any form or language.
Consent to participate
All the authors have read and agreed with the participation of this manuscript.
Consent to publish
All the authors agree with the publication of this article.
Additional information
Publisher's note.
Springer Nature remains neutral with regard to jurisdictional claims in published maps and institutional affiliations.
Rights and permissions
Open Access This article is licensed under a Creative Commons Attribution 4.0 International License, which permits use, sharing, adaptation, distribution and reproduction in any medium or format, as long as you give appropriate credit to the original author(s) and the source, provide a link to the Creative Commons licence, and indicate if changes were made. The images or other third party material in this article are included in the article's Creative Commons licence, unless indicated otherwise in a credit line to the material. If material is not included in the article's Creative Commons licence and your intended use is not permitted by statutory regulation or exceeds the permitted use, you will need to obtain permission directly from the copyright holder. To view a copy of this licence, visit http://creativecommons.org/licenses/by/4.0/ .
Reprints and permissions
About this article
Ullah, M.I., Qureshi, K.S., Rauf, A.u. et al. Advanced floodplain mapping: HEC-RAS and ArcGIS pro application on Swat River. J. Umm Al-Qura Univ. Eng.Archit. (2024). https://doi.org/10.1007/s43995-024-00054-4
Download citation
Received : 16 November 2023
Accepted : 09 March 2024
Published : 03 April 2024
DOI : https://doi.org/10.1007/s43995-024-00054-4
Share this article
Anyone you share the following link with will be able to read this content:
Sorry, a shareable link is not currently available for this article.
Provided by the Springer Nature SharedIt content-sharing initiative
- Flood risk management
- Hydrological modeling
- Find a journal
- Publish with us
- Track your research

IMAGES
VIDEO
COMMENTS
HEC-RAS One-Dimensional Hydrodynamic Modelling for Recent Major Flood Events in Pahang River ... Ph.D. thesis, Universiti Sains Malaysia. 18. Jafri AM, Hashim Z, Kavvas ML, Chen ZQ, Ohara N (2013 ...
First, the Soil and Water Assessment Tool (SWAT) was used to ii. evaluate the hydrologic responses of the upper Cache la Poudre watershed to the wildfire events. Subsequently, the results from the SWAT model were used as inputs for the hydraulic model Hydrologic Engineering Center River Analysis System (HEC-RAS) to simulate channel hydraulics ...
This proves the applicability of the HEC-RAS 1D-2D coupling method as a powerful tool in simulating accurate inundations for flood events. ... Rubio, F. Flood Risk Assessment in the Vicinity of Kartena Town Using HEC-RAS 1D-2D Models. Master's Thesis, Aleksandras Stulginskis University, Kaunas, Lithuania, 2018.
Hydraulic Modeling of a River Network for Predicting Flood Inundation using HEC-RAS and GIS Models - A Case Study in Southern Virginia . Castro Bolinaga, Celso Francisco (Virginia Tech, 2012-12-17) A flood inundation study is presented for a watershed located in south central Virginia. A HEC-RAS hydrodynamic model of the main river network ...
HEC-RAS (1D) versus HEC-RAS (2D) in hydraulic modeling. ... Detailed work of this paper is part of the M.Sc. thesis of Amir Mohammad Arash (Student), under the supervision of Mehdi Yasi (Associate supervisor) and Asghar Azizian (Co-supervisor) conducted at the Department of Irrigation and Reclamation Engineering, University of Tehran, Karaj ...
3.2 One Dimensional Flood Modeling Using HEC-RAS (Hydrological Engineering Center-River Analysis System. HEC-RAS is software that describes water hydraulics flowing through common rivers and other channels. It is a computer-based modeling program for water moving through open channel systems and calculating profiles of water surfaces.
A 2D HEC-RAS model was created to demonstrate applications of hydraulic modeling for informing ecological design. This was done by comparing the hydrologic conditions of a baseline year (2010) to that of the environmental flow prescription from Kozak et al. (2016). The inundation results are relevant to hydraulic connectivity and cypress regeneration. The hydraulic model was created by ...
HEC-RAS 2D HYDRODYNAMIC MODEL AND GEOSPATIAL TECHNOLOGIES - A CASE STUDY OF RIVER SWAT, PAKISTAN . BY MUHAMMAD FAROOQ Thesis submitted to the National Centre of Excellence in Geology, University of Peshawar in partial fulfillment of requirements for the Degree of Philosophy in Geospatial Sciences NATIONAL CENTRE OF EXCELLENCE IN GEOLOGY
A thesis submitted to School of Graduate Studies of Bahir Dar Institute of Technology, Bahir Dar University In Partial Fulfillment of the Requirements for the Degree of Master of Science in Hydraulics in Faculty of Civil and ... HEC-RAS Hydrologic engineering center for river analysis system HEC-FDA Hydrologic engineering center for flood ...
Abstract: The research presented herein develops and compares an ADCIRC and ADCIRC/HEC-RAS (1D) paired model for the purpose of compound flood modeling within the Tar River and Pamlico Sound basins of North Carolina. Both the ADCIRC and 1D HEC-RAS models are capable of simulating river systems but differ in their underlying numerical formulations.
called River Analysis System, and more commonly referred to as HEC-RAS or RAS. HEC-RAS is the best and most recent method for hydraulic design of bridges, and available documentation provides guidance for its use. The author is experienced in hydraulic design of bridges and has developed this thesis in order to address certain generalities and ...
The use of hydraulic models for carrying out flood simulations is a common practice globally. The current study used HEC-RAS (2D) in order to simulate different flood scenarios on the River Yesil (Ishim). Comparison of different mesh sizes (25, 50 and 75 m) indicated no significant difference in model performance. However, a significant difference was observed in simulation time. In addition ...
Floods frequently threaten villages near the Khazir River's floodplains, causing crop losses and threatening residential areas. We used flood-related hydrological software, including WMS and HEC-HMS, to study this issue and determine how to reduce the recurrence of flooding. The software can be used to calculate a hydrograph of torrential flows in a river drainage basin and estimate the ...
A Master of Science thesis in Civil Engineering by Heba Hussien entitled, "Water Quality Modeling of Dubai Creek using HEC-RAS," submitted in May 2015. Thesis advisor is Dr. Maruf Mortula and thesis co-advisor is Dr. Serter Atabay. Soft and hard copy available.
The HEC-RAS model outcome indicated that the flood inundation mapping area for 2, 5, 10, 25, 50, and 100-year recurrence intervals, respectively, was 71.475, 76.630, 89.150, 100.290, 105.160, and 109.462 km2. Finally, it was realized that the whole Awash Bello flood plain is under the influence of flood inundation due to the intensive rainfall ...
The object of this thesis is the evaluation of the restoration potential of small hydraulic structures for increasing water retention in river floodplain by using 2D HEC-RAS hydraulic model. A former millrace of the Rožnovská Bečva River was used for the design of restoration measures.
Quirogaa et al. applied HEC-RAS 2D to simulate the flood events of February 2014 for a case study of Llanos de Moxos, Bolivian Amazonia. They verified the same with available satellite images. Bhandari et al. used HEC-RAS 2D in a lower region of the Brazo River watershed, Richmond, TX. Maximum water depth and flood velocities were 13.1 m and 16 ...
Flood Modeling and Hazard Mapping using HEC-RAS 2D Hydrodynamic Model and Geospatial Technologies- A Case Study of River Swat, Pakistan @inproceedings{Farooq2019FloodMA, title={Flood Modeling and Hazard Mapping using HEC-RAS 2D Hydrodynamic Model and Geospatial Technologies- A Case Study of River Swat, Pakistan}, author={Muhammad Farooq}, year ...
HEC-RAS is able to model temperature, nitrogen cycle (nitrate, nitrite, ammonium, and organic nitrogen), phosphorus cycle (organic and dissolved), algae, DO, and biological oxygen demand in one dimension. ... M.Sc. thesis, Master of Science in Civil Engineering, The Ohio state university, Ohio. Palmieri V, de Carvalho RJ (2006) Qual2e model for ...
The obtained cross sections were defined in the HEC-RAS software, and the hydraulic characteristics of the flood bed and the water surface profiles of the Q 25 , Q 50 , Q 100 , and Q 500 flood recurring and one-dimensional floodplain analysis of the Tigris River were determined. ... 2005, Ph.D. thesis. View at: Google Scholar. C. Erkek and N ...
In Step 3 the DEM and peak discharge data for various return periods were loaded into the HEC-RAS. Step 4 involved a thorough examination of the HEC-RAS model in order to produce an advanced 2D model that improved our understanding of flood dynamics [26, 27]. The results of the analysis were then imported into ArcGIS Pro (Step 4) then the model ...
HEC-RAS User's Manual. Welcome to the River Analysis System. Get Started. Topics. Foreword Introduction to HEC-RAS Installing HEC-RAS Working With HEC-RAS Entering and Editing Geometric Data Developing the River System Schematic ...