- Contact Contact icon Contact
- Training Training icon Training

- Portuguese, Brazil
- Chinese, Simplified
Check the spelling in your query or search for a new term.
Site search accepts advanced operators to help refine your query. Learn more.

CAS Content
The world's largest collection of chemistry insights.
Every day CAS scientists collect and analyze published scientific literature from around the globe, building the highest quality and most up-to-date collection of scientific information in the world.
Covering advances in chemistry and related sciences over the last 150 years, the CAS Content Collection empowers researchers, business leaders, and information professionals around the world with immediate access to the reliable information they need to fuel innovation.
Human intelligence is at the heart of the CAS Content Collection. Our team of scientific analysts embodies a wealth of specialized knowledge that lets them accurately extract and verify a multitude of data and key insights from each publication, making connections and uncovering trends only possible with the combined power of expert human analysis and advanced data technology.
CAS COVID-19 Resources
Aligned with our mission as a division of the American Chemical Society, CAS is making a wide range of resources and expertise openly available to support research related to COVID-19. ( see other open access content)
As the authoritative source for chemical names, structures, and CAS Registry Numbers ® , the CAS substance collection, CAS REGISTRY ® , serves as a universal standard for chemists worldwide.
- 204 million organic and inorganic substances disclosed in literature since the early 1800s
- Includes alloys, coordination compounds, minerals, mixtures, polymers, and salts
- 69 million protein and nucleic acid sequences
- Enriched with experimental and predicted property data and spectra
- Updated daily
CAS References
Details from thousands of global scientific references are added to the CAS Content Collection every day creating a comprehensive resource to access and keep up to date on the world’s published scientific literature across disciplines including biomedical sciences, chemistry, engineering, materials science, agricultural science, and many more.
- Sourced from thousands of journals and 64 patent authorities as well as technical reports, books, conference proceedings, and dissertations published globally
- More than 59 million records from publications dating back to the early 1800s, with continuous coverage since 1907
- English language summaries translated from publications in more than 50 different languages from more than 180 different countries
- Indexed with standardized CAS Lexicon terminology for enhanced retrieval and search precision
- Enhanced with cited and citing references, legal status, and direct links to the full-text publications (where available)
CAS Reactions
The CAS reaction collection provides a current and reliable source of synthetic details sourced from journals, patents, dissertations, and seminal reference works to drive synthesis planning and process optimization.
- Covers ~150 million reactions from 1840 to present including organometallics, total syntheses of natural products, and biotransformation reactions.
- Enhanced with yield data, detailed reaction conditions, and experimental procedures
CAS Chemical Supplier Insights
Keep your research moving forward with CAS Chemical Supplier Insights , CAS's up-to-date resource for finding commercially available chemicals, including available quantities, prices, and supplier contact information.
- Includes millions of unique commercially available products
- Covers verified catalogs from hundreds of suppliers
- Updated weekly
Chemical Regulations
Stay abreast of rapidly changing chemical regulations with CAS regulatory resources . Our collection provides the information you need to manufacture, import, export, transport, and use chemical products in key markets across the globe.
- Covers more than ~417,000 unique chemical substances
- Includes information from 150 regulatory inventories and lists
CAS Markush Representations in Patents
Crucial chemistry disclosures in patents are often buried in complex CAS Markush representations that can embody hundreds of specific substances. Common search engines cannot reliably interpret this information making it difficult to achieve a comprehensive patent search, but the scientists at CAS analyze these Markush representations and make them searchable to ensure that your queries don’t miss critical documents.
- More than 1.39 million searchable Markush representations from >570,000 patents
- Continuous coverage since 1988, with selected coverage back to 1961
Open Access Content
Covid-19 resources.
As a specialist in scientific information solutions, CAS is partnering with research organizations around the globe to tackle the rapidly evolving and complex challenge of COVID-19. Aligned with our mission as a division of the American Chemical Society, CAS is making a wide range of resources and expertise openly available to support this fight. If customized support from CAS scientists and technologists would be valuable to augment your COVID-19 research efforts, we welcome your outreach to the CAS Customer Center .
Chemical Safety Library
The Pistoia Alliance Chemical Safety Library (CSL) is a new open-access platform that streamlines access to hazardous reaction information crowd-sourced from academic, industry and government institutions around the world. Developed and hosted by CAS, the CSL supports laboratory safety by providing tools to:
- Search for hazardous reaction information by CAS Registry Number ® , chemical name, SMILES and more.
- Contribute new hazardous reaction incident information to the library.
- Download aggregated information to integrate with internal workflows and knowledge bases.
The CAS Source Index (CASSI) Search Tool is an online resource intended to support researchers and librarians who need accurate bibliographic information. This complimentary resource can be used to quickly and easily look up or confirm publication titles and abbreviations, as well as CODEN, ISBN, or ISSN codes. The CASSI database contains a listing of publications indexed by Chemical Abstracts Service (CAS) since 1907.
Common Chemistry
This publicly available resource provides authoritative information on nearly 500,000 compounds from CAS REGISTRY ® . The collection represents substances commonly found on regulatory lists, in consumer products and as part of introductory chemical curricula. Search functionality and an application programming interface (API) enable interested individuals to quickly find chemical names, CAS Registry Numbers ® , structures or basic compound properties to foster learning and promote safety.
Links to Wikipedia records (when available) have been provided by the Wikipedia Chemicals WikiProject in collaboration with CAS.
- AI Generator
chain reaction
215 chemical reaction abstract stock photos & high-res pictures, browse 215 authentic chemical reaction abstract stock photos, high-res images, and pictures, or explore additional chain reaction or innovation stock images to find the right photo at the right size and resolution for your project..
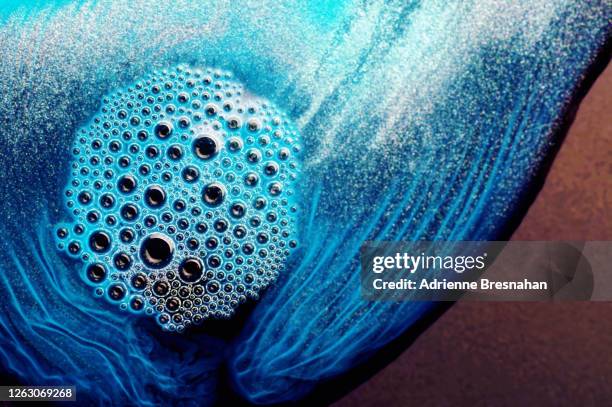
If you're seeing this message, it means we're having trouble loading external resources on our website.
If you're behind a web filter, please make sure that the domains *.kastatic.org and *.kasandbox.org are unblocked.
To log in and use all the features of Khan Academy, please enable JavaScript in your browser.
Unit 3: Chemical reactions
About this unit.
Unlock the world of chemical reactions! Get ready to decode the language of chemistry, using symbols and equations to gain a deeper understanding of how substances interact and change at the atomic level. We'll also explore the underlying energy shifts that drive these reactions, laying a solid foundation for more advanced chemical concepts.
Representing chemical reactions
- Physical and chemical changes (Opens a modal)
- Intro to chemical reactions (Opens a modal)
- Symbols in chemical equations (Opens a modal)
- Apply: representing chemical reactions Get 3 of 4 questions to level up!
- Apply: counting atoms in chemical equations Get 3 of 4 questions to level up!
Balancing chemical equations
- Balancing chemical equations (Opens a modal)
- Balancing more complex chemical equations (Opens a modal)
- Visually understanding balancing chemical equations (Opens a modal)
- Balancing another combustion reaction (Opens a modal)
- Apply: balancing equations Get 3 of 4 questions to level up!
Activity: How can a big log turn into a tiny pile of ash when it burns?
- Activity: How can a big log turn into a tiny pile of ash when it burns? (Opens a modal)
Energy of chemical reactions
- Endothermic and exothermic reactions (Opens a modal)
- Understand: energy of chemical reactions Get 3 of 4 questions to level up!
- Apply: energy of chemical reactions Get 3 of 4 questions to level up!
Chemical Reactions: Types of reactions and the laws that govern them
by Anthony Carpi, Ph.D., Adrian Dingle, B.Sc.
Did you know that chemical reactions happen all around us, such as when you light a match, start a car, or even take in a breath of air? But no matter the type of reaction, in every case a new substance is produced and is often accompanied by an energy and/or an observable change.
- The steps from a qualitative science to quantitative one, were crucial in understanding chemistry and chemical reactions more completely.
- When a substance or substances (the reactants), undergo a change that results in the formation of a new substance or substances (the products), then a chemical reaction is said to have taken place.
- Mass and energy are conserved in chemical reactions. Matter is neither created or destroyed, rather it is conserved but rearranged to create new substances. No energy is created or destroyed, it is conserved but often converted to a different form.
- Chemical reactions can be classified into different types depending on their nature. Each type has its own defining characteristics in terms of reactants and products.
- Chemical reactions are often accompanied by observable changes such as energy changes, color changes, the release of gas or the formation of a solid.
- Energy plays a crucial role in chemical reactions. When energy is released into the surroundings the reaction is said to be exothermic; when energy is absorbed from the surroundings the reaction is said to be endothermic
This is an updated version of the module Chemical Reactions (previous version) .
Chemical reactions happen absolutely everywhere. While we sometimes associate chemical reactions with the sterile environment of the test tube and the laboratory - nothing could be further from the truth. In fact, the colossal number of transformations make for a dizzying, almost incomprehensible array of new substances and energy changes that take place in our world every second of every day.
In nature, chemical reactions can be much less controlled than you’ll find in the lab, sometimes far messier, and they generally occur whether you want them to or not! Whether it be a fire raging across a forest (Figure 1), the slow process of iron rusting in the presence of oxygen and water over a period of years, or the delicate way in which fruit ripens on a tree, the process of converting one set of chemical substances (the reactants) to another set of substances (the products) is one known as a chemical reaction .
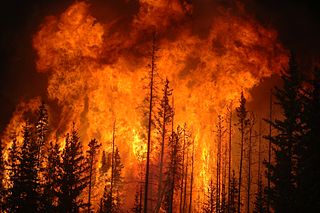
Figure 1 : A controlled fire in Alberta, Canada, set to create a barrier for future wildfires.
Though chemical reactions have been occurring on Earth since the beginning of time, it wasn’t until the 18th century that the early chemists started to understand them. Processes like fermentation, in which sugars are chemically converted into alcohol , have been known for centuries; however, the chemical basis of the reaction was not understood. What were these transformations and how were they controlled? These questions could only be answered when the transition from alchemy to chemistry as a quantitative and experimental science took place.
- Historical context
Beginning in the early Middle Ages , European and Persian philosophers became fascinated with the way that some substances seemed to “transmute” (or transform) into others. Simple stones, such as those that contained sulfur, seemed to magically burn; and otherwise unimpressive minerals were transformed, like the ore cinnabar becoming an enchanting silvery liquid metal mercury when heated. Alchemists based their approach on Aristotle’s ideas that everything in the world was composed of four fundamental substances - air, earth, fire, and water (Figure 2).
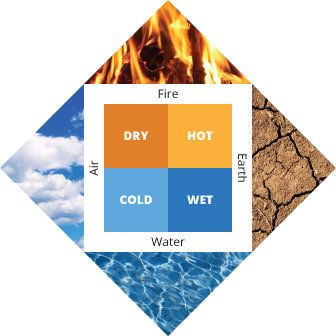
Figure 2 : Aristotle believed that everything in the world was composed of four fundamental substances - air, earth, fire, and water.
As such, they proposed, and spent generations trying to prove, that less expensive metals like copper and mercury could be turned into gold. Despite their misguided approach, many early alchemists performed foundational chemical experiments - transforming one substance into another, and so it is difficult to point to a specific date or event as the birth of the idea of an ordered, quantifiable chemical reaction . However, there are some important moments in history that have helped to make sense of it.
- Lavoisier: Law of Mass Conservation
Antoine Lavoisier was a French nobleman in the 1700s who began to experiment with different chemical reactions . At the time, chemistry still couldn’t be described as being a true, quantitative science. Most of the theories that existed to explain the way that substances changed relied upon Greek philosophy, and there was precious little experimental detail attached to the alchemist’s tinkering.
However, during the second half of the 18th century, Lavoisier performed many quantitative experiments and observed that while substances changed form during a chemical reaction , the mass of the system – or a measure of the total amount of “stuff” present – did not change. In doing so, Lavoisier championed the idea of conservation of mass during transformations (Figure 3). In other words, unlike the alchemists before him who thought that they were creating matter out of nothing, Lavoisier proposed that substances are neither created nor destroyed, but rather change form during reactions . Lavoisier’s ideas were published in the seminal work Traité élémentaire de Chimie in 1789 (Lavoisier, 1789), which is widely hailed as the birth of modern chemistry as a quantitative science.
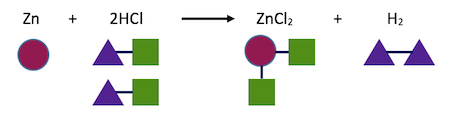
Figure 3 : Lavoisier's Law of Mass Conservation, which states that substances are neither created nor destroyed, but rather change form during reactions. In this example, the reactants (zinc and two hydrogen chloride molecules) convert into different products (zinc chloride and dihydrogen), but no mass is lost or created.
- Proust: Law of Constant Composition
Joseph Proust was a French actor who followed in Lavoisier’s footsteps. Proust performed dozens of chemical reactions , starting with different amounts of various materials. Over time he observed that no matter how he started a certain chemical reaction , the ratio in which the reactants were consumed was always constant . For example, he worked extensively with copper carbonate and no matter how he changed the ratio of starting reactants, the copper, carbon, and oxygen all reacted together in a constant ratio (Proust, 1804). As a result, in the last few years of the 18th century, Proust formulated the law of constant composition (also referred to as the law of definite proportions, Figure 4).
He realized that any given chemical substance (that we now define as a compound) always consisted of the same ratio by mass of its elemental parts regardless of the method of preparation. This was a huge step forward in modern chemistry since it had been previously believed that the substances formed during chemical reactions were random and disordered.
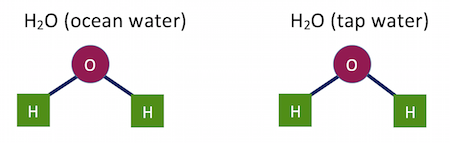
Figure 4 : An example of Proust's Law of Constant Composition, which states that any compound always consists of the same ratio by mass of its elemental parts, regardless of the method of preparation.
- Dalton: Law of Multiple Proportions
The English chemist John Dalton helped make sense of the laws of conservation of mass and definite proportions in 1803 by proposing that matter was made of atoms of unique substances that could not be created or destroyed (see our module Early Ideas about Matter for more information).
Dalton extended Proust’s ideas by recognizing that it was possible for two elements to form more than one compound , but that whatever the compound was, it would always contain elements combined in whole number ratios (Dalton, 1808). This observation is known as the law of multiple proportions (Figure 5) and with his atomic theory , helped to cement Lavoisier’s observations.
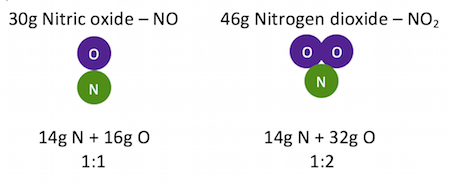
Figure 5 : Dalton's Law of Multiple Proportions, which states that two elements may form more than one compound, but whatever the compound was, it would always contain elements combined in whole number ratios
These advancements, taken together, laid the groundwork for our modern understanding of chemical reactions , chemical equations, and chemical stoichiometry , or the process of expressing the relative quantities of reactants and products in a chemical reaction .
Comprehension Checkpoint
- Types of chemical reactions
There is a staggering array of chemical reactions . Chemical reactions occur constantly within our bodies, within plants and animals, in the air that circulates around us, in the lakes and oceans that we swim in, and even in the soil where we grow crops and build our homes. In fact, there are so many chemical reactions that occur that it would be difficult, if not impossible, to understand them all. However, one method that helps us to understand them is to categorize chemical reactions into a few, general types. While not a perfect system , placing reactions together according to their similarities helps us to identify patterns, which in turn allows predictions to be made about as yet unstudied reactions. In this module, we will consider and provide some context for a few categories of reactions, specifically: synthesis , decomposition, single replacement, double replacement, REDOX (including combustion), and acid-base reactions.
No matter the type of reaction , one universal truth applies to all chemical reactions . For a process to be classified as a chemical reaction, i.e., one where a chemical change takes place, a new substance must be produced. The formation of a new substance is nearly always accompanied by an energy change, and often with some kind of physical or observable change. The physical change can be of different types, such as the formation of bubbles of a gas , a solid precipitate , or a color change. These changes are clues to the existence of a chemical reaction and are important triggers for further research by chemists.
- Synthesis reactions
Prior to Lavoisier’s work, it was poorly understood that there were different gases made up of different elements . Instead, various gases were commonly mischaracterized as types of "air" or air missing parts – for example, terms commonly used were "inflammable air," or "dephlogisticated air." Lavoisier thought differently and was convinced that these were different substances. He conducted experiments where he mixed inflammable air with dephlogisticated air and a spark, and he found that the substances combined to produce water. In response, he renamed inflammable air "hydrogen" from the Greek hydro for "water" and genes for "creator." In so doing, Lavoisier was identifying a synthesis reaction . In general, a synthesis reaction is one in which simpler substances combine to form another more complex one. Hydrogen and oxygen (which Lavoisier also renamed dephlogisticated air) combine in the presence of a spark to form water, summarized by the chemical equation shown below (for more on chemical equations see the section called Anatomy of a chemical equation ), it represents a simple synthesis reaction.
2H 2(g) + O 2(g) → 2H 2 O (l)
- Decomposition reactions
In 1774, the scientist Joseph Priestley turned his curiosity to a mineral called cinnabar – a brick red mineral. When he placed the mineral under sunlight amplified by a powerful magnifying glass, he found that a gas was produced which he described as having an “exalted nature” because a candle burned in the gas brightly (Priestley, 1775). Without realizing it, Priestley had discovered oxygen as a result of a decomposition reaction . Decomposition reactions are often thought of as the opposite of synthesis reactions since they involve a compound being broken down into simpler compounds or even elements . In the case of Priestley’s oxygen, he had broken down mercury (II) oxide (cinnabar) with heat into its individual elements. The reaction can be summarized in the following equation.
- Single replacement reactions
The British chemist and meteorologist John Daniell, invented one of the very first practical batteries in 1836 (Figure 6). In his cell, Daniell utilized a very common single replacement reaction . His early cells were complicated affairs, with ungainly parts and complicated constructs, but by contrast, the chemistry behind them was really quite simple.
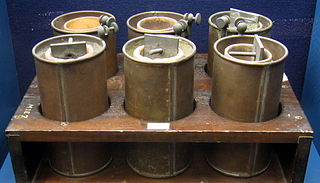
Figure 6 : Daniell cell batteries.
In certain chemical reactions , a single constituent can substitute for another one already joined in a chemical compound . The Daniell cell works because zinc can substitute for copper in a solution of copper sulfate, and in so doing exchange electrons that are used in the battery cell. The reaction can be summarized as follows:
This particular single displacement is called a metal displacement since it involves one metal replacing another metal, and many types of batteries are based on metal replacement reactions . However, several other types of single replacement reactions exist, such as when a metal can replace hydrogen from an acid or from water, or a halogen can replace another halogen in certain salt compounds .
- Combustion reactions
The controlled use of fire was a crucial development for early civilization. While it’s difficult to pin down the exact time that humans first tamed the combustion reactions that produce fire, recent research suggests it may have occurred at least a million years ago in a South African cave (Berna et al. 2012).
Chemically, combustion is no more than the reaction of a fuel (wood, oil, gasoline, etc.) with oxygen. For combustion to take place there must be a fuel and oxygen gas . However, these reactions often require activation energy (discussed in more detail in the module Chemical Bonding: The Nature of the Chemical Bond ), which can be provided by a ‘spark’ or source of energy for ignition. Fuel, oxygen, and energy are the three things make up what is known as the fire triangle (Figure 7), and any one of them being absent means that combustion will not take place.
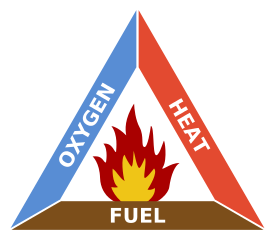
Figure 7 : The fire triangle is made up of three things - fuel, oxygen, and energy.
In the modern world, many of the fuels that are typically burned for energy , are hydrocarbons – substances that contain both hydrogen and carbon (as discussed in more detail in our Carbon Chemistry module). Plants produce hydrocarbons when they grow, and thus make an excellent fuel source, and other hydrocarbons are produced when plants or animals decay over time (such as natural gas , oil, and other substances). When these fuels combust, the hydrogen and carbon within them combine with oxygen to produce two very familiar compounds , water, and carbon dioxide. One simple example is the combustion of natural gas, or methane, CH 4 :
As with the combustion of all fuels, heat and light are products , too, and it is these products that are used to cook our food or to heat our homes.
- Reduction-oxidation reactions
Each of the four types of reaction above are sub-categories of a single type of chemical reaction known as redox reactions. A redox reaction is one where reduction and oxidation take place together, hence the name. The individual processes of oxidation and reduction can be defined in more than one way, but whatever the definition, the two processes are symbiotic, i.e., they must take place together.
In one definition, oxidation is described as the process in which a species loses electrons , and reduction is a process where a species gains electrons. In this way, we can see how the pair must take place together. If a chemical substance is to lose electrons (and therefore be oxidized), then it must have another, interdependent chemical substance that it can give those electrons to. In the process, the second substance (the one gaining electrons) is said to be reduced. Without such an electron acceptor, the original species can never lose the electrons and no oxidation can take place. When the electron acceptor is present, it gets reduced and the redox combination process is complete. Redox reactions of this type can be summarized by a pair of equations – one to show the loss of electrons (the oxidation), and the other to show the gain of electrons (the reduction). Using the example of the Daniell cell above,
The electrons shown being lost by zinc in the first reaction , are the same electrons being accepted by the copper ions in the second. Together, the reactions can be combined to cancel out the electrons on either side of the reactions, into the overall redox reaction:
Other definitions of oxidation and reduction also exist, but in every case, the two halves of the redox reaction remain symbiotic – one loses and the other gains. The loss from one species cannot happen without the other species gaining.
- Double displacement reactions
When soap won’t easily produce a lather in water, the water is said to be ‘hard’. Hard water causes all kinds of problems that go beyond just making it difficult to form a lather. The buildup of compounds in water pipes (known as ‘scale’), can block the flow of water and can cause problems in industrial processes. Textile manufacturing and the beverage industry rely heavily on water. In those situations, the quality of the water can make a difference to the end product , so controlling the water composition is crucial.
Hard water contains magnesium or calcium ions in the form of a dissolved salt such as magnesium chloride or calcium chloride. When soap (sodium stearate) comes into contact with either of those salts, it enters into a double displacement reaction that forms the insoluble precipitate known as ‘soap scum’.
A double displacement reaction (also known as a double replacement reaction) occurs when two ionic substances come together and both substances swap partners. In general:
Where A and C are cations (positively charged ions), and B and D are anions (negatively charged).
In the case of the reaction of soap with calcium chloride, the reaction is:
The solid calcium stearate is what we call soap scum, which is formed by the reaction of the soluble sodium stearate salt (the soap) in a double replacement reaction with calcium chloride.
- Acid-Base reactions
Acid-base reactions happen around, and even inside of us, all the time. From the classic elementary school baking soda volcano to the process of digestion, we encounter acids and bases on a daily basis.
When a hydrogen atom loses its only electron , it forms a positive ion , H + . This hydrogen ion is the essential component of all acids , and indeed one definition of an acid is that of a hydrogen ion donor. Compounds such as the citric acid in lemon juice, the ethanoic acid in vinegar, or a typical laboratory acid like hydrochloric acid, all give their hydrogen ions away in chemical reactions known as acid-base reactions . The chemical opposites of acids are known as bases , and bases can be defined as hydrogen ion acceptors. Whenever an acid donates a hydrogen ion to a base, an acid-base reaction has taken place, for example, when hydrochloric acid donates a hydrogen ion to a base such as sodium hydroxide:
A closer look at this reaction reveals that in water the HCl gives away an H + as shown below:
The resulting species , H 3 O + (the hydronium ion), can, in turn, act as an acid when it comes into contact with any species that can accept a hydrogen ion , such as hydroxide ions from sodium hydroxide:
Combining equations #9a and #9b gives us equation #9c.
Equation #9c can be re-written to show the individual ions that are found in solution , thus:
Removing the spectator ions from the equation above, we get the net ionic equation:
Any chemical reaction that forms water from the reaction between an acid and base as in equation #9e is known as a neutralization reaction.
- Anatomy of a chemical equation
Chemical equations are always linked to chemical reactions since they are the shorthand by which chemical reactions are described. That fact alone makes equations incredibly important, but equations also have a crucial role to play in describing the quantitative aspect of chemistry, something that we formally call stoichiometry .
All chemical reactions take on the same, basic format. The starting substances, or reactants , are listed using their chemical formula to the left-hand side of an arrow, with multiple reactants separated with plus signs. In the case of a reaction between carbon and oxygen:
To the right hand of the arrow one finds the chemical formulas of the new substance or substances (known as the products) that are produced by the chemical reaction . In this case, since carbon dioxide is the result of burning carbon in the presence of oxygen:
Since reactions can result in both physical as well as chemical changes, each substance is given a state symbol written as a subscript to the right of the formula , this describes the physical form of the reactants and products . Common state abbreviations are (s) for solids , (l) for liquids , (g) for gases and (aq) for any aqueous substances, i.e., those dissolved in water.
Finally, in order to ensure that this representation abides by the law of conservation of mass , the equation may need to be balanced by the addition of numbers in front of each species that create equal numbers of atoms of each element on each side of the equation. In the case of the formation of carbon dioxide from carbon and oxygen, there is no need for the addition of such numbers (called the stoichiometric coefficients), since 1 carbon atom and 2 oxygen atoms appear on each side of the equation.
- Energy changes
In nature, chemical reactions are often driven by exchanges in energy . In this respect, reactions are generally separated into two categories – those that release energy and those that absorb energy.
Exothermic reactions are those that release energy to the surroundings (Figure 8, right). Combustion reactions are an obvious example because the energy released by the reaction is converted into the light and heat seen in the immediate surroundings.
By contrast, endothermic reactions are those that absorb energy from the surroundings (Figure 8, left). In this situation, one may have to heat up the reaction or add some other form of energy to the system before seeing the reaction proceed.
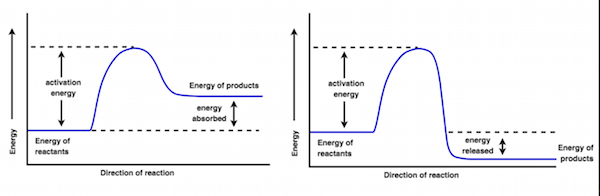
Figure 8 : On the left is an endothermic reaction, where energy is absorbed from the surroundings. In contrast, on the right is an exothermic reaction, which releases energy into the surroundings.
In both cases it is important to note that energy is neither created nor destroyed, rather it is transferred from one type of energy to another, for example from chemical energy to that of heat or light . The energy that goes into the formation of chemical bonds is exchanged for other types of energy with the environment around that reaction . A classic example is the photosynthesis reaction, in which plants absorb light energy from the sun in order to create bonds between atoms that make up sugars , which are stored as chemical energy for later use by the plant. The process of respiration is essentially the reverse of photosynthesis, where the bonds in sugar molecules are broken and the released energy is then used by the plant.
- The context of chemical reactions
Chemical reactions happen all around us every day. Whether it is a single replacement reaction in the battery of our flashlight, a synthesis reaction that occurs when iron rusts in the presence of water and oxygen, or an acid-base reaction that happens when we eat – we experience chemical reactions in almost everything we do. Understanding these reactions is not an abstract concept for a chemist in a far off laboratory, rather it is critical to understanding life and the world around us. To truly master chemical reactions, we need to understand the quantitative aspect of these reactions, something referred to as stoichiometry , and a concept we will discuss in another module.
Table of Contents
Activate glossary term highlighting to easily identify key terms within the module. Once highlighted, you can click on these terms to view their definitions.
Activate NGSS annotations to easily identify NGSS standards within the module. Once highlighted, you can click on them to view these standards.
Students & Educators —Menu
- Educational Resources
- Educators & Faculty
- Standards & Guidelines
- Periodic Table
- Adventures in Chemistry
- Landmarks Directory
- Frontiers of Knowledge
- Medical Miracles
- Industrial Advances
- Consumer Products
- Cradles of Chemistry
- Nomination Process
- Science Outreach
- Publications
- ACS Student Communities
- You are here:
- American Chemical Society
- Students & Educators
- Explore Chemistry
- Chemical Landmarks
Chemical Abstracts Service
National historic chemical landmark.
Dedicated June 14, 2007, at the Chemical Abstracts Service in Columbus, Ohio.
Commemorative Booklet (PDF)
The Chemical Abstracts Service, a division of the American Chemical Society, has provided the most comprehensive repository of research in chemistry and related sciences for over 100 years. CAS innovations have fueled chemical research through development of the CAS Registry SM and CAS databases which contain invaluable information for chemical scientists, including SciFinder ® and STN ® .
Early Chemical Abstracting in the United States
Growth of chemical abstracts, cas expansion: 1950’s and 1960’s, digital age and computer-enabled abstracting, the cas registry, online abstracting tools: stn, scifinder, and more, further reading, landmark designation and acknowledgments, cite this page.
The early history of chemical abstracting in the United States is intertwined with the Noyes family. In 1895, Arthur A. Noyes, a professor of physical chemistry at the Massachusetts Institute of Technology, began the Review of American Chemical Research (the Review ) as a supplement to MIT's Technology Quarterly . He began the Review because he believed American chemists were not receiving adequate credit in European publications for their accomplishments, and under his editorship, the Review published only abstracts of American chemical papers.
In 1897, the Review became part of the Journal of the American Chemical Society ( JACS ). Five years later, William A. Noyes, Sr. (Noyes), a distant cousin of Arthur A. Noyes, became editor of JACS and the Review . Noyes long believed that the American Chemical Society should publish a comprehensive and inclusive separate journal of abstracts. He worked diligently to persuade the Society to authorize an abstract journal, and in 1906, the ACS Council approved publication of Chemical Abstracts , with Noyes as editor. The first issue appeared in January 1907.
Chemical Abstracts was not the first publication to abstract chemical information. Scientific abstracts first appeared in primary journals, which published abstracts of work reported in other sources in addition to original research. One example was the Philosophical Transactions of the Royal Society. The first specifically chemical publication to include abstracts was Crell's Chemische Journal fÜr die Freunde der Naturlehre , which was published from 1778 to 1781. Other German publications appeared in the late 1700s as a forum for chemists to exchange information and research. French ( Annales de Chemie) and British ( Journal of the Chemical Society ) publications followed suit in the next century.
At the same time, publications specifically devoted to chemical abstracts began to appear. The most famous of these was Chemisches Zentralblatt , which debuted in 1830. It was published weekly under the editorship of Gustav Theodor Fechner. Chemisches Zentralblatt initially covered only German literature, and while it expanded its coverage in the early 20th century, the emphasis was always on German material. Chemisches Zentralblatt remained an important abstracting service until World War II. It was the emphasis on German chemistry that prompted the interest in an American abstract service.
Noyes served as editor of Chemical Abstracts for its first two years. At first, Noyes worked from his laboratory at the National Bureau of Standards in Washington, where he was chief chemist. He and Chemical Abstracts moved to the University of Illinois when Noyes became chair of the chemistry department there in 1907. Noyes’ contribution to the success of Chemical Abstracts cannot be exaggerated. Not only did he persuade ACS to sponsor an abstract journal, but he organized, planned and edited the publication while serving as ACS secretary and working full time. Many of the policies he implemented served Chemical Abstracts for decades.
One of Noyes's most important legacies was his insistence that Chemical Abstracts cover applied as well as theoretical chemistry. In the early 20th century the gulf between industrial and academic or theoretical chemists was wide and many of the latter thought that ACS, and its publications, should belong to "pure" chemists only with applied chemists shunted off to a separate organization. In England and Germany chemists split along these lines and in both countries distinct abstract journals existed. Chemical Abstracts pioneered in the merging of the interests of these two groups of chemists.
In 1909 Austin Patterson succeeded Noyes as editor, and the editorial offices moved to The Ohio State University (OSU) in Columbus at the invitation of William McPherson, head of the OSU Chemistry Department. Chemical Abstracts remained at Ohio State until 1965, when the Chemical Abstracts Service moved into its own building on a 50-acre site adjacent to the university.
Back to top

The mission of CAS is to be the world's leading provider of chemical information." — Robert J. Massie, President, CAS
(Quoted in Ross, Linda Romaine, "CAS Aims to Keep Customers by Improved Service, Pricing, Management," Chemical & Engineering News 72 (October 31, 1994): 20.)
The early history of Chemical Abstracts is the story of Evan J. Crane, who served as editor for 43 years. Crane succeeded John Miller, editor for a brief time in 1914 following Austin Patterson's resignation. Crane joined Chemical Abstracts in 1911 at the age of 22 as an associate editor; he became acting editor in 1915 after Miller’s resignation.
During Crane's stewardship, Chemical Abstracts grew from a fledgling operation into the trendsetter for scientific abstracting and indexing journals. Crane did this through some very difficult times—money was often so scarce that he dipped into his own small salary for business travel. Crane himself wrote that it was his "long-established policy to trim his budget sails as well as possible to fit the Society's current circumstances and then to keep within budget limits." During his tenure he did that, never exceeding the publication’s annual budget.
Crane said that "in times of financial difficulty, keeping within limits [was] accomplished principally by shortening abstracts rather than by failing to report all papers and patents containing new information of chemical interest. The history of Chemical Abstracts is in part a series of ups and down as far as length of abstracts is concerned, with most of the emphasis on 'downs.'" Crane's perseverance in the face of financial and other difficulties insured the success of Chemical Abstracts . He won many honors, including the Society's Priestley Medal, but perhaps Crane's greatest honor came in 1956 when he was named the first director of the renamed Chemical Abstracts Service (CAS), a new division of the American Chemical Society.
Under Crane's tutelage, Chemical Abstracts grew rapidly. In its first year of operation, 1907, the service published 12,000 abstracts. Half of those were on works published in Germany. The number of abstracts published rose almost every year after and the service soon claimed complete coverage of the chemical literature. Of course, the definition of "completeness" is itself abstract; as Crane wrote "completeness in an abstract journal is a somewhat indefinite goal never quite attained, but the whole history of Chemical Abstracts has been one of striving for complete coverage so that the user of this journal can have confidence in the thoroughness of his survey without searching elsewhere." He defined completeness as reporting all suitable papers and patents, insuring that each abstract is thorough to allow full indexing, and guaranteeing that the index contains all the useful information that a searcher needs.
Completeness meant, among other things, that the number of abstracts published each year soared. It was not until 1939—32 years after publishing began—that the number of abstracts published reached one million; it took eighteen years to generate the next million; eight years for the third million. In 2006, for the first time in one year, more than one million abstracts were added to the CAS databases.
Crane recognized early in his tenure that comprehensive and accurate indexes were necessary. Cursory author and subject indexes were provided from the beginning, but Crane undertook to release a thorough Chemical Abstracts Decennial Index in 1916, doing much of the work himself. This project prompted a search for a more thorough system of indexing. The solution was to devise a systematic index for compounds on the grounds that the absence of such a system would mean that references to compounds would be scattered throughout the index and related compounds would not be grouped together. The indexing system had an important influence on chemical nomenclature.
Over the years indexing assumed increased importance because of the volume of material being abstracted. An annual formula index was added in 1920. Other indexes were added: for example, numerical patent indexes and a keyword subject index. The amount of material abstracted became so large that in 1962 Chemical Abstracts began publishing Volume Indexes semiannually rather than annually.

Crane retired in 1958. He was replaced by Dale Baker, who became Director, while Charles Bernier was appointed Editor and Leonard Capell, CAS' nomenclature expert, was named Executive Consultant. The most important task facing these three men was to oversee CAS' transition from an ACS-supported service to a financially independent operation. In the beginning, ACS-member dues financed Chemical Abstracts and Society members could receive it free. In 1933 a small subscription fee was charged, as a supplement to the allocation from dues, a system that satisfied CAS' financial needs for a time.
After World War II, this financial arrangement proved inadequate. The rapid expansion of scientific publications—which meant more abstracts—coupled with post-War inflation forced CAS to look elsewhere for funding. For a time, industry was asked to make up the service's deficit. In 1955, the ACS Board of Directors stepped in and changed subscription prices with the aim that Chemical Abstracts should break even. Fees were raised so that, in the words of Board Chair Ernest Volwiler, Chemical Abstracts would become "a joint responsibility of the profession and of those governmental, industrial, and commercial organizations that have a direct stake in its availability."
The post-Crane leadership had a second problem to tackle—housing for a rapidly expanding organization. Since 1909, CAS had been housed on the OSU campus, first in a 15-by-30 foot room, then a room twice that size, and in 1928 in 1,600-square foot section of McPherson Chemistry Laboratory. In the 1950s, when CAS had a staff of 100, a building jointly financed by ACS and OSU was erected on campus to house the abstract service. In 1965—now with a staff of 300—CAS moved into a four-story, multi-million dollar building of its own on a 50-acre site adjacent to OSU's campus. Less than a decade later CAS added a second building.
Until the 1960s, Chemical Abstracts relied on volunteers for the bulk of its abstracting. In 1907, Chemical Abstracts utilized 129 volunteer chemists; by the mid-1960s the number of volunteers reached more than 3,200. Crane referred to some of the volunteers as "the iron men of CA [ Chemical Abstracts ]," and many served for decades, with thirteen volunteers abstracting for more than 50 years. Over the years the abstractors took on an international caste as chemists from 70 nations contributed entries. In the mid-1960s, CAS began to phase out its use of volunteers, and in 1994 it entirely ceased using volunteer abstractors.
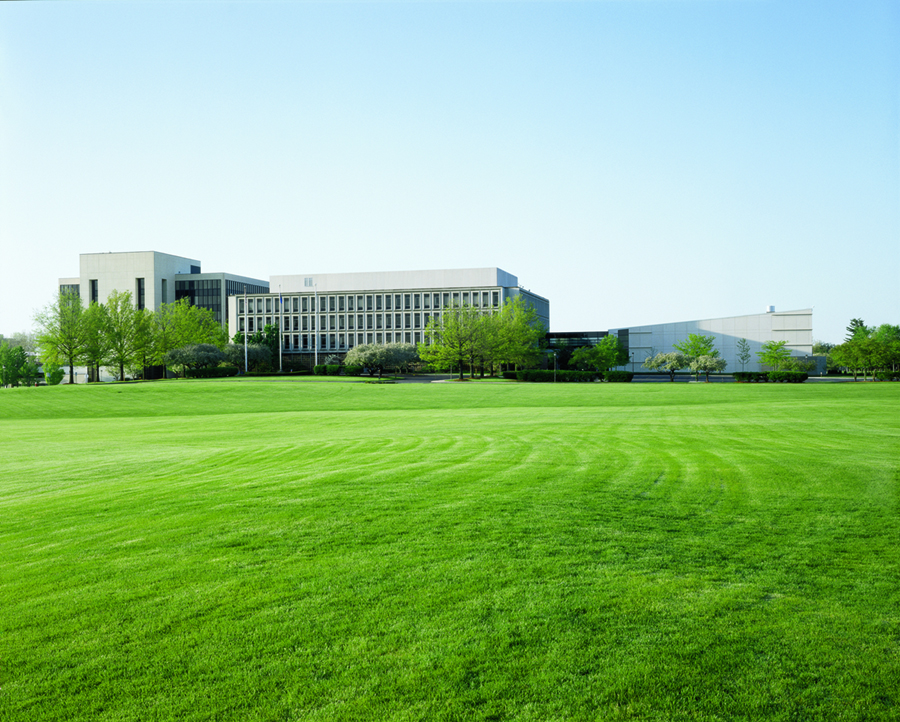
The decline in use of volunteers corresponded to CAS' entry into the digital age. Baker and the rest of the CAS leadership understood the need to modernize procedures due to the vast increase in chemically-related published material. Older methods for producing abstracts did not suffice by the 1960s. On one level, this meant professionalizing of the staff; at another, it meant new ways of processing information.
CAS had been moving into the information age slowly. In 1955 it established a research and development department. In 1959 Baker hired G. Malcolm Dyson, an Englishman who had worked on an early linear notation system for representing chemical structures. It was Dyson—working part-time in Columbus from 1959 to 1963—who developed many of the cutting-edge innovations in information processing that CAS introduced in these years, starting with Chemical Titles in 1961. Chemical Titles was the first periodical to be organized, indexed and composed by computer, which meant greater publishing speed. The issue of Chemical Titles listing a given article often reached subscribers before the journal in which that article appeared.
CAS information needs began to drive the technology. For example, CAS required a composition system that did not yet exist. This problem was solved in 1967 when CAS acquired an IBM unit and then developed a software-based, computer-driven composition system for it. It was put to good use, and by 1970 all Chemical Abstracts indexes were being organized and composed by computer. Computerization meant considerable savings in staff time and costs. It also meant more precise data; in addition, staff no longer had to do the routine fact checking which could now be done by the computer. This freed staff for the more intellectual tasks of analyzing the primary chemical literature. For chemists, it meant ease and speed. Information seekers in the past might have to wait a day or more for a librarian to find the appropriate references (only to find sometimes that the references were not appropriate). With computers, the chemist could do his own searching and do it quickly and accurately.
CAS revenues sources shifted with computerization. In 1975 95% of CAS' revenues came from print services; only 5% from electronic sources. By the end of the 20th century, that had shifted to 19% of revenues from printed sources with 79% from electronic services (the remaining 2% came from consulting and other sources). In 2006 95% of CAS' revenue stream derived electronic sources with the remaining 5% from print and other services.
The mechanization of information yielded CAS' most significant and far-reaching innovation: The Chemical Registry System. G. Malcolm Dyson first suggested the idea of a registry in the late 1950s. With the increasing complexity and sheer numbers of substances appearing in the literature, CAS needed an alternative to chemical nomenclature as a basis for this registry.
Chemists have an advantage over many other scientists in being able to communicate by referring to chemical compounds through unambiguous and universally understood structural diagrams. These diagrams indicate a compound's chemical atoms, the bonds that connect them, and even the spatial arrangement of the atoms. But there was no good way to catalogue these structures that enabled easy retrieval. At CAS, every time a chemical compound was indexed it was copied by hand and then given a name, which may or may not have agreed with the name given it by its presumed discoverer. Was the compound really new? Had it been reported in the literature? Indexed by Chemical Abstracts ? The rapid increase in the number of named structures led to much confusion; some compounds were named over and over.
Clearly, CAS needed a system that recognized previously named structures and allowed for easy retrieval by CAS staff. In the early 1960s a solution was found when Harry Morgan of CAS developed an algorithm that generates a unique and unambiguous two-dimensional record of a substance's structure. The record could be read by a computer and was so simple that staff with little special training could employ it. The algorithm became the basis of the CAS Chemical Registry System (CAS Registry).
In late 1964 an experimental Chemical Registry System went into operation supporting the new publication Chemical-Biological Activities . By early 1965, the CAS Registry, no longer experimental, began to include all chemical substances indexed in Chemical Abstracts , with their structures and names recorded in computer-readable files. Every substance was given a permanent, unambiguous and unique identifying number, the CAS Registry Number.
To show the usefulness of the CAS Registry to researchers, think about a hypothetical new drug developed by a pharmaceutical company. This drug could have a systematic chemical name about 70 characters long, and be marketed under several different trade names. But it can be identified concisely by CAS Registry Number of up to 10 digits, such as 58-08-2 for caffeine. Today scientists and researchers around the world rely upon the CAS Registry Number as the globally accepted standard for defining and describing a chemical substance.
Though the system would go through several iterations over the years, it quickly became an integral part of CAS’s indexing procedures. More advanced versions were soon added which refined and improved nomenclature and the ability to recreate structure diagrams algorithmically. It took only ten years for the Registry to record three million unique structures. But the Registry's virtue is not limited to size or number; its success has eliminated the vagaries of chemical nomenclature since every unique substance gets a unique CAS Registry Number.
In the 1970s and 1980s the Chemical Abstracts Service adopted the tools of the new digital age—the computer, the internet, etc.—to assist CAS in fulfilling its core mission: Providing scientists with access to chemical literature and information. What changed were the methods of delivery (away from paper and traditional forms of publishing) and the speed with which CAS was able to deliver information to clients. In the past, chemists needing information might have to wait a day or several days for a librarian to find the requested references, which might not be entirely relevant. But in the digital age, scientists are only seconds from the information sought. Not only is information retrieval faster, but it can be done by the scientist himself.
CAS entered the electronic information age slowly. Before the 1980s online access to CAS databases went through commercial vendors. On November 1, 1980, CAS introduced CAS ONLINE ® , which provided access to about one-third of CAS's substance records. Gradually, other segments of the CAS Registry were added as search capacity increased. Although initially limited, CAS ONLINE ® permitted bench chemists and information experts to identify substances by specified molecular structures, something difficult, if not impossible, to do with printed materials. Although searching has been augmented over the years and the display of CAS Registry records has improved, the most remarkable advantage researchers derive from CAS ONLINE ® remains the ability to perform structure-based searching of the CAS database.
In 1983 CAS introduced CA File, which enabled subscribers to retrieve post-1967 bibliographic references. Other enhancement followed, but by the 1980s CAS began planning a new online network, one that grew out of an agreement between the American Chemical Society and the German scientific organization, FIZ Karlsruhe, to create an international network of databases to include areas beyond chemistry and chemical engineering. The result was STN SM International—the Scientific and Technical Information Network.
STN ® offered access to CAS files and Physics Briefs. Initially, the creation of STN ® provided European customers access to CAS files and search systems. Eventually, CAS databases would become available globally. As the CAS web site puts it: "STN ® is an online database service that provides global access to published research, journal literature, patents, structures, sequences, properties, and other data."
Dale Baker, who had ushered CAS into the digital age, retired in 1986 after serving as director for 28 years. Ronald Wigington, who had been director of research and development, succeeded Baker as director with no change in the emphasis on moving CAS toward the delivery of electronic information services. Wigington left in 1991 and was replaced a year later by Robert Massie.
Massie's first challenge was to insure CAS' financial well-being. At the same time, he wanted to guarantee CAS' leadership in providing scientific information. To further the latter, CAS moderated its price increases—to keep old and attract new customers—and strengthened product development. This meant even greater emphasis on electronic services in the 1990s as CAS embraced the Internet and World Wide Web as tools for distributing information. In 1995 CAS introduced CAplus SM , a database covering literature worldwide in chemistry, chemical engineering, biochemistry and related sciences since 1967.
A major launch in the 1990s was SciFinder ® . Much research went into the development of this forward-looking tool. Although the Internet was still in its infancy, CAS recognized the need for a new mode of data searching and retrieval. The rapid increase in personal computers meant that it would be possible to put chemists in direct connection with CAS databases. In 1991, CAS began to look into creating a desktop research tool, and the next year created a product development team. This group solicited views from CAS staff members and from customers. From users, the CAS researchers learned that scientists wanted more control over research and direct access to information. Moreover, the team understood that most scientists were not familiar with the language of information retrieval; accordingly, the search mechanisms had to be conversational and intuitive. In other words, users should not be asked to learn a command language, but rather they should be able to sit at their computer and use the system without training.
After extensive testing of prototypes by pharmaceutical and chemical companies, SciFinder ® was launched in 1995. From the beginning it allowed for simple interaction with the search system. The complex algorithms and other tools that buttress the CAS Registry and other CAS files remain in the background. In 1997 SciFinder ® Scholar TM appeared as a version of SciFinder ® for chemistry students and faculty.
CAS quickly adapted to the online environment as it became popular in the closing years of the 20th century. Web-based initiatives undertaken by CAS included creation in 1994 of its website, http://www.cas.org ; introduction of Chemical Patents Plus in 1996, which covers U.S. patents since 1974; STN ® Easy, also in 1996, to make access to some STN ® databases even easier; ChemPort ® in 1997, a joint service with ACS Publications to provide links from the records in the searchable databases to full-text journals and patents on the Web; a whole host of new STN ® products, including STN ® Express™, STN ® AnaVist™, STN ® on the Web SM ; and many others.
By 2007, when CAS celebrated its 100th anniversary, its databases demonstrated the exponential growth in scientific publishing and research. Those databases contained more than 27 million records of journal and patent literature, more than 170 million citations and more than 30 million substance records in the CAS Registry. In the words of Robert Massie, "CAS celebrates one hundred years of service to world science as an integral part of the American Chemical Society. That is a century in pursuit of a single mission—to provide access to chemical and related information that speeds and enables scientific discovery to improve people's lives."
- Chemical Abstracts Service History (Chemical Abstracts Service/ACS)
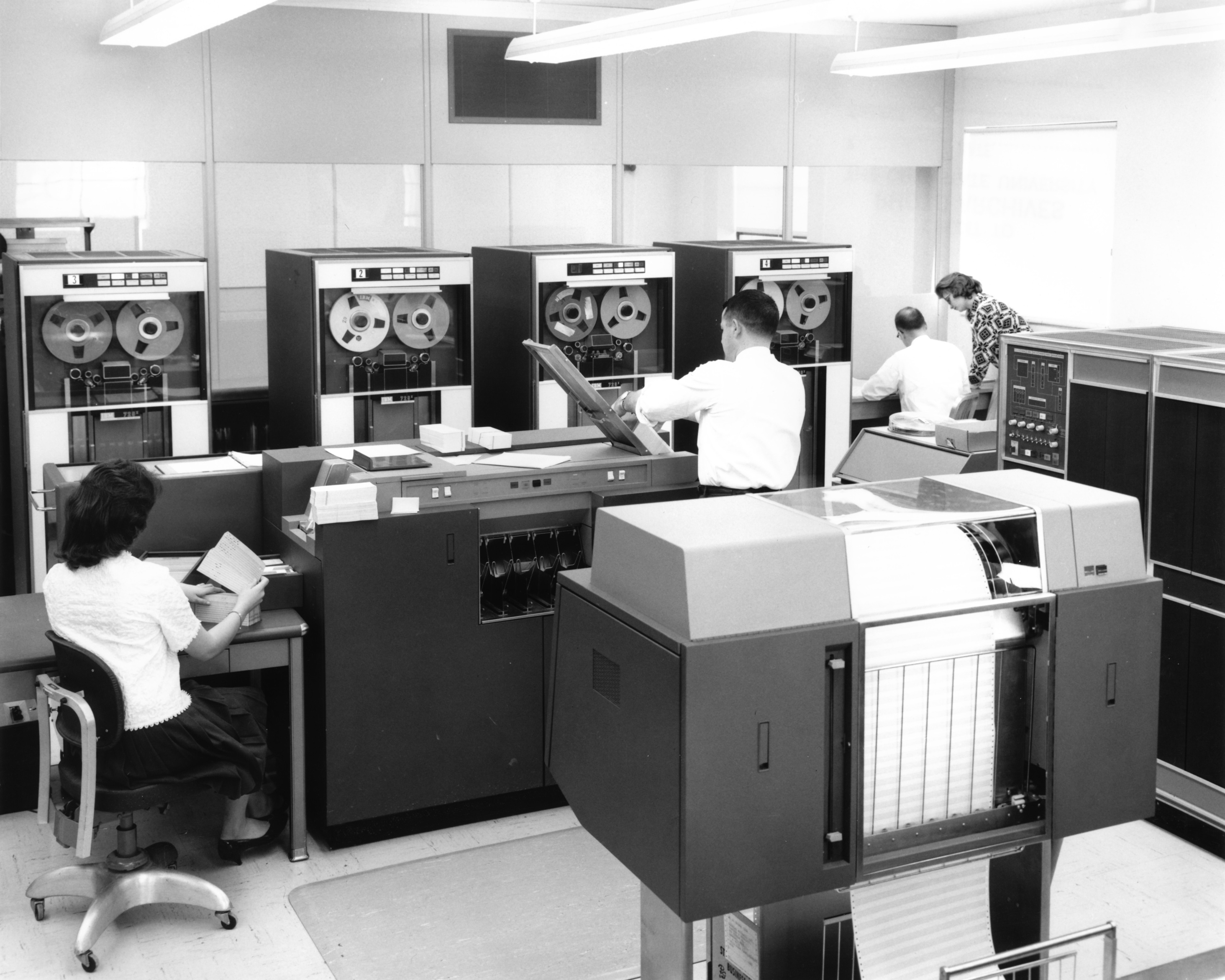
Landmark Designation
The American Chemical Society designated the Chemical Abstracts Service a National Historic Chemical Landmark in a ceremony at CAS headquarters in Columbus, Ohio, on June 14, 2007. The text of the commemorative plaque reads:
Founded in 1907 with the first publication of Chemical Abstracts TM , CAS has provided generations of scientists with unparalleled access to the most comprehensive collection of chemical information. The CAS Chemical Registry SM , established in 1965, is the recognized global standard for chemical substance identification and the largest database of chemical substances in the world. CAS continues to pursue its mission to provide access to chemical and related information that speeds and enables scientific discovery to improve people's lives .
Also on June 14, 2007, the American Chemical Society presented a plaque to The Ohio State University for its support of CAS, which was located on the OSU campus for many years. The text of the plaque presented to OSU reads:
From 1909 to 1965, The Ohio State University provided the American Chemical Society a range of support services and facilities that allowed the nascent Chemical Abstracts TM publication to grow into the world's most important resource for chemistry-related research. CAS moved to its own campus in 1965, adjacent to The Ohio State University, and cherishes the memory of that half century of generous and farsighted support.
Acknowledgments
Adapted for the internet from “Chemical Abstracts Service (CAS),” produced by the National Historic Chemical Landmarks program of the American Chemical Society in 2007.
American Chemical Society National Historic Chemical Landmarks. Chemical Abstracts Service (CAS). http://www.acs.org/content/acs/en/education/whatischemistry/landmarks/cas.html (accessed Month Day, Year).

Accept & Close The ACS takes your privacy seriously as it relates to cookies. We use cookies to remember users, better understand ways to serve them, improve our value proposition, and optimize their experience. Learn more about managing your cookies at Cookies Policy .
1155 Sixteenth Street, NW, Washington, DC 20036, USA | service@acs.org | 1-800-333-9511 (US and Canada) | 614-447-3776 (outside North America)
- Terms of Use
- Accessibility
Copyright © 2024 American Chemical Society

- University of Texas Libraries
- UT Libraries
- Chemical Abstracts
- For New Researchers
- Literature Tutorial
- Support the Library
- Science of Synthesis
- Landolt-Börnstein
- Gmelin Handbook
- Beilstein Handbook
- Textbooks & OER
- Conferences & Symposia
- Tech Reports
- Historical Information
- Free Resources
- Analytical Chemistry
- Biochemistry This link opens in a new window
- Crystallography
- Electrochemistry
- General Chemistry
- Inorganic Chemistry
- Organic Chemistry
- Physical Properties
- Polymers and Plastics
- Safety, Hazards, Environment
- Spectroscopy

- History of Chemical Abstracts ACS Chemical Landmark series.
- Organization
- CA Abstract Numbers
- For Librarians
Abstracts There were 26 weekly issues per semiannual "volume." Each Abstract issue was divided into 80 Subject Sections. An abstract appeared in just one section, based on the novelty of the process or substance being reported in the literature. Each weekly issue also contained indexes by author, subject keyword (not official headings), and patent number. The issue indexes were superseded first by a volume index published every six months, and then by the 5-year Collective Index. (The library did not retain the issue and volume indexes.)
Collective Indexes Every five years CAS published a Collective Index (CI). The 14th CI was published in 2002 and covers the years 1997-2001. The library has all Collective Indexes up to this point. They are divided into:
- Author Index , 1907-2001
- Subject Index 1907-71 (included chemical substance names through 1971)
- Chemical Substance Index , 1972-2001 (includes all CA Index Names used during the specific index period)
- General Subject Index , 1972-2001 (includes all subject and compound-class terms that are not systematic CA Index Names)
- Formula Index , 1920-2001
- Patent Index , 1907-2001
Index Guides The Index Guide (IG) for each Collective Index period provides cross-references from commonly used chemical names to official CA Index Names (with registry numbers) used in the corresponding Chemical Substance Index. It also serves as a thesaurus of all controlled-vocabulary subject headings used in the General Subject Index. The Index Guide should always be consulted before looking up a chemical name or subject term in the Collective Indexes.
Ring Systems Handbook The RSH leads you from a ring or cage structure to the CA Index Name and Registry Number of a ring parent compound, for searching in the Chemical Substance Index. Entries are in ring analysis order and are indexed by molecular formula and Index Name.
Registry Handbook The Registry Handbook - Number Section was a cumulative numerical listing of Registry Numbers assigned to chemical substances from 1965 to 1996. If you have only a registry number and need the CA Index Name for that compound, look it up here first and then use the name to consult the Chemical Substance Indexes. A corresponding Names Section issued on microfiche provided registry numbers for several hundred thousand of the most-indexed common names.
CASSI CASSI (Chemical Abstracts Service Source Index) is the comprehensive and retrospective list of publications that have been indexed by Chemical Abstracts since it began in 1907. It includes journals, books, conferences, and other series, arranged by CA abbreviation. This is the source you use to translate journal title abbreviations into full titles for searching in the library catalog and other finding aids. The last print edition of CASSI (1907-2004) is kept in the Librarian's office. It is also available in a somewhat limited form on the web:
Doing a manual search in printed Chemical Abstracts is a tedious, mutli-step process. This is how it was done.
- Author: Entries are arranged by last name, then by first and second initials (not by first name). Qualifying text is the title of the document. Coauthors are cross-referenced to first author.
- Formula: Entries contain only abstract numbers unless there is a large number of them, and no qualifying text. It's best to use the Formula Index to get the corresponding CA Index Name, then look up that name in the corresponding Chemical Substance or Subject (1907-71) index, where the entries are more detailed. Formulas are listed in Hill order: C, then H, then other elements in alphabetical order.
- Chemical Substance name: Start with the Index Guide to see if there's an entry for the name you have. If not, use the Formula Index or Ring Systems Handbook to get the name. In the CSI you must use only the specific CA Index Name for that CI period. There are no cross references to earlier or generic names. Names are arranged by "parent" (the structural skeleton) followed by substituents and modifications. Qualifying text in each entry indicates what the document is primarily about, followed by an abstract number. About 600 of the most frequently indexed compounds are called "Qualified Substances." Their document entries are grouped into seven categories: Analysis, Biological studies, Occurrence, Preparation, Properties, Reactions, Uses and miscellaneous.
- Subject term: Check the Index Guide first to find an appropriate term to look up in the Subject Index (1907-71) or General Subject Index (1972- ). Classes of compounds (e.g. Carcinogens), undefined compounds and mixtures (e.g. Gasoline), processes, plant/animal species, and other general topical terms are found in this index, along with cross references and scope notes.
- Patent number: Arranged by issuing country/organization, then by patent number. CA abstracts only the first member of a patent family, and links later equivalent patents to this parent patent. Equivalents are cross-referenced to the parent. Prior to 1981 the equivalents were listed in the Patent Concordance.
- Note Abstract Numbers from the entries of interest. Abstract numbers prefixed "R" indicate a review; "P" indicates a patent.
- Go to the corresponding Abstracts volume and look up the abstract by its number.
- Repeat this process for earlier or later index periods. Remember that Index Names and subject headings changed over time, so consult the Index Guide for each CI period.
For Librarians: Retention of Chemical Abstracts

- Here's the most important consideration: It's highly unlikely that any scientist born after the mid-1970s would have any experience using print CA (or any printed index for that matter), or even be aware of its existence. Therefore academic libraries should expect all potential use to be initiated and mediated by a library staff member or senior faculty member who has working knowledge of this tool. If no such persons remain on the campus, then print CA is almost certainly a waste of space. (Similarly, there is no longer any practical reason to teach students how to use it!)
- SciFinder is not identical to Chemical Abstracts. All (or nearly all) the metadata content of the latter is included in the CAPLUS file and robustly substance-indexed via the Registry file. But it is inaccurate to say that you can do everything in SciFinder that you could do in the print.
- The Collective subject/substance/formula indexes allow browsing of chemical names, formulas, and subject headings in a way that isn't possible in SciFinder. SciFinder is great for snapshots, but it provides only a limited view of the hierarchical structure of the CA database, or its indexing and nomenclature practices; nor does it allow easy browsing for derivatives, salts, and other variants of a parent structure. In other words, you can't browse online for nearby entries like you can in the print, which removes a serendipity factor. For some purposes, this is an important distinction. Browsing and searching CA indexing terms for concepts, chemical classes, and taxonomic vocabulary from the CAS Lexicon (thesaurus) is possible in SciFinder, as of 2023.
- When you can't figure out how CAS has defined the structure or formula of certain types of compounds, especially inorganic (salts, hydrates, ions, decimals, etc), coordination compounds, and multicomponent substances, SciFinder can be frustrating. Using the Index Guide and Chemical Substance Index can actually save some time, and when you find the Registry number then you can go back to SciFinder, locate the substance record and complete the literature search. (Of course, this method only works for compounds registered before your last Collective Index.)
- Pre-1967 CA abstract numbers are not searchable or displayed in SciFinder, and can only be looked up or verified in the print. These numbers were occasionally cited in the older literature, especially as stand-ins for obscure and foreign documents.
- Some older printed abstracts may contain structure graphics that aren't duplicated online.
- If you have bound any of the six-month volume indexes, and you have the equivalent Collectives and their Index Guides, the former are expendable and should be discarded to save space. And hopefully the indexes in the back of the weekly issues were sliced out and discarded before binding -- those are indeed useless and add a significant amount of linear footage.
- Production of printed CA ceased in 2009, and the hardcopy is now only applicable to historical searching. It is not a viable substitute for any form of current online searching.
- Even if you decide to discard the bulk of CA, consider retaining the most valuable parts, such as the Index Guides (potentially useful for finding contemporary index terms, synonyms, controlled vocabulary, Registry Numbers, etc.). If you wish to split the run by time period, collective wisdom suggests that the older (and smaller) pre-1967 portion of CA is more useful than the post-1967 volumes.
- If the facility lacks space and staff who can retrieve and consult CA volumes to mediate a reference question, stored CA can't be used as designed.
- If storage space is at a premium, it's difficult to justify the space CA would occupy there. (A complete set of CA with indexes can occupy as much as 1000 linear feet of shelving, depending on how a library has bound it.) The trend toward shared/consortial storage may allow multiple institutions to share a single print copy.
- If the item-specific metadata in your catalog don't include abstract number ranges -- as opposed to issue numbers, which are useless -- remote usage/retrieval of CA volumes becomes even more problematic and impractical.
- ACS does not require institutions to retain print CA for chemistry program approval. (There's no requirement for SciFinder either. See ACS Committee on Professional Training guidelines for more information.)
- Last Updated: May 6, 2024 9:41 AM
- URL: https://guides.lib.utexas.edu/chemistry
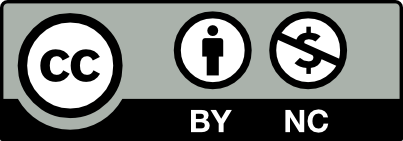
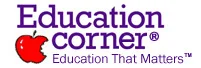
68 Best Chemistry Experiments: Learn About Chemical Reactions

Whether you’re a student eager to explore the wonders of chemical reactions or a teacher seeking to inspire and engage your students, we’ve compiled a curated list of the top 68 chemistry experiments so you can learn about chemical reactions.
While the theories and laws governing chemistry can sometimes feel abstract, experiments bridge the gap between these concepts and their tangible manifestations. These experiments provide hands-on experiences illuminating the intricacies of chemical reactions, molecular structures, and elemental properties.
1. Covalent Bonds
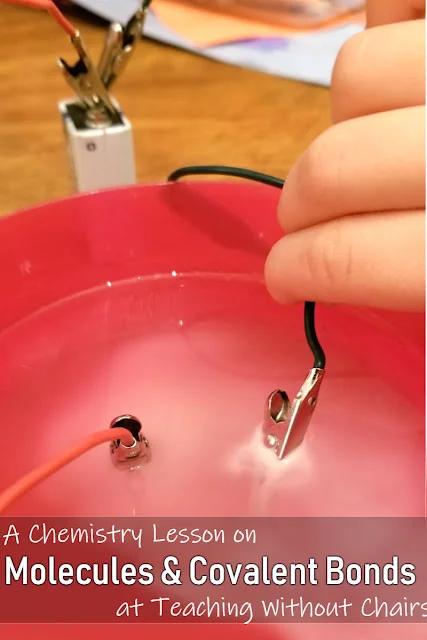
By engaging in activities that demonstrate the formation and properties of covalent bonds, students can grasp the significance of these bonds in holding atoms together and shaping the world around us.
Learn more: Covalent Bonds
2. Sulfuric Acid and Sugar Demonstration
Through this experiment, students can develop a deeper understanding of chemical properties, appreciate the power of chemical reactions, and ignite their passion for scientific exploration.
3. Make Hot Ice at Home
Making hot ice at home is a fascinating chemistry experiment that allows students to witness the captivating transformation of a liquid into a solid with a surprising twist.
4. Make a Bouncing Polymer Ball
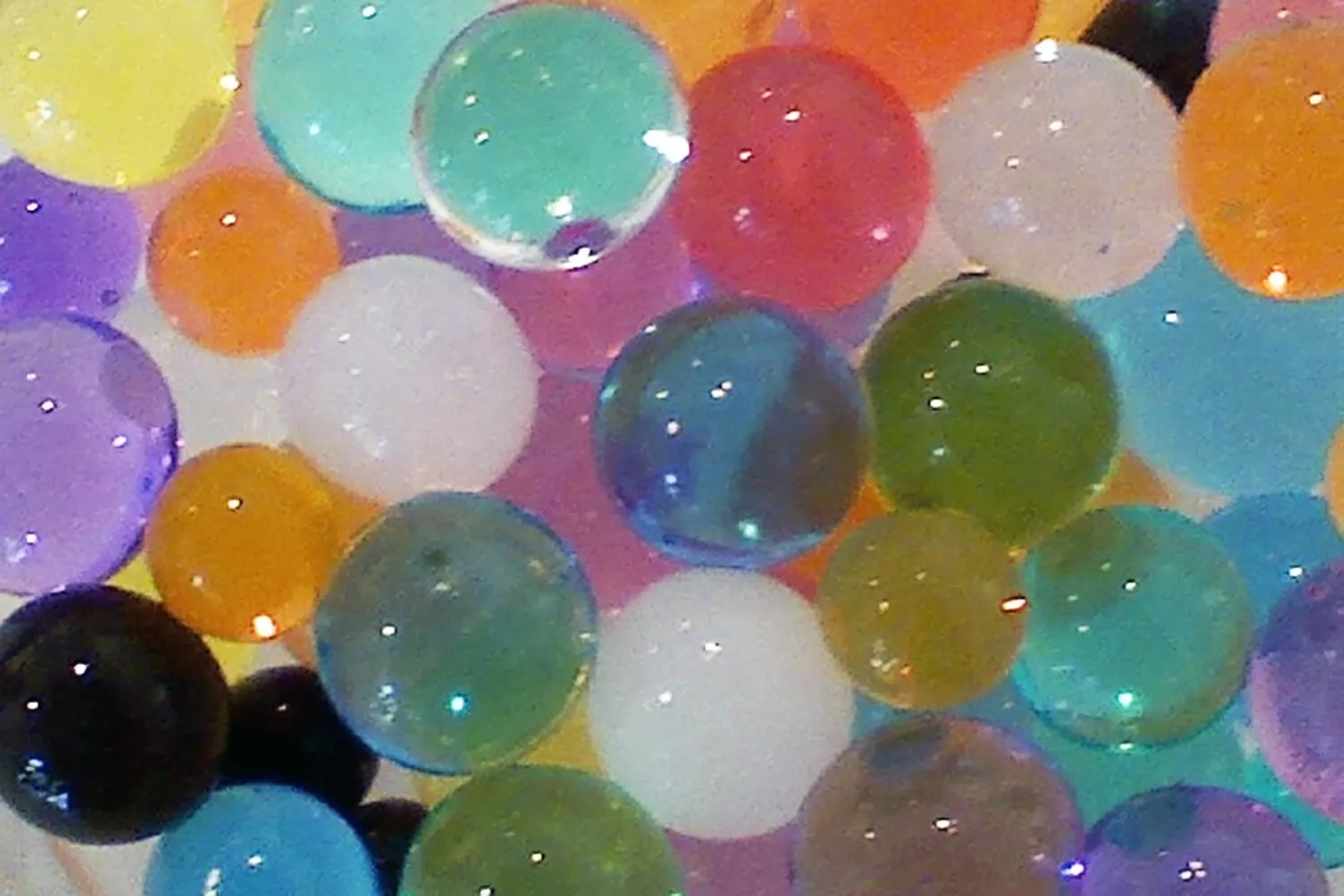
This hands-on activity not only allows students to explore the fascinating properties of polymers but also encourages experimentation and creativity.
Learn more: Thought Co
5. Diffusion Watercolor Art
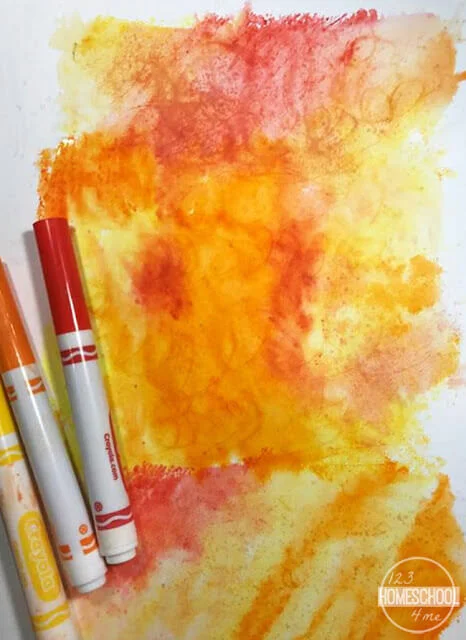
This experiment offers a wonderful opportunity for students to explore the properties of pigments, observe how they interact with water, and discover the mesmerizing patterns and textures that emerge.
Learn more: Diffusion Watercolor Art
6. Exploding Baggie
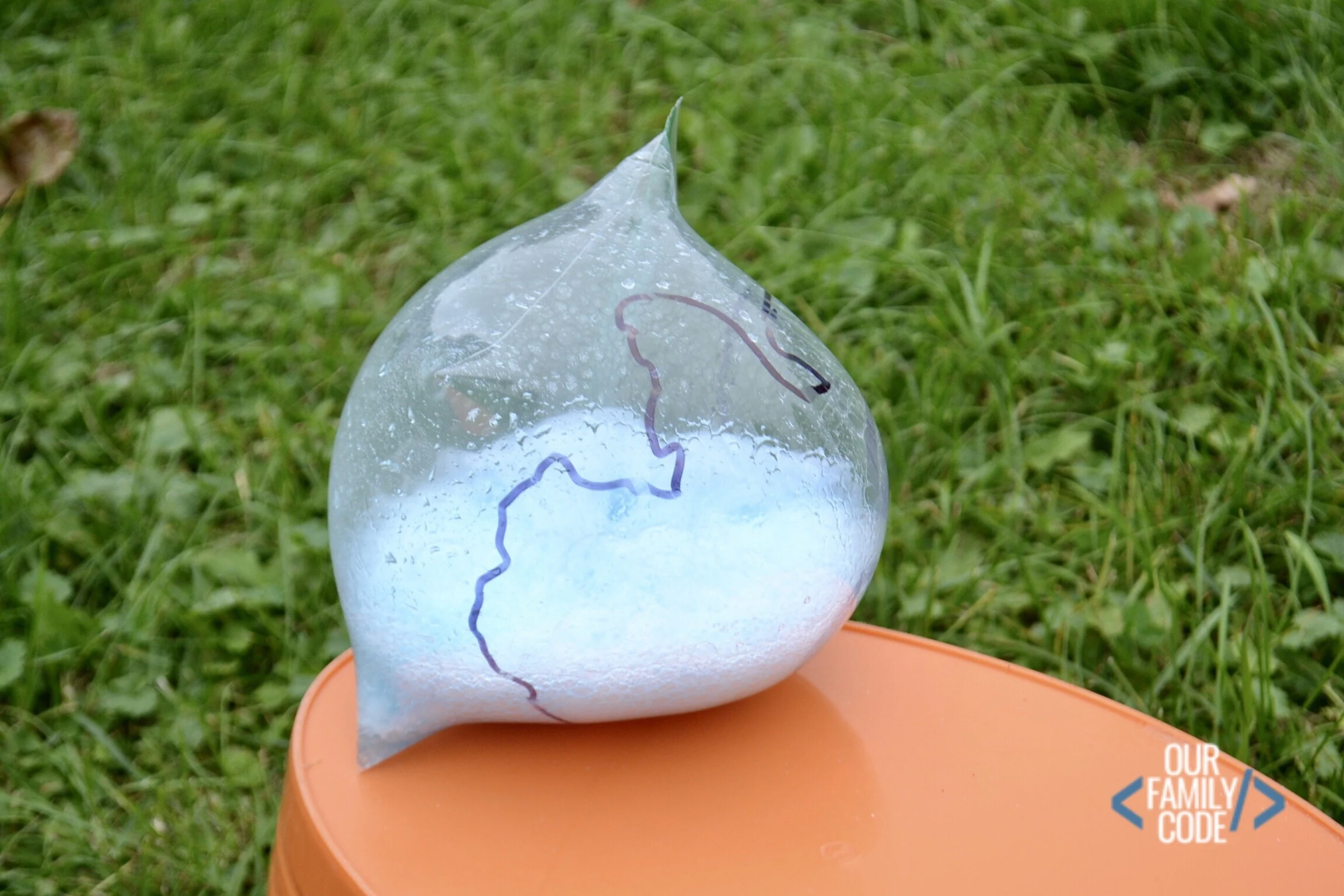
The exploding baggie experiment is a captivating and dynamic demonstration that students should engage in with caution and under the supervision of a qualified instructor.
Learn more: Exploding Baggie
7. Color Changing Chemistry Clock
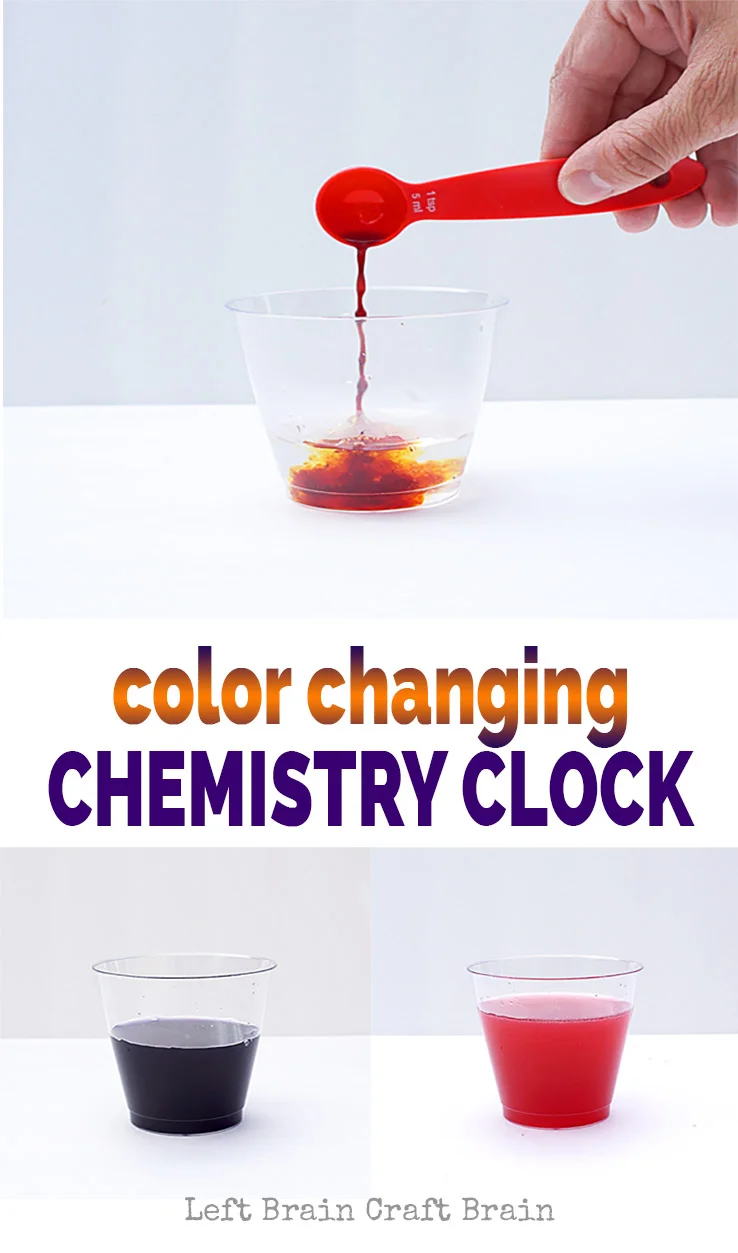
This experiment not only engages students in the world of chemical kinetics but also introduces them to the concept of a chemical clock, where the color change acts as a timekeeping mechanism.
Learn more: Color Changing Chemistry Clock
8. Pipe Cleaner Crystal Trees
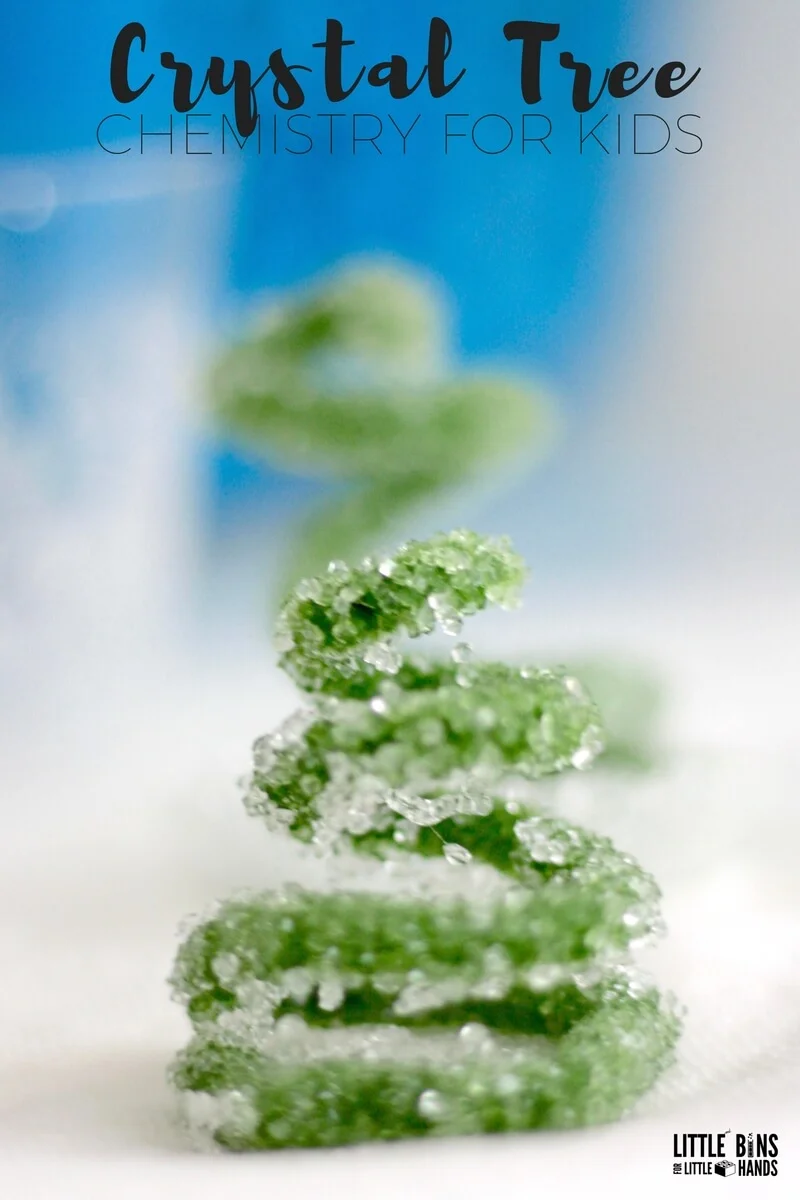
By adjusting the concentration of the Borax solution or experimenting with different pipe cleaner arrangements, students can customize their crystal trees and observe how it affects the growth patterns.
Learn more: Pipe Cleaner Crystal Trees
9. How To Make Ice Sculptures
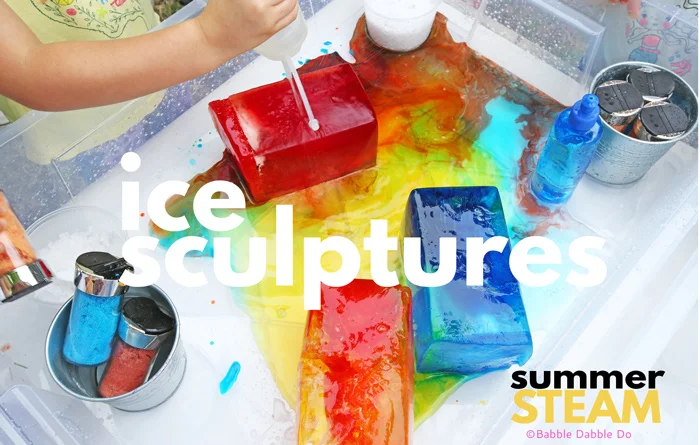
Through this experiment, students gain a deeper understanding of the physical and chemical changes that occur when water freezes and melts.
Learn more: Ice Sculpture
10. How to Make Paper
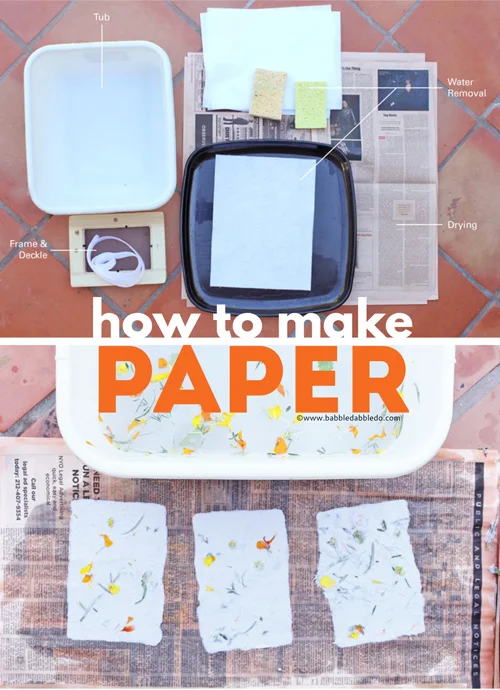
Through this hands-on activity, students gain a deeper understanding of the properties of cellulose fibers and the transformative power of chemical reactions.
Learn more: How to Make Paper
11. Color Changing Chemistry
Color changing chemistry is an enchanting experiment that offers a captivating blend of science and art. Students should embark on this colorful journey to witness the mesmerizing transformations of chemicals and explore the principles of chemical reactions.
12. Gassy Banana
The gassy banana experiment is a fun and interactive way for students to explore the principles of chemical reactions and gas production.
Learn more: Gassy Banana
13. Gingerbread Man Chemistry Experiment
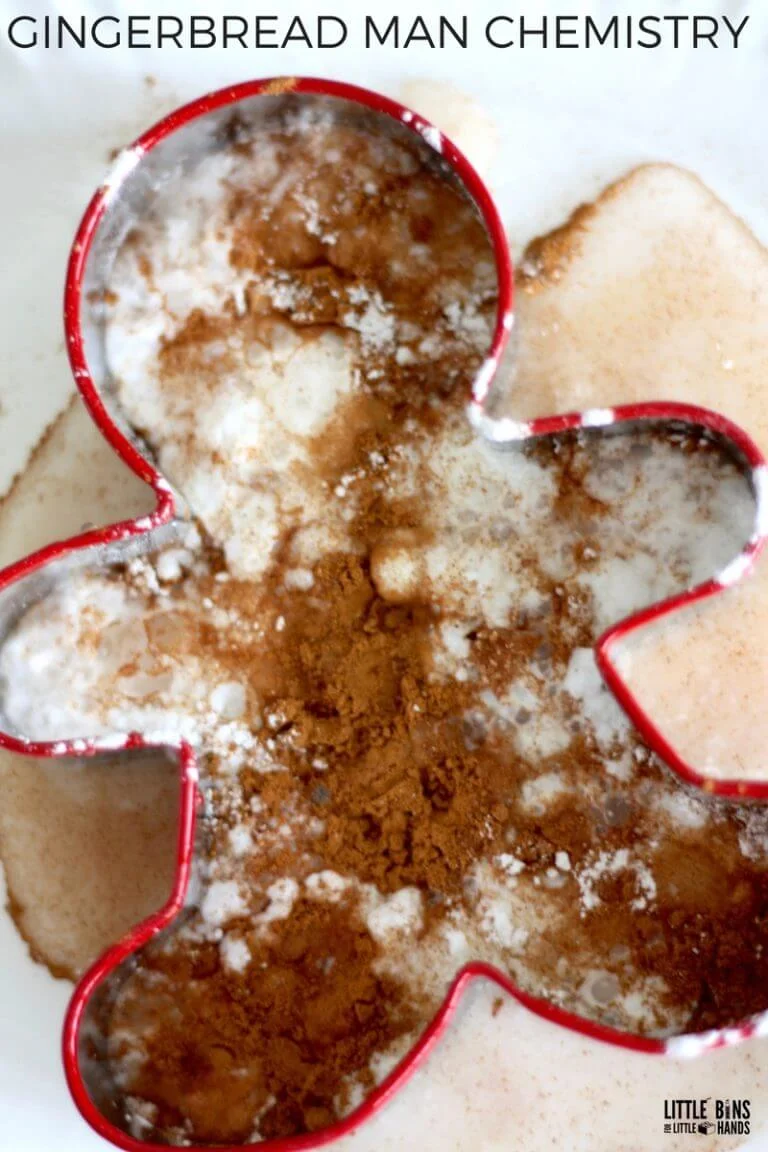
This hands-on activity not only introduces students to the concepts of chemical leavening and heat-induced reactions but also allows for creativity in decorating and personalizing their gingerbread creations.
Learn more: Gingerbread Man Chemistry Experiment
14. Make Amortentia Potion
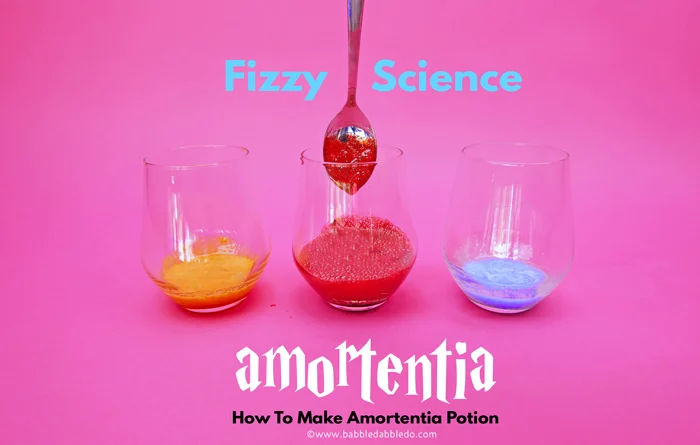
While the love potion is fictional, this activity offers a chance to explore the art of potion-making and the chemistry behind it.
Learn more: How to Make Amortentia Potion
15. Strawberry DNA Extraction
This hands-on experiment offers a unique opportunity to observe DNA, the building blocks of life, up close and learn about its structure and properties.
16. Melting Snowman
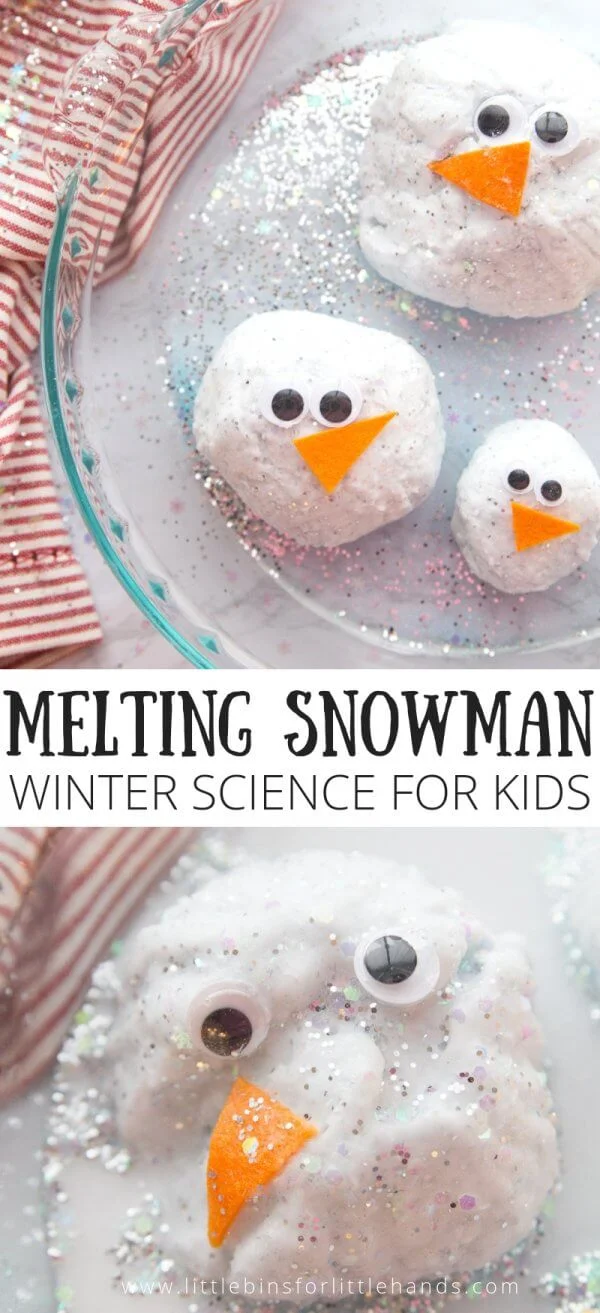
The melting snowman experiment is a fun and whimsical activity that allows students to explore the principles of heat transfer and phase changes.
Learn more: Melting Snowman
17. Acid Base Cabbage Juice
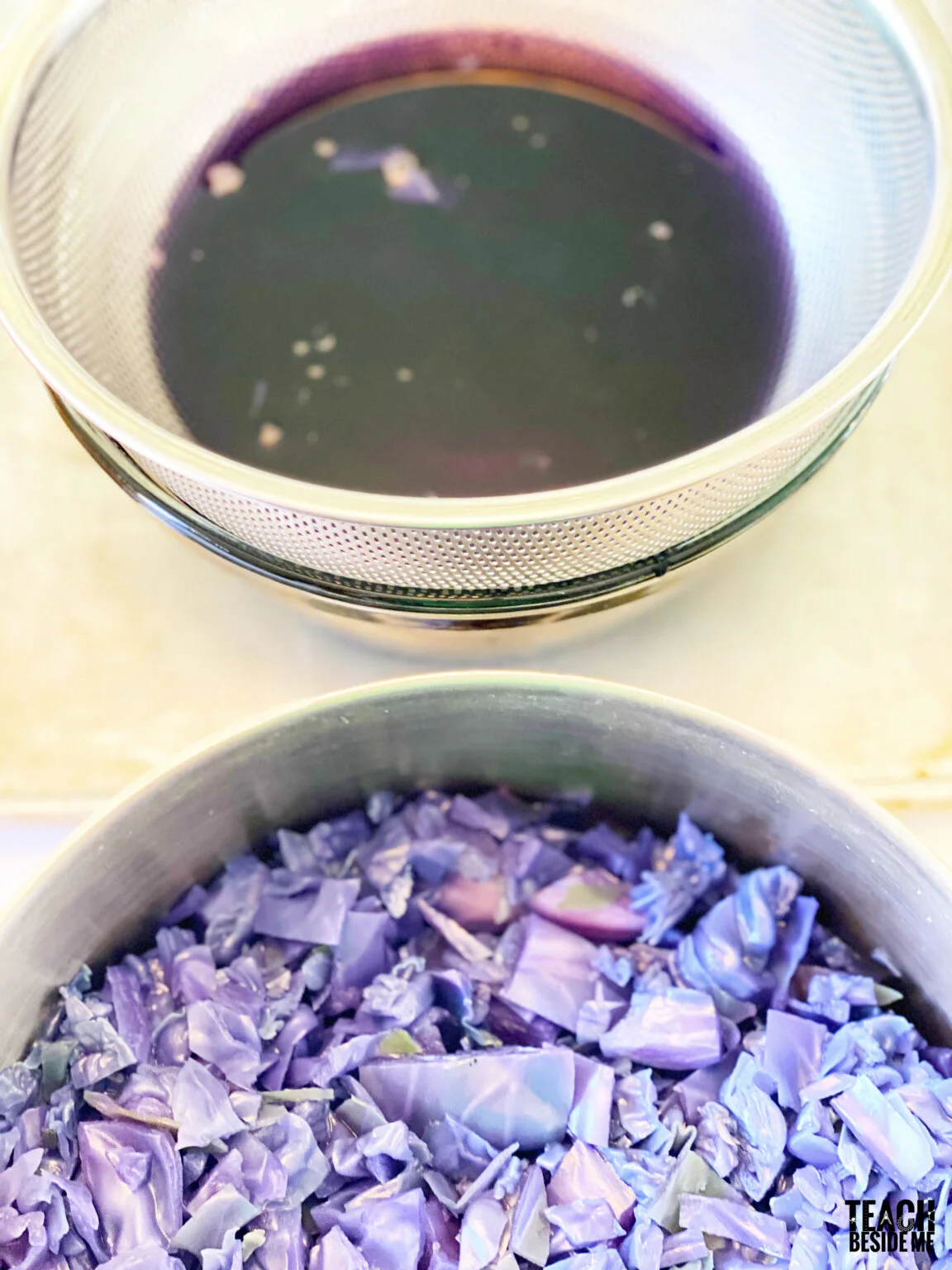
The acid-base cabbage juice experiment is an engaging and colorful activity that allows students to explore the pH scale and the properties of acids and bases.
By extracting the purple pigment from red cabbage leaves and creating cabbage juice, students can use this natural indicator to identify and differentiate between acidic and basic substances.
Learn more: Acid Base Cabbage Juice
18. Magic Milk
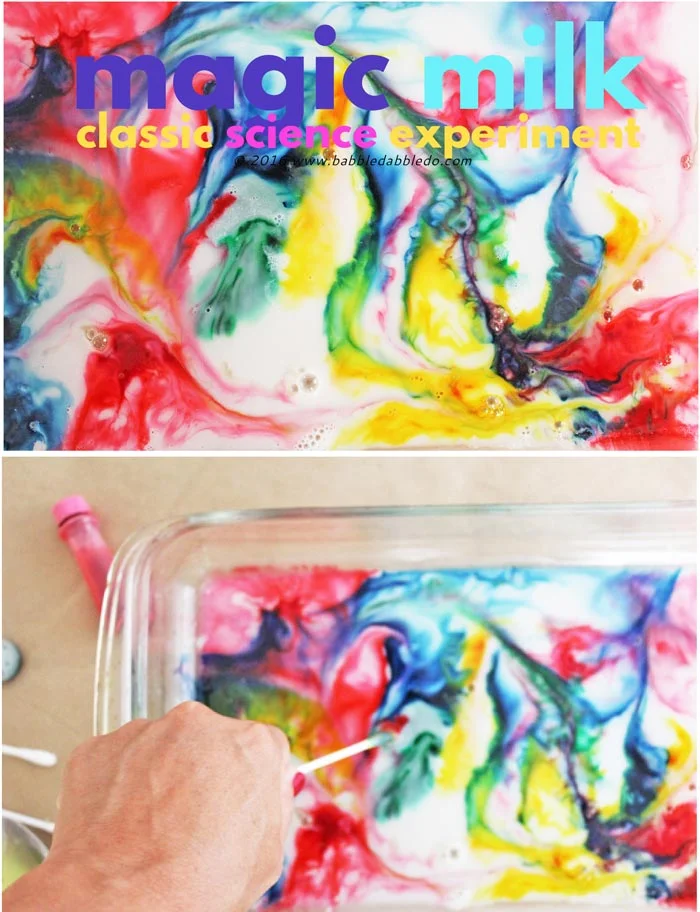
The magic milk experiment is a mesmerizing and educational activity that allows students to explore the concepts of surface tension and chemical reactions.
By adding drops of different food colors to a dish of milk and then introducing a small amount of dish soap, students can witness a captivating display of swirling colors and patterns.
Learn more: Magic Milk
19. Melting Ice with Salt and Water
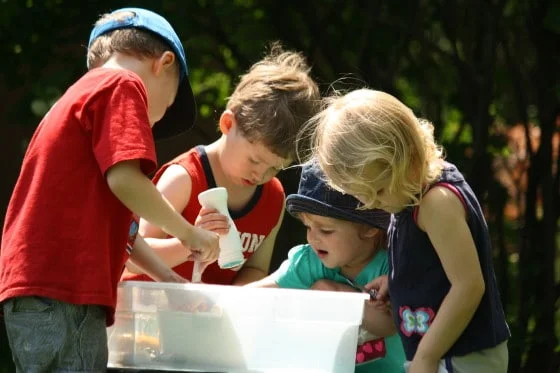
Through this hands-on activity, students can gain a deeper understanding of the science behind de-icing and how different substances can influence the physical properties of water.
Learn more: Melting Ice with Salt and Water
20. Barking Dog Chemistry Demonstration
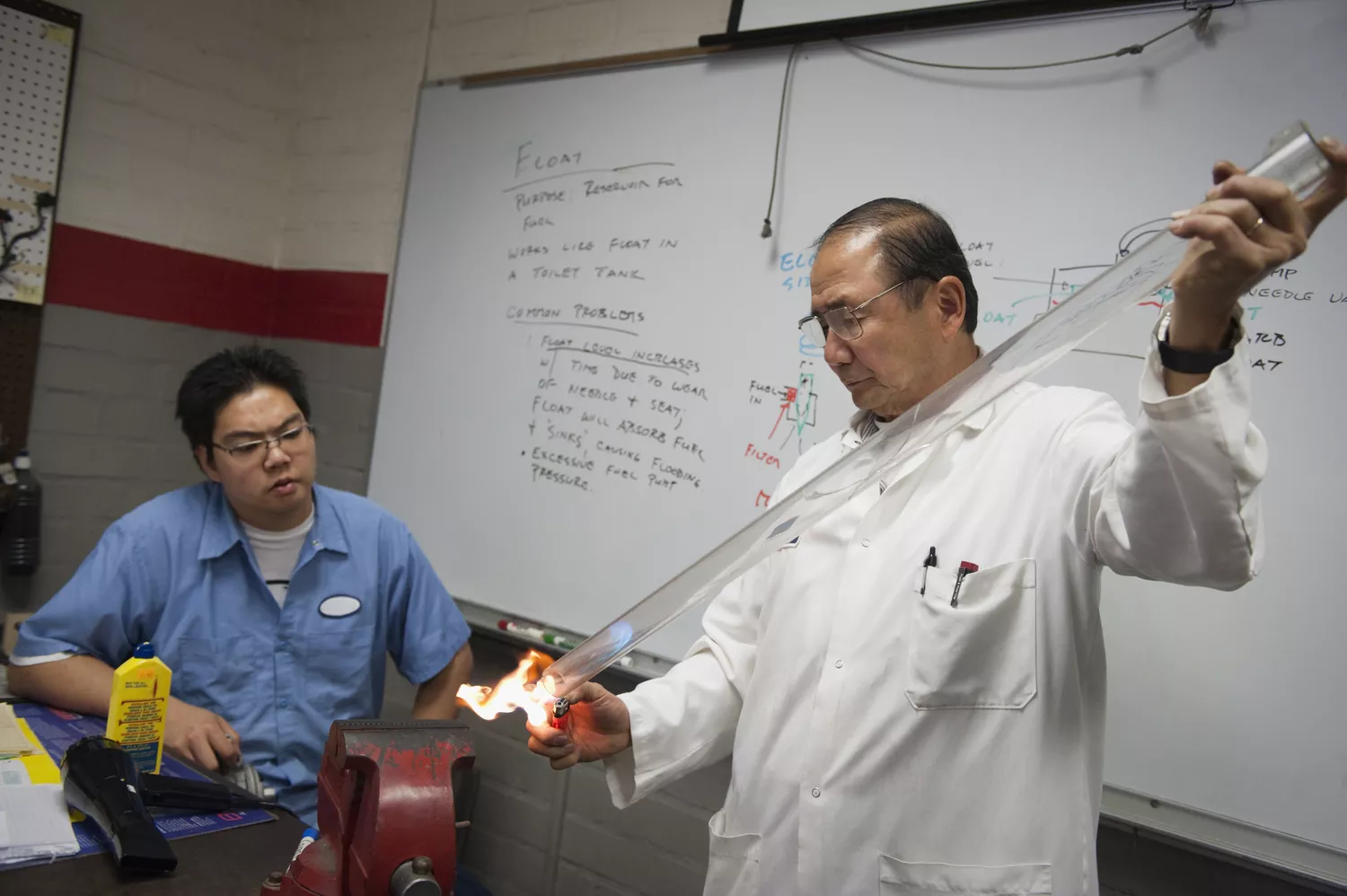
The barking dog chemistry demonstration is an exciting and visually captivating experiment that showcases the principles of combustion and gas production.
21. How to Make Egg Geodes
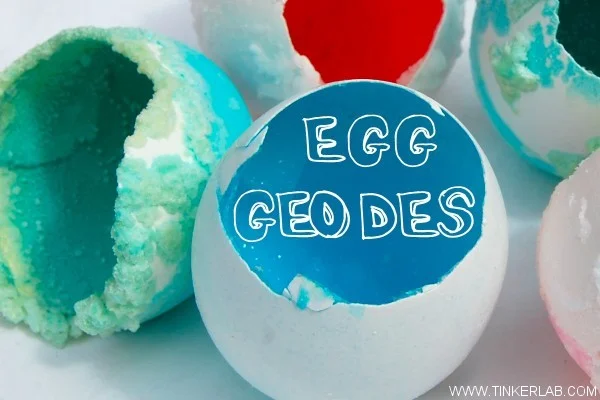
Making egg geodes is a fascinating and creative chemistry experiment that students should try. By using common materials like eggshells, salt, and food coloring, students can create their own beautiful geode-like crystals.
Learn more: How to Make Egg Geodes
22. Make Sherbet
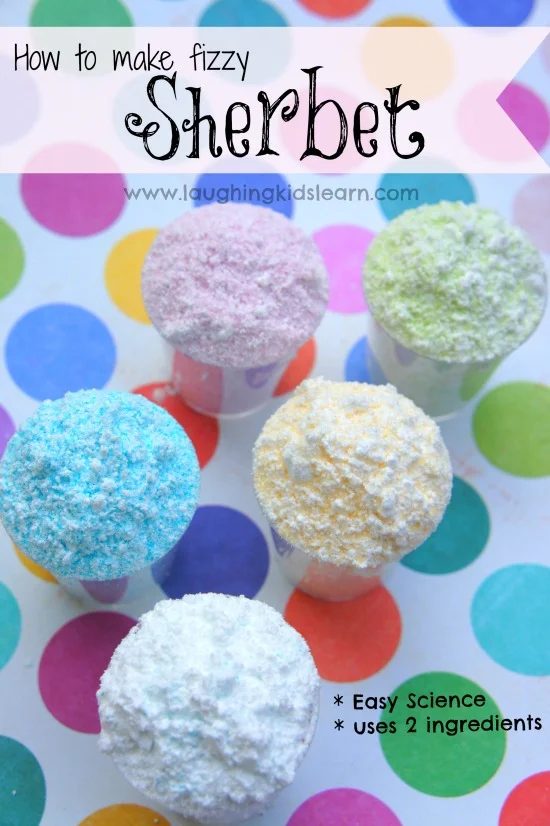
This experiment not only engages the taste buds but also introduces concepts of acidity, solubility, and the chemical reactions that occur when the sherbet comes into contact with moisture.
Learn more: Make Sherbet
23. Hatch a Baking Soda Dinosaur Egg
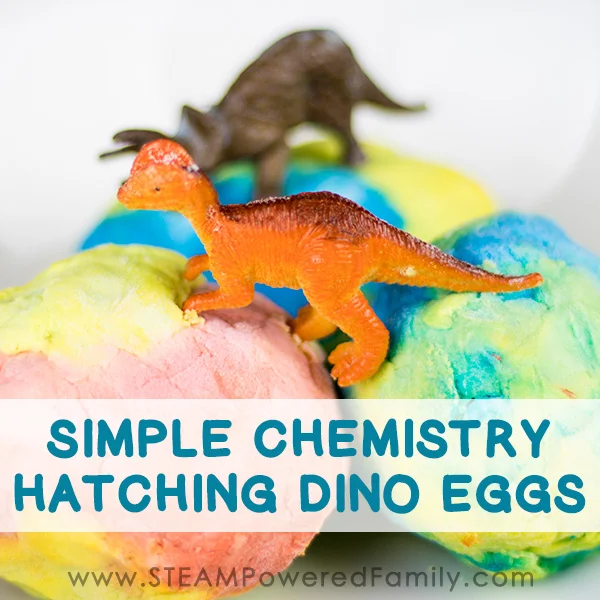
As the baking soda dries and hardens around the toy, it forms a “shell” resembling a dinosaur egg. To hatch the egg, students can pour vinegar onto the shell, causing a chemical reaction that produces carbon dioxide gas.
Learn more: Steam Powered Family
24. Chromatography Flowers
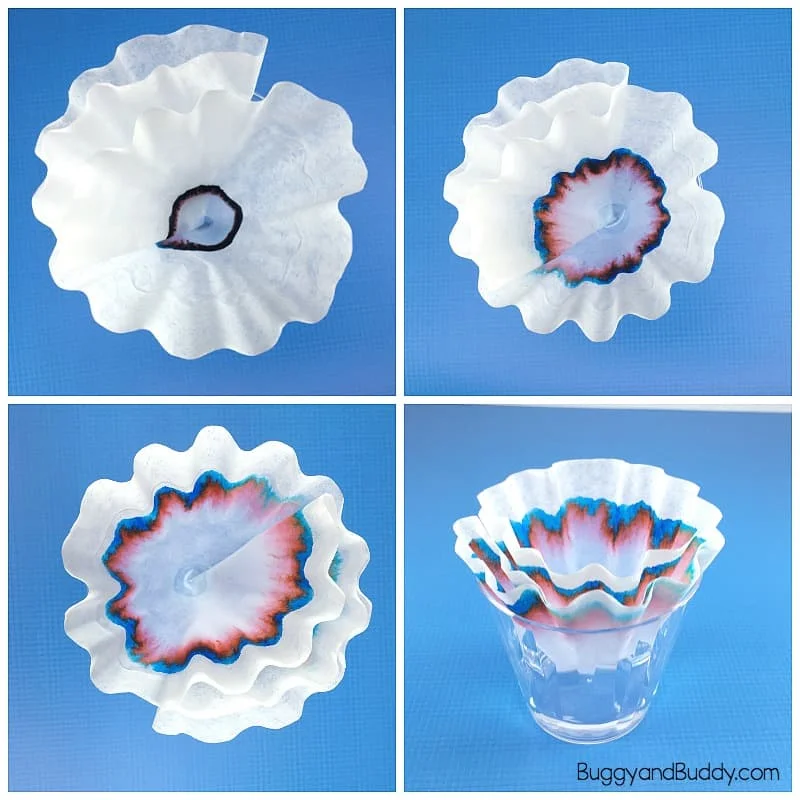
By analyzing the resulting patterns, students can gain insights into the different pigments present in flowers and the science behind their colors.
Learn more: Chromatography Flowers
25. Turn Juice Into Solid
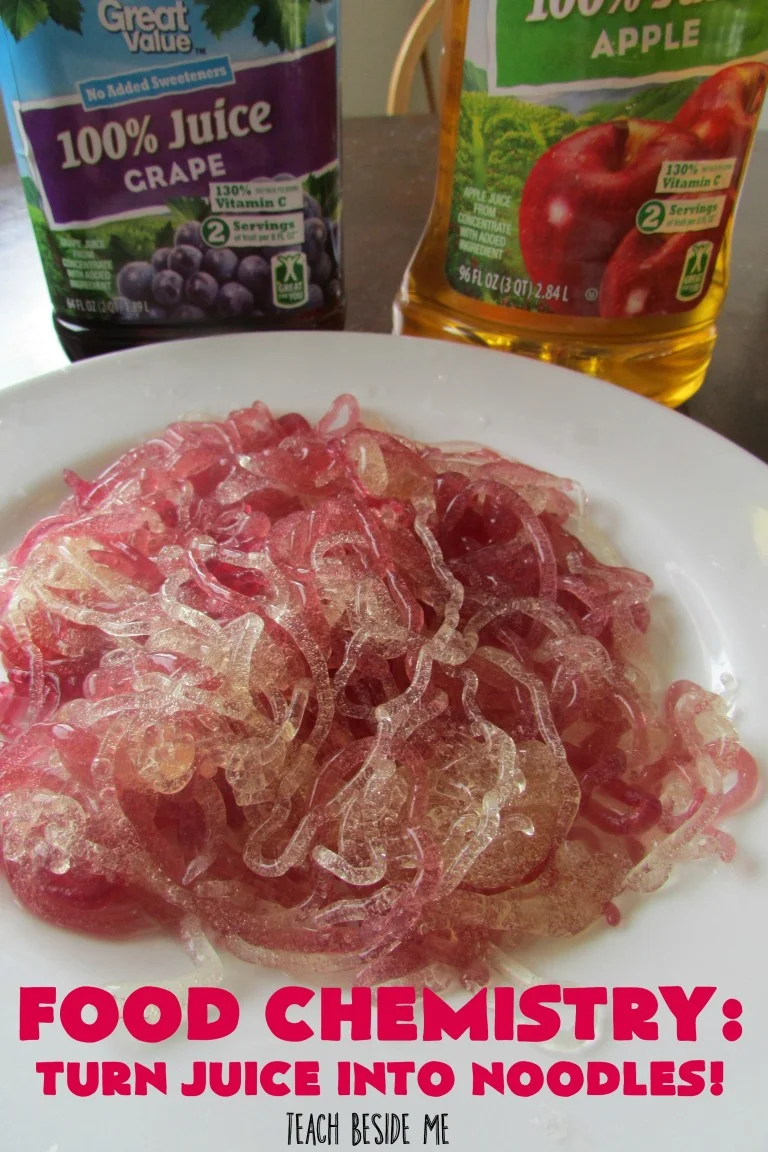
Turning juice into a solid through gelification is an engaging and educational chemistry experiment that students should try. By exploring the transformation of a liquid into a solid, students can gain insights of chemical reactions and molecular interactions.
Learn more: Turn Juice into Solid
26. Bouncy Balls
Making bouncy balls allows students to explore the fascinating properties of polymers, such as their ability to stretch and rebound.
27. Make a Lemon Battery
Creating a lemon battery is a captivating and hands-on experiment that allows students to explore the fundamentals of electricity and chemical reactions.
28. Mentos and Soda Project
The Mentos and soda project is a thrilling and explosive experiment that students should try. By dropping Mentos candies into a bottle of carbonated soda, an exciting eruption occurs.
29. Alkali Metal in Water
The reaction of alkali metals with water is a fascinating and visually captivating chemistry demonstration.
30. Rainbow Flame
The rainbow flame experiment is a captivating and visually stunning chemistry demonstration that students should explore.
31. Sugar Yeast Experiment
This experiment not only introduces students to the concept of fermentation but also allows them to witness the effects of a living organism, yeast, on the sugar substrate.
32. The Thermite Reaction
The thermite reaction is a highly energetic and visually striking chemical reaction that students can explore with caution and under proper supervision.
This experiment showcases the principles of exothermic reactions, oxidation-reduction, and the high temperatures that can be achieved through chemical reactions.
33. Polishing Pennies
Polishing pennies is a simple and enjoyable chemistry experiment that allows students to explore the concepts of oxidation and cleaning methods.
34. Elephant Toothpaste
The elephant toothpaste experiment is a thrilling and visually captivating chemistry demonstration that students should try with caution and under the guidance of a knowledgeable instructor.
35. Magic Potion
Creating a magic potion is an exciting and imaginative activity that allows students to explore their creativity while learning about the principles of chemistry.

36. Color Changing Acid-Base Experiment
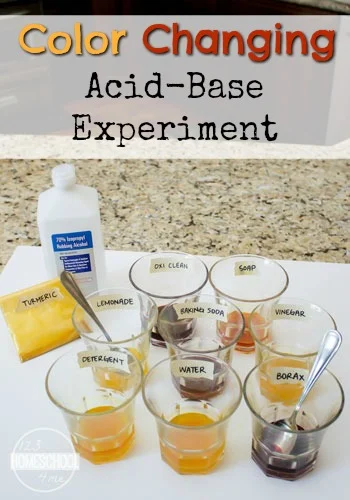
Through the color changing acid-base experiment, students can gain a deeper understanding of chemical reactions and the role of pH in our daily lives.
Learn more: Color Changing Acid-Base Experiment
37. Fill up a Balloon
Filling up a balloon is a simple and enjoyable physics experiment that demonstrates the properties of air pressure. By blowing air into a balloon, you can observe how the balloon expands and becomes inflated.
38. Jello and Vinegar
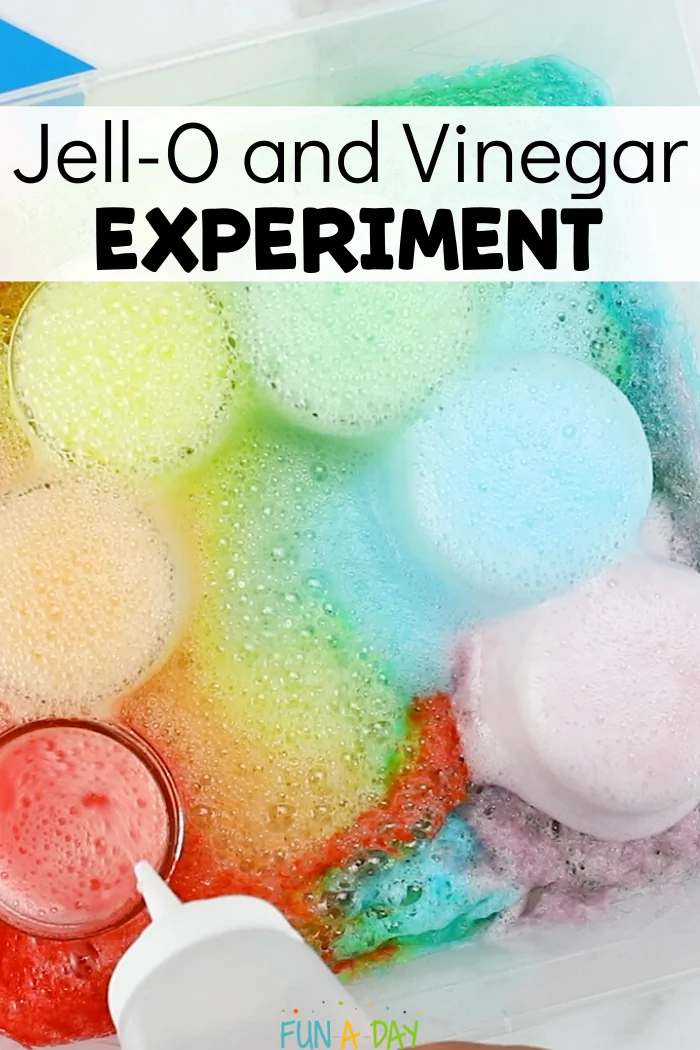
The combination of Jello and vinegar is a fascinating and tasty chemistry experiment that demonstrates the effects of acid on a gelatin-based substance.
Learn more: Jello and Vinegar
39. Vinegar and Steel Wool Reaction
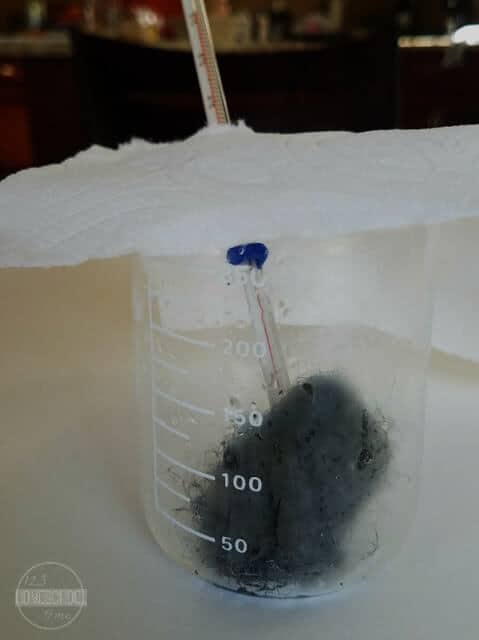
This experiment not only provides a visual demonstration of the oxidation process but also introduces students to the concept of corrosion and the role of acids in accelerating the process.
Learn more: Vinegar and Steel Wool Reaction
40. Dancing Rice
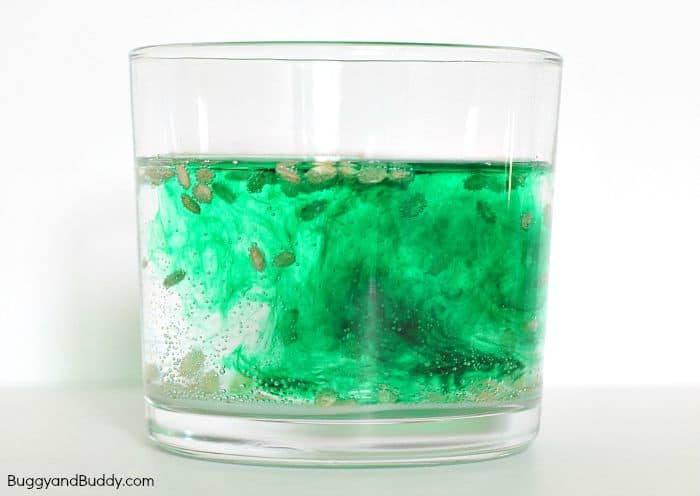
The dancing rice experiment is a captivating and educational demonstration that showcases the principles of density and buoyancy.
By pouring a small amount of uncooked rice into a clear container filled with water, students can witness the rice grains moving and “dancing” in the water.
Learn more: Dancing Rice
41. Soil Testing Garden Science
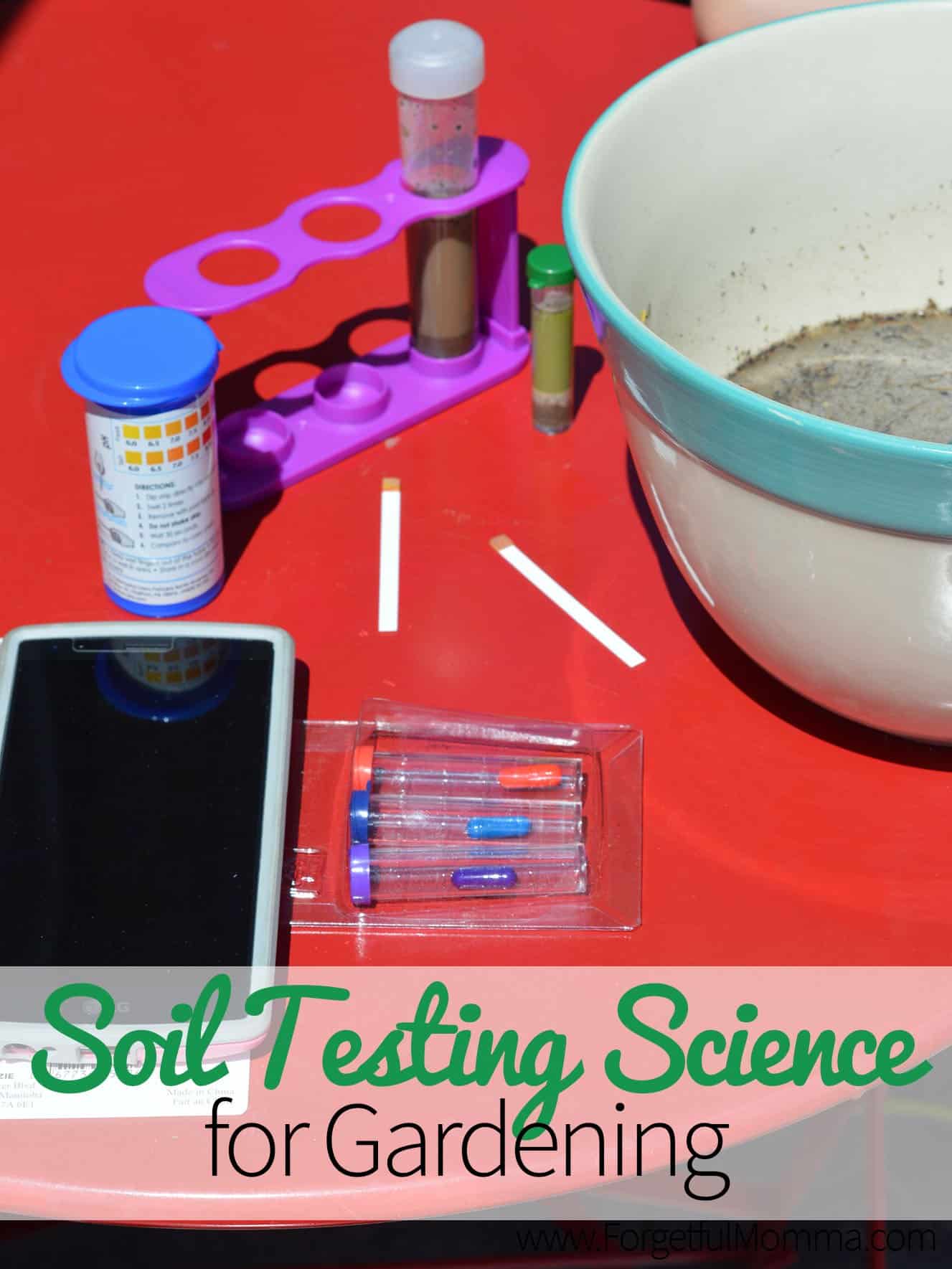
Soil testing is a valuable and informative experiment that allows students to assess the composition and properties of soil.
By collecting soil samples from different locations and analyzing them, students can gain insights into the nutrient content, pH level, and texture of the soil.
Learn more: Soil Testing Garden Science
42. Heat Sensitive Color Changing Slime
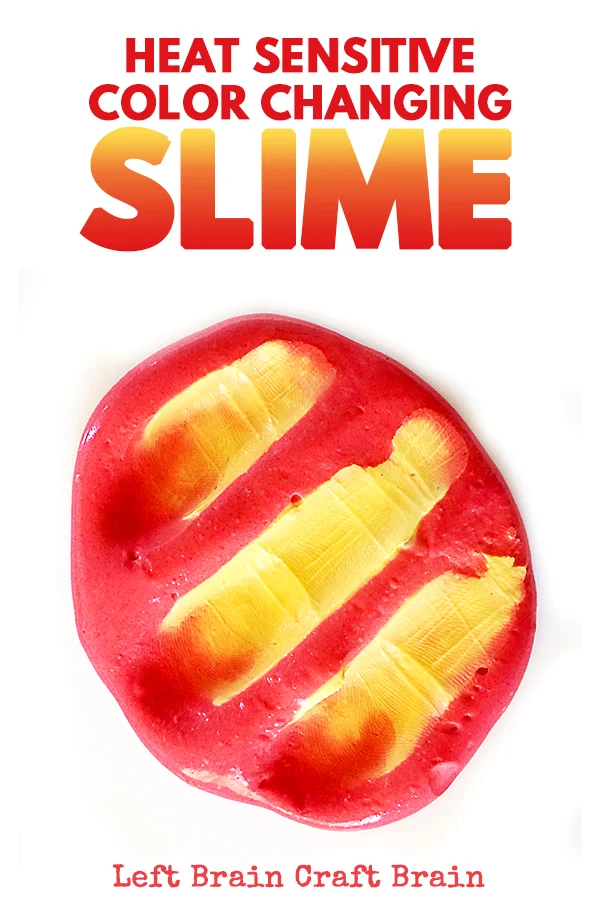
Creating heat-sensitive color-changing slime is a captivating and playful chemistry experiment that students should try.
Learn more: Left Brain Craft Brain
43. Experimenting with Viscosity
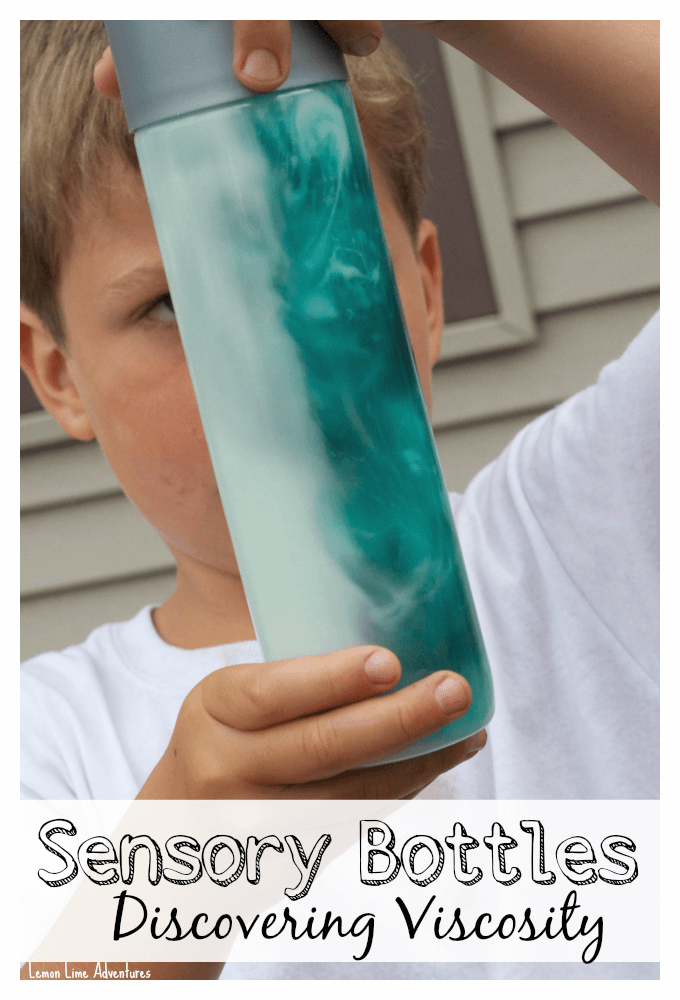
Experimenting with viscosity is an engaging and hands-on activity that allows students to explore the flow properties of liquids.
Viscosity refers to a liquid’s resistance to flow, and this experiment enables students to investigate how different factors affect viscosity.
Learn more: Experimenting with Viscosity
44. Rock Candy Science
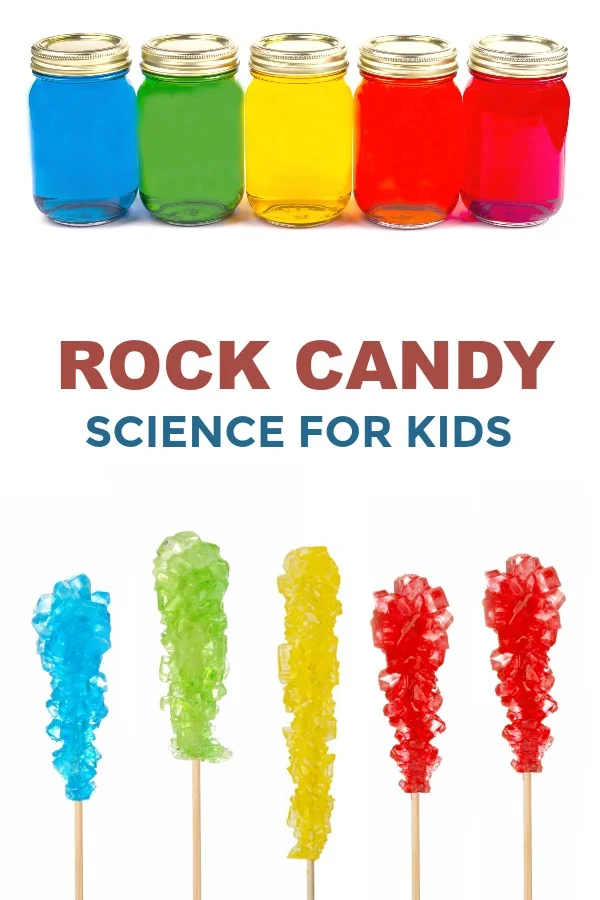
Rock candy science is a delightful and educational chemistry experiment that students should try. By growing their own rock candy crystals, students can learn about crystal formation and explore the principles of solubility and saturation.
Learn more: Rock Candy Science
45. Baking Soda vs Baking Powder
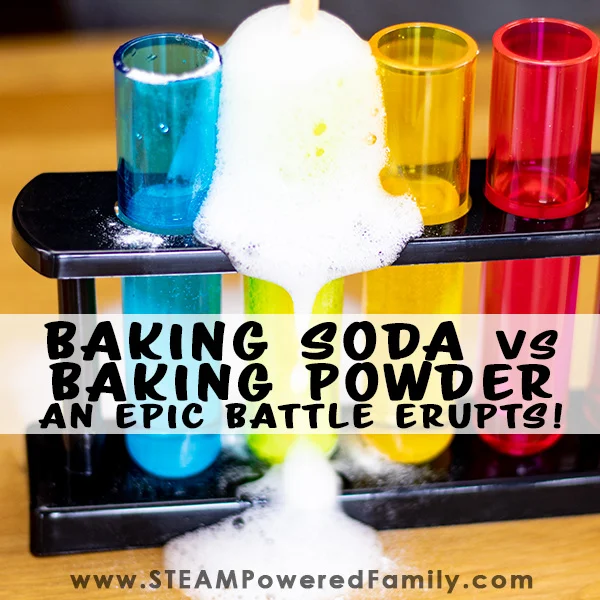
Baking soda and baking powder have distinct properties that influence the leavening process in different ways.
This hands-on experiment provides a practical understanding of how these ingredients interact with acids and moisture to create carbon dioxide gas.
46. Endothermic and Exothermic Reactions Experiment
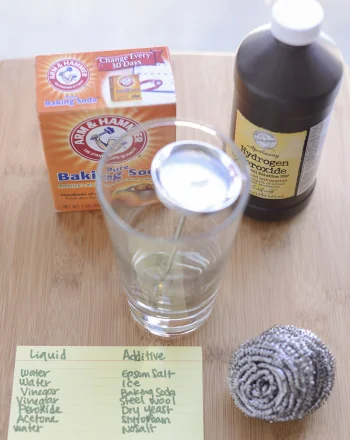
The endothermic and exothermic reactions experiment is an exciting and informative chemistry exploration that students should try.
By observing and comparing the heat changes in different reactions, students can gain a deeper understanding of energy transfer and the concepts of endothermic and exothermic processes.
Learn more: Education.com
47. Diaper Chemistry
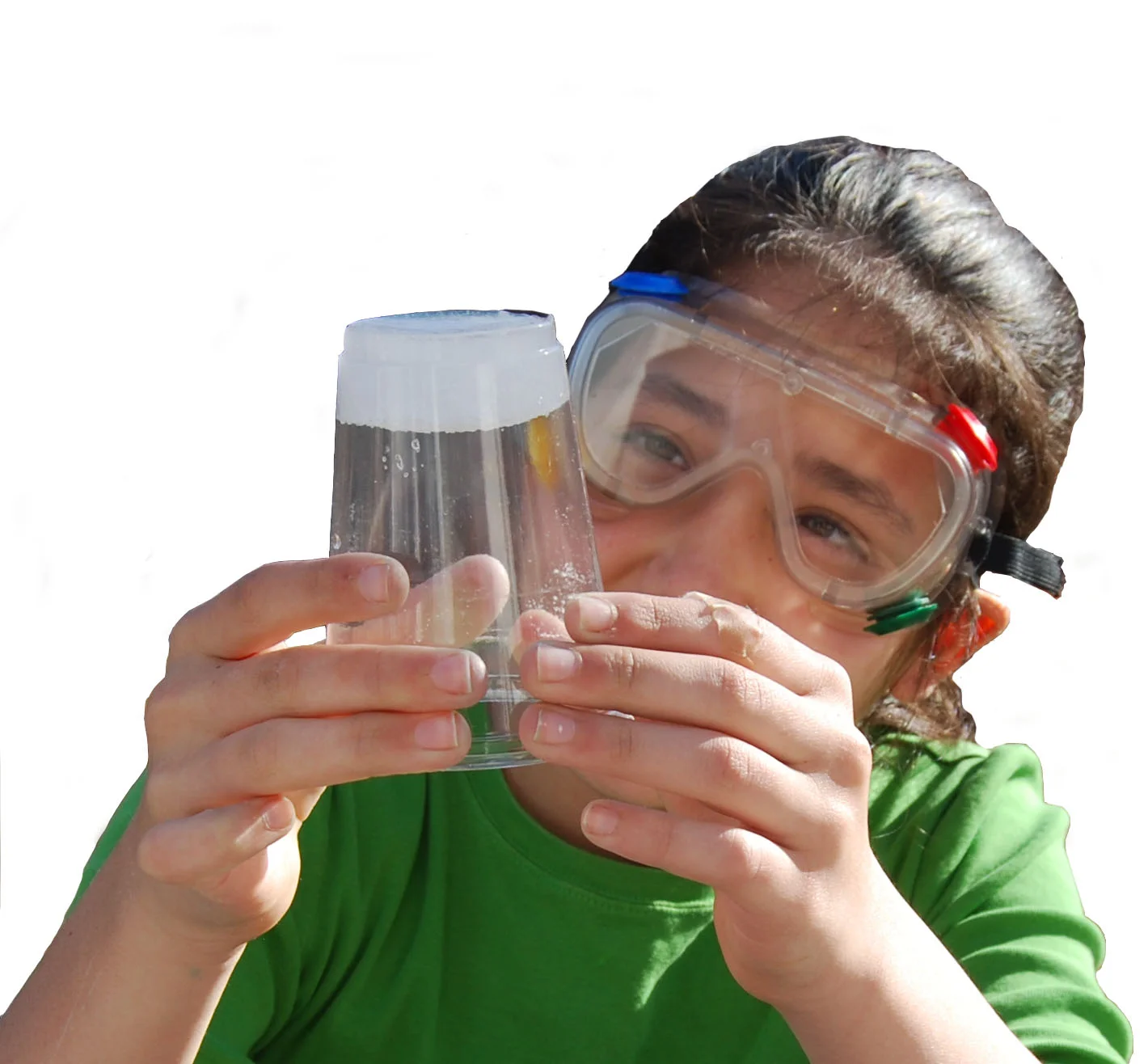
By dissecting a diaper and examining its components, students can uncover the chemical processes that make diapers so effective at absorbing and retaining liquids.
Learn more: Diaper Chemistry
48. Candle Chemical Reaction
The “Flame out” experiment is an intriguing and educational chemistry demonstration that students should try. By exploring the effects of a chemical reaction on a burning candle, students can witness the captivating moment when the flame is extinguished.
49. Make Curds and Whey
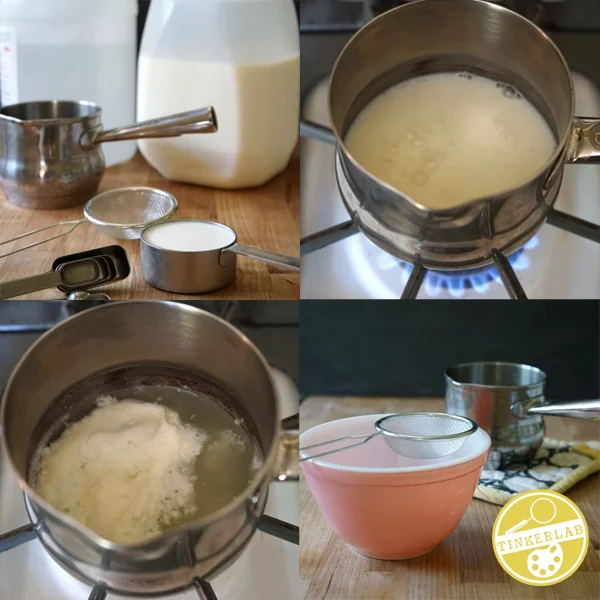
This experiment not only introduces students to the concept of acid-base reactions but also offers an opportunity to explore the science behind cheese-making.
Learn more: Tinkerlab
50. Grow Crystals Overnight
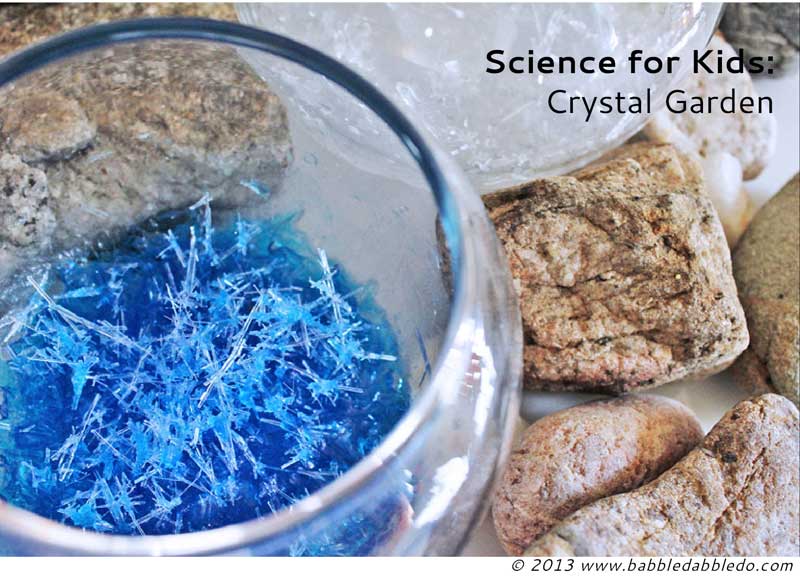
By creating a supersaturated solution using substances like epsom salt, sugar, or borax, students can observe the fascinating process of crystal growth. This experiment allows students to explore the principles of solubility, saturation, and nucleation.
Learn more: Grow Crystals Overnight
51. Measure Electrolytes in Sports Drinks
The “Measure Electrolytes in Sports Drinks” experiment is an informative and practical chemistry activity that students should try.
By using simple tools like a multimeter or conductivity probe, students can measure the electrical conductivity of different sports drinks to determine their electrolyte content.
52. Oxygen and Fire Experiment
The oxygen and fire experiment is a captivating and educational chemistry demonstration that students should try. By observing the effects of oxygen on a controlled fire, students can witness the essential role of oxygen in supporting combustion.
53. Electrolysis Of Water
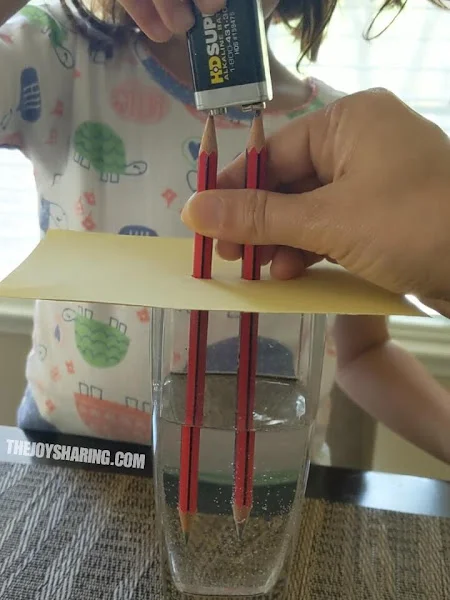
The electrolysis of water experiment is a captivating and educational chemistry demonstration that students should try.
Learn more: Electrolysis Of Water
54. Expanding Ivory Soap
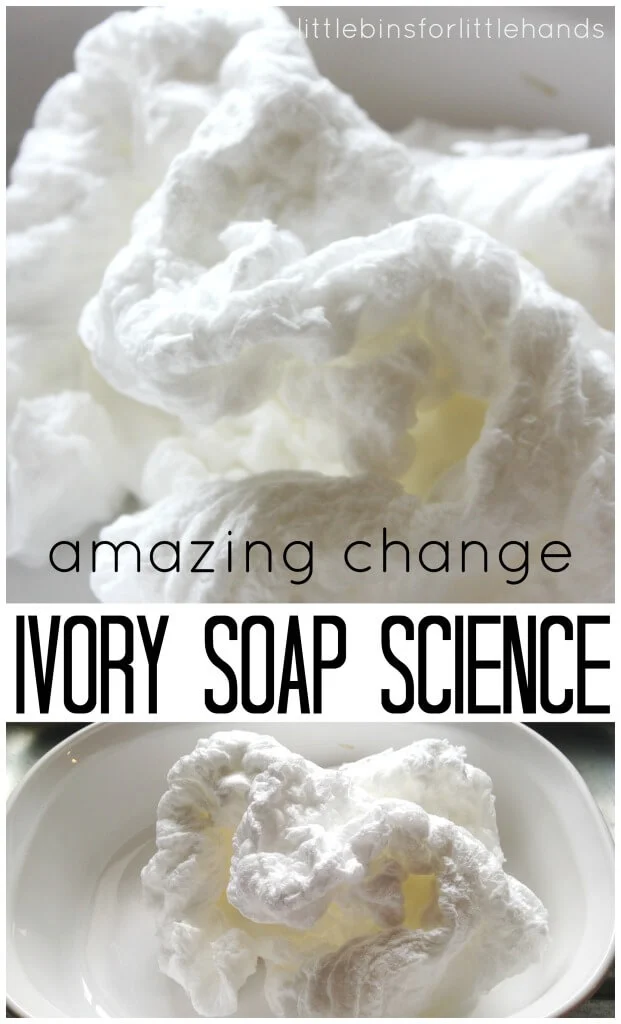
The expanding Ivory Soap experiment is a fun and interactive chemistry activity that students should try. By placing a bar of Ivory soap in a microwave, students can witness the remarkable expansion of the soap as it heats up.
Learn more: Little Bins Little Hands
55. Glowing Fireworks
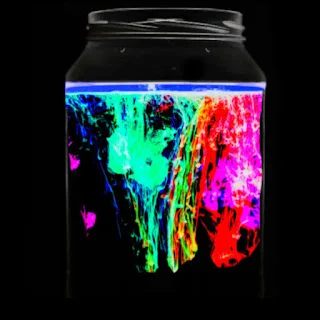
This experiment not only introduces students to the principles of pyrotechnics and combustion but also encourages observation, critical thinking, and an appreciation for the physics and chemistry behind.
Learn more: Glowing Fireworks
56. Colorful Polymer Chemistry
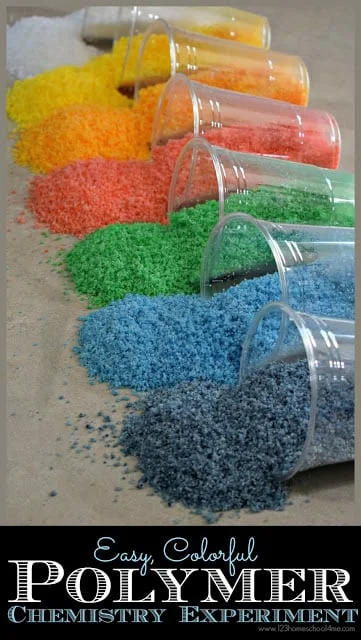
Colorful polymer chemistry is an exciting and vibrant experiment that students should try to explore polymers and colorants.
By combining different types of polymers with various colorants, such as food coloring or pigments, students can create a kaleidoscope of colors in their polymer creations.
Learn more: Colorful Polymer Chemistry
57. Sulfur Hexafluoride- Deep Voice Gas
This experiment provides a firsthand experience of how the density and composition of gases can influence sound transmission.
It encourages scientific curiosity, observation, and a sense of wonder as students witness the surprising transformation of their voices.
58. Liquid Nitrogen Ice Cream
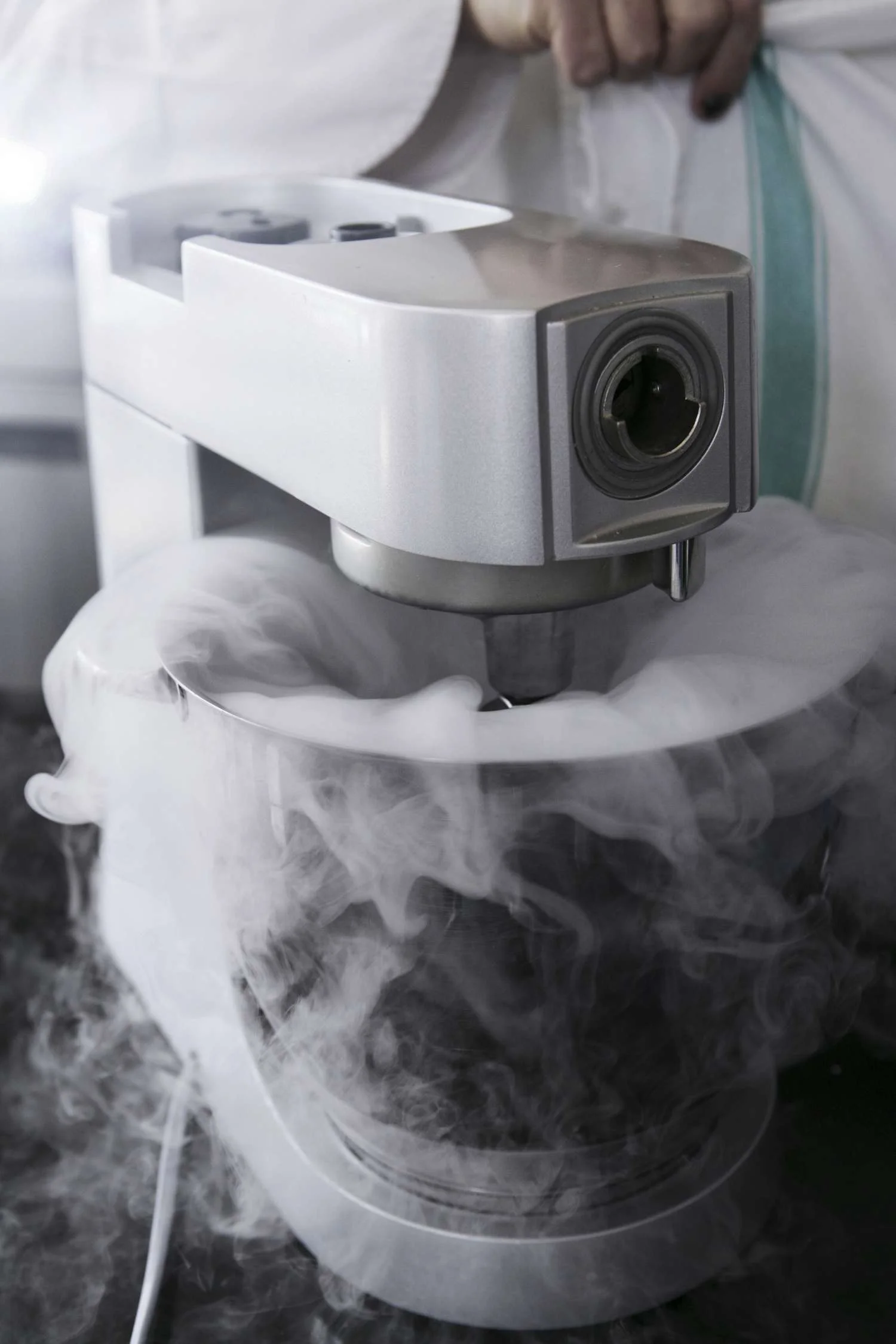
Liquid nitrogen ice cream is a thrilling and delicious chemistry experiment that students should try. By combining cream, sugar, and flavorings with liquid nitrogen, students can create ice cream with a unique and creamy texture.
59. White Smoke Chemistry Demonstration
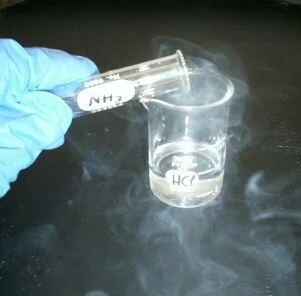
The White Smoke Chemistry Demonstration provides an engaging and visually captivating experience for students to explore chemical reactions and gases. By combining hydrochloric acid and ammonia solutions, students can witness the mesmerizing formation of white smoke.
60. Nitrogen Triiodide Chemistry Demonstration
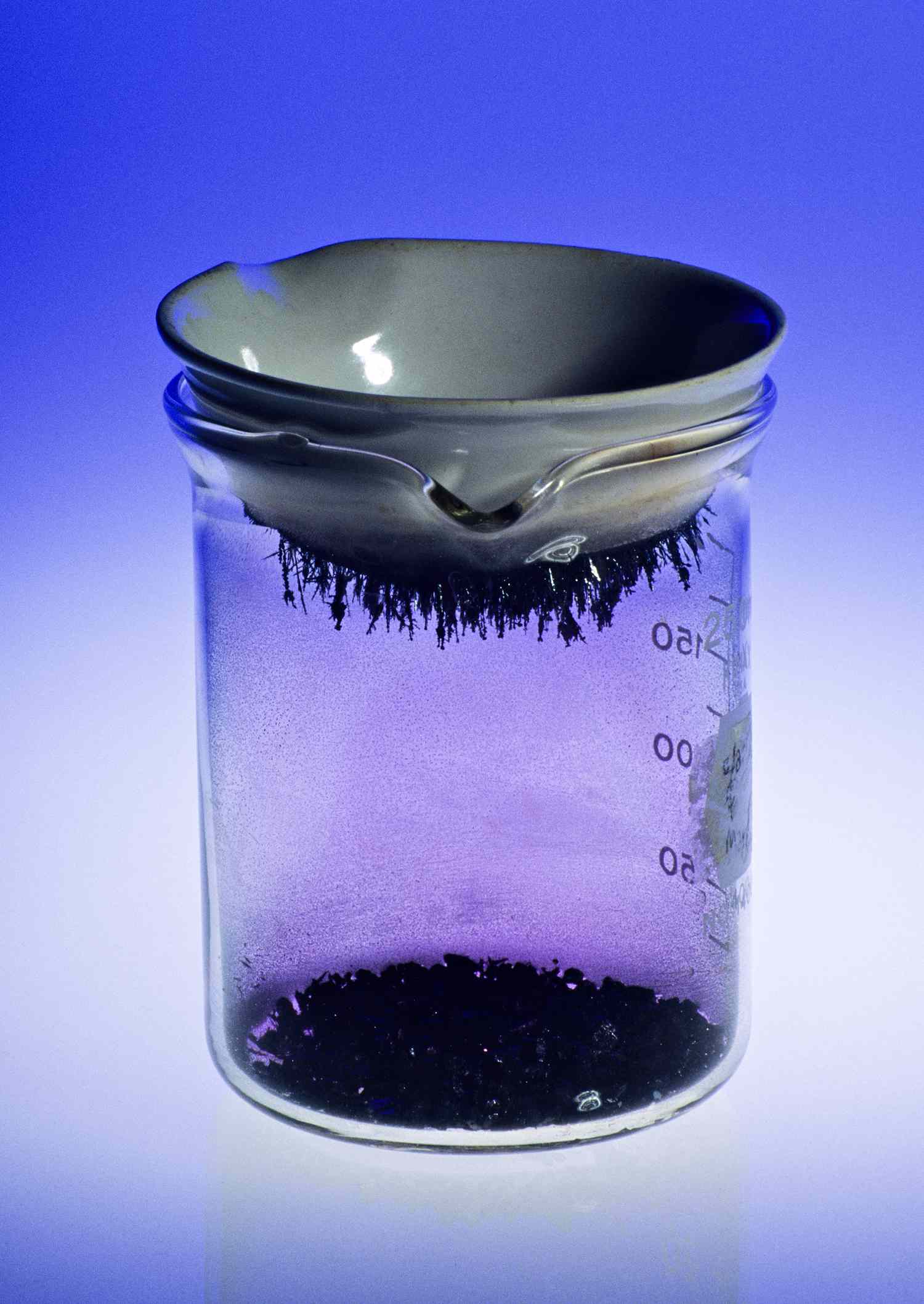
The nitrogen triiodide chemistry demonstration is a remarkable and attention-grabbing experiment that students should try under the guidance of a knowledgeable instructor.
By reacting iodine crystals with concentrated ammonia, students can precipitate nitrogen triiodide (NI3), a highly sensitive compound.
61. Make a Plastic- Milk And Vinegar Reaction Experiment
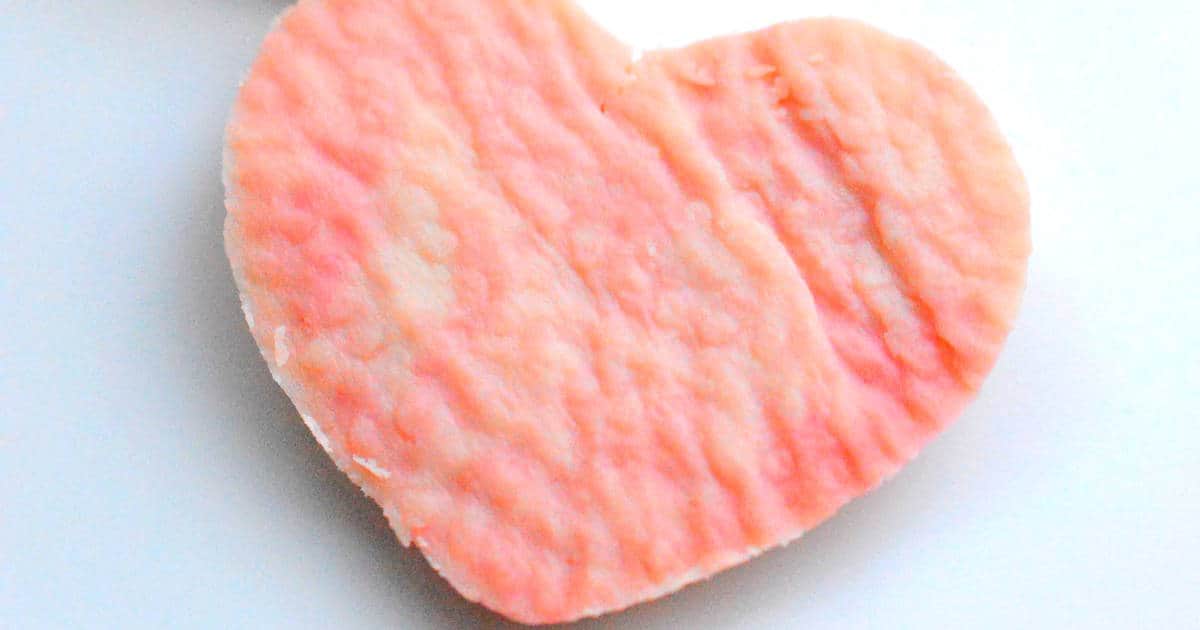
Through the “Make a Plastic – Milk and Vinegar Reaction” experiment, students can gain a deeper understanding of the chemistry behind plastics, environmental sustainability, and the potential of biodegradable materials.
Learn more: Rookie Parenting
62. Eno and Water Experiment
This experiment not only introduces students to acid-base reactions but also engages their senses as they witness the visible and audible effects of the reaction.
63. The Eternal Kettle Experiment
By filling a kettle with alcohol and igniting it, students can investigate the behavior of the alcohol flame and its sustainability.
64. Coke and Chlorine Bombs
Engaging in this experiment allows students to experience the wonders of chemistry firsthand, making it an ideal choice to ignite their curiosity and passion for scientific exploration.
65. Set your Hand on Fire
This experiment showcases the fascinating nature of combustion and the science behind fire.
By carefully following proper procedures and safety guidelines, students can witness firsthand how the sanitizer’s high alcohol content interacts with an open flame, resulting in a brief but captivating display of controlled combustion.
66. Instant Ice Experiments
The Instant Ice Experiment offers an engaging and captivating opportunity for students to explore the wonders of chemistry and phase changes.
By using simple household ingredients, students can witness the fascinating phenomenon of rapid ice formation in just a matter of seconds.
67. Coke Cans in Acid and Base
Engaging in this experiment allows students to gain a deeper understanding of the chemical properties of substances and the importance of safety protocols in scientific investigations.
68. Color Changing Invisible Ink
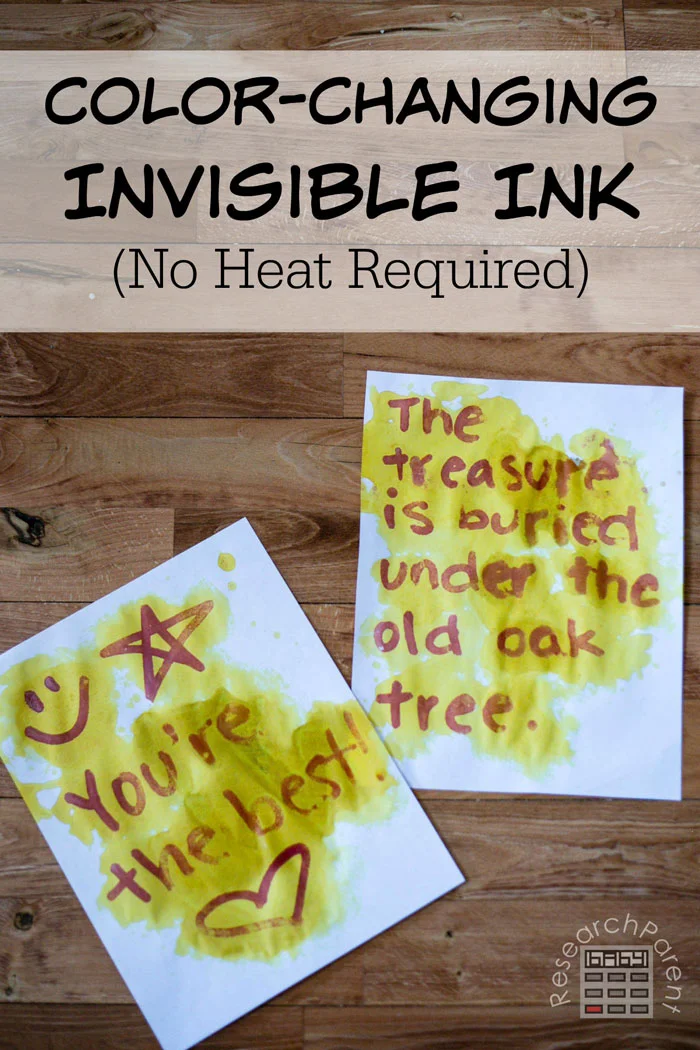
The Color Changing Invisible Ink experiment offers an intriguing and fun opportunity for students to explore chemistry and learn about the concept of chemical reactions.
Learn more: Research Parent
Similar Posts:
- Top 100 Fine Motor Skills Activities for Toddlers and Preschoolers
- 37 Water Science Experiments: Fun & Easy
- Top 40 Fun LEGO Science Experiments
Leave a Comment Cancel reply
Save my name and email in this browser for the next time I comment.
- Architecture and Design
- Asian and Pacific Studies
- Business and Economics
- Classical and Ancient Near Eastern Studies
- Computer Sciences
- Cultural Studies
- Engineering
- General Interest
- Geosciences
- Industrial Chemistry
- Islamic and Middle Eastern Studies
- Jewish Studies
- Library and Information Science, Book Studies
- Life Sciences
- Linguistics and Semiotics
- Literary Studies
- Materials Sciences
- Mathematics
- Social Sciences
- Sports and Recreation
- Theology and Religion
- Publish your article
- The role of authors
- Promoting your article
- Abstracting & indexing
- Publishing Ethics
- Why publish with De Gruyter
- How to publish with De Gruyter
- Our book series
- Our subject areas
- Your digital product at De Gruyter
- Contribute to our reference works
- Product information
- Tools & resources
- Product Information
- Promotional Materials
- Orders and Inquiries
- FAQ for Library Suppliers and Book Sellers
- Repository Policy
- Free access policy
- Open Access agreements
- Database portals
- For Authors
- Customer service
- People + Culture
- Journal Management
- How to join us
- Working at De Gruyter
- Mission & Vision
- De Gruyter Foundation
- De Gruyter Ebound
- Our Responsibility
- Partner publishers

Your purchase has been completed. Your documents are now available to view.
Concept of chemical reaction in chemistry textbooks
For more than 20 years the science teachers, the researchers in science education, the educationists and the other pedagogues have been acquainted with the model of educational reconstruction. In this model there are integrated three areas of this scientific interest: the recognition of pupils’ conceptions, the investigation of scientific conceptions with didactics branch approach and the creation of an educational content (Duit, R., Gropengießer, H., & Kattmann, U. (2005). Towards science education research that is relevant for improving practice: The model of educational reconstruction. In H. E. Fischer, (Ed.), The Developing standards in research on science education (pp. 1–9). London: Taylor & Francis; Duit, R., Gropengießer, H., Kattmann, U., Komorek, M., & Parchmann, I. (2012). The model of educational reconstruction – a framework for improving teaching and learning science. In D. Jorde & J. Dillon (Eds.), Science education research and practice in Europe. Cultural perpectives in science education, 5 (pp. 13–37). Rotterdam: Sense Publishers; Kattmann, U., Duit, R., Gropengießer, H., & Komorek, M. (1997). Das Modell der Didaktischen Rekonstruktion – Ein Rahmen für naturwissenschaftsdidaktische Forschung und Entwicklung.Zeitschrift für Didaktik der Naturwissenschaften, 3(3), 3–18). There is required to reconstruct the educational content for learning and teaching purposes. It leads to the educational structuring and to the clarification in scientific field. Therefore, in this contribution there is analysed a formation of the concept chemical reaction from the perspectives of the chemistry textbooks used in the 19 th , the 20 th and the 21 st centuries in Slovakia. These findings could be useful in the process of comparison between scientific and pedagogical knowledge in this branch not only in neighbouring countries, even in the broaden European or worldwide content.
Introduction
For last 20 years, many educational researchers have been focused on educational reconstruction ( Jelemenská, Sander, & Kattmann, 2003 ) by analyzing and re-evaluation of the science teaching content at primary and secondary schools in Slovakia and even more in other central European countries. It is connected with a researchers’ effort to the complete understanding of the individuals’ cognitive ontogenesis and also to the acquintation of phylogeny of particular concepts. The simplification and the reduction of the scientific concepts explanation ( Knecht, 2007 ) do not mean automatically the holding of the concept nature and its depth but often it leads to the misunderstandings and misapplications in practice. Therefore, there is reasonable the thoroughly approach to the making the content available to pupils and students. It is important to find out their current content knowledge as well as the cognition of historical background and the basis of science key concepts. The educational reconstruction ( Jelemenská et al., 2003 ; Kattmann, Duit, & Gropengießer, 1998 ) is one of the contemporary approaches, which is connected with mediation of the scientific content to pupils. This approach was presented for the first time in 1997 by professor Kattmann. Its importance is connected with the fact, that it does not only include the selection of a suitable scientific content and, subsequently, its mediation to pupils, but it focuses mainly on the systematic identification of pupils’ assumptions and conceptions about the scientific knowledge available to them ( Knecht, 2007 ). Pupils’ conceptions as the subjective mental constructions those come from their everyday experience are not understood as the obstacles or the barriers, but as the useful learning tools ( Jelemenská, 2009 ). The findings are compared or confronted with the knowledge that was relevant in the past and which is up-to-date nowadays. The result of this approach is then the reconstruction of educational content taking into account the objectives of the educational process, pupils’ ideas and scientific knowledge ( Knecht, 2007 ). The subject of teaching is not knowledge given by scientific field and which can be pedagogically shaped into a complex form. The purpose of the educational reconstruction is to connect scientific knowledge with interdisciplinary knowledge and with pupils’ experience, their attitudes, their value system and their comprehension. The educationally reconstructed school subject represents more complex educational content, not only simplified or didactically reduced one. It proceeds in three steps: summarization, explication, structuring. The educational reconstruction includes a supporting of meaningful learning. The mission is to inquire and to discover important relation and bonds between scientific knowledge and learning conditions of individuals. There are influenced by their motivation, social conditions and cognitive level ( Kattmann, 2009 ). Therefore, there should be created the opportunities during the teaching scientific content, to be possible to confront and evaluate critically the results of learning process. Pupils would be able to recognize the reasons for scientific appropriate undertanding of reality ( Jelemenská, 2009 ).
Methodology
The idea of the historical way of understanding scientific concepts in different scientific field is one of the principal postulates of the educational reconstruction concept. Such a historical excursus shall help in the prediction of probable mental processes, obstacles, difficulties and manners how to overcome a priori conceptions in the way of deeper science concepts understanding. A certain analogy between the scientific knowledge phylogeny and individual knowledge ontogenesis is presumed. From our point of view, the historical excursus is needed because of the progress of educational concepts during the knowledge development in educational system. It is important especially for teachers, who are preparing their own models of forming concept in pupils’ minds. According to the historical changes of forming the particular concepts in teaching subject the teacher better see and understand the requirements of scholar practice and his own approach is then more inventive. That was the reason we decided to study the content of chemistry textbooks which were used in Slovakia in the past and which are used today. We were focused on the phenomenon of chemical reaction in our searching. We were monitoring several approaches to the understanding of the concept of chemical reaction. The textbook content analysis was used as a research method. The way of making the construct understandable according to the pupil’s age, the type of school he/she attends was the aim of research in analysis of the accessible chemistry textbooks (and their historical equivalents). The historical aspect of concept chemical reaction is presented in more than 100 years period including its composition into other basic chemical knowledge, into the source concepts and associated concepts ( Prokša, 2017 ). We compared the chemical content in textbooks from the 2 nd half of 19 th century with the explanation of the concept of chemical reaction 100 years later. Nowaday understanding presented in textbooks is offered too. The content analysis of these selected textbooks will show how the knowledge has evolved over time.
Analysis and results
The key concepts and the connotations to the concept of chemical reaction in the analysed chemistry textbooks from the 2 nd half of the 19 th century.
The key concepts and the connotations to the concept chemical reaction in the analysed chemistry textbooks from the 2 nd half of the 20 th century.
The key concepts and the connotations to the concept of chemical reaction in the analysed chemistry textbooks using today.
The definition of chemical reaction was shaped in the past and was forming to the contemporary version what is written down in the Figure 1 .

The genesis of the definitions to the concept of chemical reaction in chemistry textbooks since 1869.
Nowadays there is generally accepted and in chemical sources presented the definition of the chemical reaction: Chemical reaction is a change in which one or more chemical elements or compounds (the reactants) form new compounds (the products). All reactions are to some extent reversible, the products can also react to give the original reactants. However, in many cases the extent of this back reaction is negligibly small, and the reaction is regarded as irreversible ( Daintith, 2008 ). There were appeared a lot of definitions of the concept of Chemical reaction in textbooks. In the currently used textbook of chemistry is chemical reaction considered as a process during which the substances are changing: new chemical substances are forming from some chemical substances. The subtances which are getting in and are reacting together are called reactants and substances which are a result of chemical reaction are called products. Therefore, during the chemical reaction the reactants are changing into products. The chemical reactions are written in the way of the chemical schemes – the chemical equations. The simple one is written with one reaction scheme and more complex are written with set of equations ( Vicenová & Ganajová, 2017 ).
This specification is the result of analysis of the historical sources, of the phylogeny of key concept in connection with the scientific knowledge of that times and their reflection in the textbooks. Only this process could lead to precise the concept into contemporary perception and acceptation by the professionals and scientific experts. Several simple information and facts about the chemical reactions of chosen substances are in the textboks for pupils of lower secondary education. The content proceeds from the concepts the compound, the element, the chemical combination, the chemical decomposition to help to define the concept of chemical reaction. It is very important to point out the condition of the forming, of the regulation of progress and of practical impact for everyday life. Then it is possible to explain the different kinds of the chemical reaction from many points of view ( Dillinger, 1977 ).
Conclusions
The presented analysis of forming the concept of chemical reaction in Slovak pupils’ conceptions in the diapason of past more than 100 years could help teacher to find a focus of his own model. The comparison of the object content in Slovak textbooks shows the reason of existence of two absolute different accesses to the depth and to the width of the curriculum related to the chemical reaction in the borders of more than 100 years lasting history. The conception from the 19 th century is meaningful nowadays itself, because the need of teaching chemistry in connection of the everyday life is reasonable. There are not only expectant scientists and chemists, but most of the pupils will be in their future “the practical chemists”, who will use their knowledge in the kitchen, in the bathroom etc. It is necessary for the school practice to handle safely the “traps of chemical phenomena” that surround us. On the other hand, we also need to prepare future scientists, who will make headway in the human knowledge in that research field. That’s why, the conception of the didactical scientism from the second half of 19 th century has its application in modified and limited version.
According to the possibilities and the limits of the achievement of time period the textbooks also has been a part of process of developing. In the first half of the 20 th century, the information were simplified, with black-white pictures (especially of chemical technologies) and schemes. Nowadays, many different visual elements are used, not only in the macroscopic level, but also in the submicroscopic and microscopic levels. Several textbooks contain the e-version on DVD with the visualisations and the simulations of the chemical reactions. The complexity of information has been gradually arising and according to the didactic reconstruction of teaching science it should be carefully chosen and selected in respect of pupils’ and students’ individual mental development and also the recent chemistry knowledge. We found out that in the oldier chemistry textbooks only the simple definition of chemical reaction was published with information about existing exo- and endothermic reactions. The chemical and physical processes were explained separately in chemistry and in physics. Nowadays, they are clearly differentiated also in topic of the chemical reaction. The chemical reaction content deepen gradually including the knowledge of termochemistry, chemical reaction rate and chemical equilibrium. Pupils are able to understand the chemical reactions as the changes linked with the moving and interaction of valence electrons, connected with forming and destroying of the chemical bonds after learning about the chemical bonds and the electron theory ( Dillinger, 1977 ). In the newer chemistry textbooks the aspects of chemical reaction rate were added to this field of research. There were mentioned the factors, which influence the rate of chemical reactions firstly without the principle how they works, later with the explanation which factor and how it affects the chemical reaction rate. Nowadays, the processes and the principles are better clearing up. The writting chemical reaction was firstly only verbal (as it is in the first acquiatance with the concept in the 7 th grade pupils), later with the simply schemes, then there appeared symbolic scripts with punctuated arrows to recent written ways of chemical reactions. The chemical equation is a record of what happens in the chemical reaction ( Green & Danji, 2001 ).
While analyzing the textbooks we found out, that not only in the older textbooks even in the new ones it is possible to read the claims and defintions that could be eventually the sources of key concept misconception of pupils. We should be carefull and critical to the information in textbooks too, because of possible misconceptons that will appear, if the information is not very well presented and understood. According to the textbook content analysis we found out that not only in the older textbooks the claim such as: While breathing we breath an oxygen in and a nitrogen returns back not used or the air consists of an oxygen (which is maintaining a fire) a nitrogen and a carbonic acid (what are not keeping a fire) appeared ( Kožehuba, 1872 ), but in nowadays textbooks for lower secondary education the claim: The chemical reaction rate is appointed by the time needed for changing of the reactants into products ( Vicenová & Ganajová, 2017 ) is mentioned, what is incorrect according to the physical units use for rate and of time. The several concepts appeared or got lost from the basic content, for example in the textbook from 2017 the concept of exothermic and endothermic reaction is mentioned, but several years earlier (2011) was not used. The concept of activation energy existed in the textbook published in 2011 and caused misunderstanding of this concept for several years in chemistry teaching, but in new one textbook (published in 2018) is not found.
Taber (2001) , found out, that students may often have difficulty visualising molecular level processes and have very different mental image of the quantaction process and they thought that the reaction occurs so that the atoms present can get full shells. Many students do not differ between a chemical change and a change of state, which chemists call a “physical change” ( Barker, 2000 ). Ahtee and Varjola (1998) dissolving and change of state were chemical reactions. The endeavour of science teacher is to respect teaching principles such as scientism and appropriateness in the whole context of understanding, but not to simplify the content into the level which could cause misunderstandings. That is the reason for being interested in historical point of view and for entreching key concepts from this scientific field into the conditions of pedagogical practice.
Funding source: Vedecká Grantová Agentúra MŠVVaŠ SR a SAV
Award Identifier / Grant number: VEGA 1/0166/16
Funding statement: This research was realized with a support of grant VEGA 1/0166/16, Funder id: http://dx.doi.org/10.13039/501100006109 and UK/309/2018.
Adamkovič, E., & Šimeková, J. (2001). Chémia 9 . (Chemistry 9) (pp. 177). Bratislava: SPN. ISBN 80-08-03094-1. Search in Google Scholar
Adamkovič, E., Hofmannová, V., Pumpr, V., Šramko, T., & Tomeček, O. (1982). Chémia pre 7. ročník základnej školy. (Chemistry for the 2nd grade of lower secondary education) (pp. 176). Bratislava: SPN. Search in Google Scholar
Adamkovič, E., Beneš, P., Pumpr, V., Šramko, T., & Tomeček, O. (1990). Chémia pre 7. ročník základnej školy . (Chemistry for the 2nd grade of lower secondary education) (pp. 128). Bratislava: SPN. ISBN 80-08-00449-5. Search in Google Scholar
Adamkovič, E., Šimeková, J., & Šramko, T. (2000). Chémia 8 . (Chemistry 8) (pp. 120). Bratislava: SPN. ISBN 80-08-01380-X. Search in Google Scholar
Ahtee, M., & Varjola, I. (1998). Students’ understanding of chemical reaction. International Journal of Science Education , 20 (3), 305–316. 10.1080/0950069980200304 Search in Google Scholar
Barker, V. (2000). Beyond appearances: Students’ misconceptions about basic chemical ideas: A report prepared for the Royal Society of Chemistry (pp. 78). London: Education Division, Royal Society of Chemistry. Search in Google Scholar
Bežo, J. (1873). Silozpyt čili Fyzika pre národné školy . (Strenght teaching or Physics for national schools) (pp. 42). Senica. 2. vydanie. Search in Google Scholar
Dillinger, M., Klein, M., Hollý, Š., Horváth, S., Merva L., Ružička, I., Ružička, J., & Smik, L. (1977). Kapitoly z didaktiky chémie . (Chapters of chemistry teaching) (pp. 336). Bratislava: SPN. Search in Google Scholar
Duit, R., Gropengießer, H., & Kattmann, U. (2005). Towards science education research that is relevant for improving practice: The model of educational reconstruction. In H. E. Fischer, (Ed.), Developing standards in research on science education (pp. 1–9). London: Taylor & Francis. Search in Google Scholar
Duit, R., Gropengießer, H., Kattmann, U., Komorek, M., & Parchmann, I. (2012). The model of educational reconstruction – a framework for improving teaching and learning science. In D. Jorde & J. Dillon (Eds.), Science education research and practice in Europe. Cultural perpectives in science education, 5 (pp. 13–37). Rotterdam: Sense Publishers. 10.1007/978-94-6091-900-8_2 Search in Google Scholar
Ertl, H. (1893). Učebná kniha - Tankönyv pre slovenské každodenné a opakujúce ľudové školy . (Textbook for Slovak everyday and repeated folk schools) (pp. 144). Budapest: Singer & Wolfner. Search in Google Scholar
Greb, E., Alfred Kemper, A., & Quinzler, G. (1995). Chémia pre základné školy . transl. Daniela Joniaková Chemistry for lower school education (pp. 240). Bratislava: SPN. ISBN 80-08-02291-4. Search in Google Scholar
Green, J., & Danji, S. (2001). International baccalaureate Chemistry (second ed.) (pp. 738). Victoria: IBID Press, ISBN 1 876659 41 6. Search in Google Scholar
Györffy, J. (1899). Prírodopis dľa ministerialného rozvrhu učebného pre žiakov ľudových škôl . (The natural history according to the ministry schedule for pupils of folks schools) (9th ed.) (pp. 60). Ostrihom: Gustáv Buzárovits. Search in Google Scholar
Jelemenská, P. (2009). Model didaktické rekonstrukce z metodologického pohledu. (Model of content reconstructionfrom methodology point of view). In Janíková, M., & Vlčková, K. (Eds.), Výzkum výuky: Tematické oblasti, výzkumné přístupy a metody . (Educational research: Topics, research approaches and methods) (pp. 145–172). Brno: Paido. Search in Google Scholar
Jelemenská, P., Sander, E., & Kattmann, U. (2003). Model didaktickej rekonštrukcie: Impulz pre výskum v odborových didaktikách (Model of content knowledge: The impuls for research in technology of education). Pedagogika , 53 (2), 190–201. Search in Google Scholar
Kattmann, U. (2009). Didaktická rekonstrukce: učitelské vzdělávání a reflexe výuky (Content reconstruction: teachers´ education and teaching reflection). In Janík, T., (Ed.), Možnosti rozvíjení didaktických znalostí obsahu u budoucích učitelú. (Developing possibilities of educational content knowledge by pre-service teachers) (pp. 17–31). Brno: Paido. Search in Google Scholar
Kattmann, U., Duit, R., Gropengießer, H., & Komorek, M. (1997). Das Modell der Didaktischen Rekonstruktion – Ein Rahmen für naturwissenschaftsdidaktische Forschung und Entwicklung. Zeitschrift für Didaktik der Naturwissenschaften , 3 (3), 3–18. Search in Google Scholar
Kattmann, U., Duit, R., & Gropengießer, H. (1998). The model of educational reconstruction. Bringing together issues of scientific clarification and students’ conceptions. In Bayrhuber, H. & F. Brinkman (Eds.), What–Why–How? Research in Didaktik of Biology. Proceedings of the first conference for European researchers in Didaktik of biology (ERIDOB) (pp. 253–262). Kiel, Germany: IPN. Search in Google Scholar
Knecht, P. (2007). Didaktická transformace aneb od “didaktického zjednodušení” k “didaktické rekonstrukci”. Orbis scholae , Praha: Karolinum, 2 (1), 67–81, ISSN 1802-4637. 10.14712/23363177.2018.152 Search in Google Scholar
Kordoš, G. (1871). Stručný prírodopis pre slovenské národné školy . (Brief natural history for Slovak national schools) (pp. 45). Skalica: Fr. X. Škarnycl sons. Search in Google Scholar
Kordoš, G. (1872). Prostonárodnia Fysika, Silozpyt pre školu a dom dľa induktívnej methody . (Simple folk physics, strength teaching for school and household in inductive method) (pp. 112). Levoča. Search in Google Scholar
Kordoš, G. (1873). Stručná Fyzika čili Silozpyt pre slov. národnie školy dľa induktívnej methody . (Brief physics or strength teaching for Slovak national schools in inductive method) (pp. 57). Levoča. Search in Google Scholar
Kordoš, G. (1874). Stručná Fysika čili Silozpyt pre slovenské národnie, nedelnie a opakovacie školy dľa induktívnej methody . (Brief physics or strength teaching for Slovak national, sunday and repeted schools in inductive method) (pp. 65). Turčiansky Svätý Martin. 2. vydanie. Search in Google Scholar
Kožehuba, J. (1872). Prírodopis pre národné školy . (Natural history for national schools). Diel 3 (pp. 32). Nerastopis. Turčiansky Svätý Martin. Search in Google Scholar
Matzenauer, F. O. (1874). Krátky prírodopis pre slovenské národnie školy . (Brief natural history for Slovak national schools) (pp. 94). Banská Štiavnica. 2. vydanie. Search in Google Scholar
Daintith, J. (2008). Oxford dictionary of chemistry. (6th ed.) (pp. 592). Oxford University Press. p.118, ISBN 9780199204632. Search in Google Scholar
Prokša, M. (2017). Od chemického k didaktickému konceptu chemickej väzby. Pedagogická revue , 64 (1), 90–102. ISSN 1335-1982. Search in Google Scholar
Seidel, P. (1874). Obrázkový prírodopis pre národnie školy . (Pictorial natural history for national schools) (pp. 40). Budapest: Athenäum. Search in Google Scholar
Šramko, T., Beneš, P., Čaklóšová, Z., Klein, M., Pumpr, V., & Šimek, M. (1982). Chémia 8 . (Chemistry 8) (pp. 140). Bratislava: SPN. Search in Google Scholar
Taber, K. (2001). Building the structural concepts of chemistry: Some considerations from educational research. Chemistry Education Research and Practice , 2 (2), 123–158. 10.1039/B1RP90014E Search in Google Scholar
Taber, K. (2002). Chemical Misconceptions: Prevention, diagnosis and cure: Theoretical background (1st ed.) (pp. 190). London: Royal Society of Chemistry. ISBN 978-0-85404-386-6. Search in Google Scholar
Trtílek, J., Krsička, R., & Ondrášek, J. (1963). Chémia 8 . (Chemistry 8) (pp. 144). SPN: Praha. Search in Google Scholar
Vicenová, H. (2011). Chémia pre 8. ročník základných škôl a 3. ročník gymnázií s osemročným štúdiom. (Chemistry for the 3 rd grade of lower secondary education) (pp. 112). Bratislava: Expol Pedagogika. ISBN 978-80-8091-223-9. Search in Google Scholar
Vicenová, H. (2018). Chémia pre 8. ročník základnej školy a 3. ročník gymnázia s osemročným štúdiom (učebnica) . (Chemistry for the 3 rd grade of lower secondary education (textbook)) (pp. 96). Bratislava: Expol Pedagogika. ISBN 978-80-8091-489-9. Search in Google Scholar
Vicenová, H., & Ganajová, M. (2017). Chémia pre 7. ročník základnej školy a 2. ročník gymnázia s osemročným štúdiom. (Chemistry for the 2 nd grade of lower secondary education) (pp. 96). Bratislava: Expol Pedagogika. ISBN 978-80-8091-427-1. Search in Google Scholar
Vicenová, H., Zvončeková, V., Adamkovič, E., & Romanová, D. (2010). Chémia pre 7. ročník základnej školy a 2. ročník gymnázií s osemročným štúdiom. (Chemistry for the 2 nd grade of lower secondary education) (pp. 80). Bratislava: Expol Pedagogika. ISBN 978-80-8091-218-5. Search in Google Scholar
Zoch, I.B. (1869). Physika čili Silozpyt . (Physics or Strength-teaching) (pp. 123). Skalica: Fr. X. Škarnicl sons. Search in Google Scholar
Zoch, I.B. (1870). Physika čili Silozpyt . (Physics or Strength-teaching) (pp. 177). Skalica: Fr. X. Škarnicl sons. Search in Google Scholar
© 2019 IUPAC & De Gruyter, Berlin/Boston
This work is licensed under the Creative Commons Attribution 4.0 Public License.
- X / Twitter
Supplementary Materials
Please login or register with De Gruyter to order this product.
Journal and Issue
Articles in the same issue.
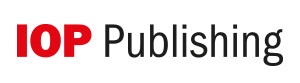
We apologize for the inconvenience...
To ensure we keep this website safe, please can you confirm you are a human by ticking the box below.
If you are unable to complete the above request please contact us using the below link, providing a screenshot of your experience.
https://ioppublishing.org/contacts/
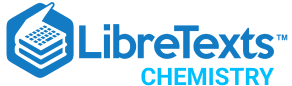
- school Campus Bookshelves
- menu_book Bookshelves
- perm_media Learning Objects
- login Login
- how_to_reg Request Instructor Account
- hub Instructor Commons
Margin Size
- Download Page (PDF)
- Download Full Book (PDF)
- Periodic Table
- Physics Constants
- Scientific Calculator
- Reference & Cite
- Tools expand_more
- Readability
selected template will load here
This action is not available.
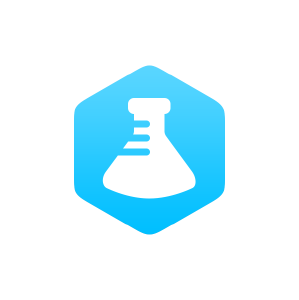
6: Types of Chemical Reactions (Experiment)
- Last updated
- Save as PDF
- Page ID 93998
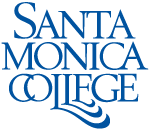
- Santa Monica College
\( \newcommand{\vecs}[1]{\overset { \scriptstyle \rightharpoonup} {\mathbf{#1}} } \)
\( \newcommand{\vecd}[1]{\overset{-\!-\!\rightharpoonup}{\vphantom{a}\smash {#1}}} \)
\( \newcommand{\id}{\mathrm{id}}\) \( \newcommand{\Span}{\mathrm{span}}\)
( \newcommand{\kernel}{\mathrm{null}\,}\) \( \newcommand{\range}{\mathrm{range}\,}\)
\( \newcommand{\RealPart}{\mathrm{Re}}\) \( \newcommand{\ImaginaryPart}{\mathrm{Im}}\)
\( \newcommand{\Argument}{\mathrm{Arg}}\) \( \newcommand{\norm}[1]{\| #1 \|}\)
\( \newcommand{\inner}[2]{\langle #1, #2 \rangle}\)
\( \newcommand{\Span}{\mathrm{span}}\)
\( \newcommand{\id}{\mathrm{id}}\)
\( \newcommand{\kernel}{\mathrm{null}\,}\)
\( \newcommand{\range}{\mathrm{range}\,}\)
\( \newcommand{\RealPart}{\mathrm{Re}}\)
\( \newcommand{\ImaginaryPart}{\mathrm{Im}}\)
\( \newcommand{\Argument}{\mathrm{Arg}}\)
\( \newcommand{\norm}[1]{\| #1 \|}\)
\( \newcommand{\Span}{\mathrm{span}}\) \( \newcommand{\AA}{\unicode[.8,0]{x212B}}\)
\( \newcommand{\vectorA}[1]{\vec{#1}} % arrow\)
\( \newcommand{\vectorAt}[1]{\vec{\text{#1}}} % arrow\)
\( \newcommand{\vectorB}[1]{\overset { \scriptstyle \rightharpoonup} {\mathbf{#1}} } \)
\( \newcommand{\vectorC}[1]{\textbf{#1}} \)
\( \newcommand{\vectorD}[1]{\overrightarrow{#1}} \)
\( \newcommand{\vectorDt}[1]{\overrightarrow{\text{#1}}} \)
\( \newcommand{\vectE}[1]{\overset{-\!-\!\rightharpoonup}{\vphantom{a}\smash{\mathbf {#1}}}} \)
- To perform and observe the results of a variety of chemical reactions.
- To become familiar with the observable signs of chemical reactions.
- To identify the products formed in chemical reactions and predict when a reaction will occur.
- To write balanced equations for the reactions studied.
- To use the results from the single replacement reactions to devise a partial activity series.
Matter undergoes three kinds of change: physical, chemical, and nuclear. While the composition of a chemical substance is not altered by physical changes (such as freezing and evaporation), chemical changes, or reactions, result in the formation of new substances when bonds are formed and/or broken. Some relatively simple but common types of chemical reactions are illustrated in this experiment. Examples and descriptions of each reaction type appear in the following section.
Chemical reactions are often accompanied by observable changes as they occur. These include:
- Color change.
- Formation of a precipitate—noted as the formation of a cloudy solution, formation of a gel, or
- an obvious solid.
- Evolution of a gas—noted as bubbling in the solution.
- Appearance or disappearance of distinct separation between two or more liquids.
- Evolution of heat—noted as a temperature increase.
- Absorption of heat—noted as a temperature decrease.
- Plating out of one metal on another.
- Decomposition, pitting, or the disappearance of a solid metal
One or more of these changes may occur in the reactions that are performed in this experiment.
Reaction Types
Please note that some reactions may be classified as more than one type of reaction; for example, combination and decomposition reactions that involve elemental substances are also oxidation-reduction reactions.
Combination Reactions (also called Synthesis Reactions) occur when two or more substances, elements or compounds, combine to form one new substance. For example, hydrogen and oxygen gases combine to give water:
\[\ce{2H2 (g) + O2 (g) -> 2H2O(l)}\]
Decomposition Reactions occur when a compound breaks apart to yield two or more new substances. As an example, potassium chlorate decomposes when heated to yield potassium chloride and oxygen gas. Potassium chlorate is one of the ingredients used in match heads. This reaction occurs as the match ignites, causing the match head to be surrounded by an oxygen atmosphere.
\[\ce{2KClO3 (s) -> 2KCl(s) + 3O2 (g)}\]
Displacement Reactions (also called Single Replacement Reactions) involve the displacement of one element in a compound by another element. Several examples of displacement reactions are given below.
- Displacement of one metal by another:
\[\ce{Pb (s) + Cu(NO3)2 (aq) -> Cu (s) + Pb(NO3)2 (aq)}\]
- Displacement of hydrogen gas from an acid by a metal:
\[\ce{Mg(s) + 2HCl(aq) -> MgCl2 (aq) + H2 (g)}\]
- Displacement of hydrogen gas from water by a metal:
\[\ce{2 K (s) + 2 H2O (l) -> 2 KOH (aq) + H2 (g)}\]
- Displacement of one halogen by another:
\[\ce{Cl2 (aq) + NaBr (aq) -> NaCl (aq) + Br2 (aq)}\]
Displacement reactions are also classified as oxidation-reduction reactions. For example, in the first reaction given above, elemental lead is oxidized to lead(II) and copper is reduced from copper(II) to elemental copper. Two electrons are transferred from lead to copper in this process:
\[ \ce{Pb (s) -> Pb^{2+} (aq) + 2 e^-} \quad \quad \text{oxidation of lead}\]
\[ \ce{Cu^{2+} (aq) + 2e^- -> Cu (s)} \quad \quad \text{reduction of copper}\]
The ability of one metal to displace another depends on their relative ease of oxidation—a more active metal (one that is more easily oxidized) displaces a less active metal. In the first reaction above, lead is more active than copper. The relative activities of metals can be tabulated in an activity series, ranking the metals by relative ease of oxidation. A metal that displaces hydrogen gas from acid is more active than hydrogen. A metal that displaces hydrogen gas from acid, but not from water, is less active than one that can displace hydrogen from both acids and water. The ease with which a substance is oxidized is quantified as its standard oxidation potential; you will learn more about this in the second semester of General Chemistry.
Exchange Reactions (also called Double Replacement or Metathesis Reactions) occur when two compounds that form ions in solution react by switching ion partners. Thus, these reactions have the general form:
\[\ce{AB + CD -> AD + CB}\]
One of three conditions must be met for these reactions to occur: (1) the formation of an insoluble ionic compound, observed as a precipitate, (2) the formation of a gas, or (3) the production of water from hydroxide and hydrogen ions (an example of an acid-base neutralization). In each case one of the products results by combining two ions that are removed from the solution by the reaction.
Precipitation Reactions occur when aqueous solutions of two ionic compounds are mixed and the ions combine to make a compound that is insoluble in water (the precipitate). For example, sodium phosphate can be used in an exchange reaction to precipitate calcium ions out of hard water as calcium phosphate, a reaction that is used in some commercial water softeners.
\[\ce{3 CaCl2 (aq) + 2 Na3PO4 (aq) -> Ca3(PO4)2 (s) + 6 NaCl (aq)}\]
The solubility behavior of the ions that you will be using is summarized in the following table:
Gas Forming Reactions typically go to completion because one or more of the products are removed from the reaction vessel via the formation of a gas, which leaves the reaction mixture as bubbles. Gases thus produced include hydrogen sulfide, sulfur dioxide, carbon dioxide and ammonia.
Hydrogen sulfide, \(\ce{H2S}\), is formed by the direct combination of an acid (source of \(\ce{H^{+}}\)) and the sulfide ion:
\[\ce{Na2S(aq) + 2HCl(aq) -> H2S(g) + 2NaCl(aq)}\]
Sulfur dioxide, \(\ce{SO2}\), is formed by the decomposition of sulfurous acid, which is initially formed in a reaction between an acid and the sulfite ion:
\[\ce{Na2SO3 (s) + 2 HCl (aq) -> H2SO3 (aq) + 2 NaCl (aq)}\]
\[\ce{H2SO3 (aq) -> H2O (l) + SO2 (g)}\]
\[\ce{Na2SO3 (s)+2HCl(aq) -> H2O(l) + SO2 (g) + 2NaCl(aq)}\]
Carbon dioxide, \(\ce{CO2}\), is formed by the decomposition of carbonic acid, which is initially formed in a reaction between an acid and the carbonate ion:
\[\ce{Na2CO3 (s) + 2 HCl (aq) -> H2CO3 (aq) + 2 NaCl (aq)}\]
\[\ce{H2CO3 (aq) -> H2O (l) + CO2 (g)}\]
\[\ce{Na2CO3 (s)+2HCl(aq) -> H2O(l) + CO2 (g) + 2NaCl(aq)}\]
Ammonia, \(\ce{NH3}\), is formed from the combination of ammonium and hydroxide ions:
\[\ce{NH4Cl (aq) + NaOH (aq) -> NaCl (aq) + H2O (l) + NH3 (g)}\]
Neutralization Reactions (also called Acid-Base Reactions) involve the transfer of a proton (\(\ce{H^+}\)) from the acid to the base. Water is always a product when the base contains the hydroxide ion (see example below). Some of the gas-forming reactions may also be classified as neutralization reactions.
\[\ce{HCl (aq) + NaOH (aq) -> NaCl (aq) + H2O (l)}\]
Materials and Equipment
Solids: \(\ce{Mg}\), \(\ce{CuSO4*5H2O}\), \(\ce{Ca}\), \(\ce{Cu}\), \(\ce{Zn}\), \(\ce{NaHCO3}\) Solutions: 6 M \(\ce{HCl}\), 6 M \(\ce{NaOH}\), 6 M \(\ce{H2SO4}\), 1 M \(\ce{NH4NO3}\), and 0.1 M solutions of \(\ce{CuSO4}\), \(\ce{ZnSO4}\), \(\ce{AgNO3}\), \(\ce{NaCl}\), \(\ce{Ni(NO3)2}\), \(\ce{Pb(NO3)2}\), and \(\ce{K2CrO4}\)
Equipment: crucible tongs, one large test tube, two small test tubes, ten small test tubes, test tube holder, test tube rack, 100-mL beaker, red litmus paper, Bunsen Burner Supplies for Instructor Demonstrations: \(\ce{CaO}\) solution (prepared in advance by stockroom), sucrose, 18 M \(\ce{H2SO4}\), distilled water, \(\ce{Na}\), means of cutting \(\ce{Na}\) and removing from its storage vessel, two 100-mL beakers, straw, red and blue litmus paper, glass stirring rod
- Do not stare directly at the magnesium when it burns as the light can hurt your eyes.
- Do not touch metals with your hands.
Instructions for Performing each Reaction
Perform each of the following reactions except those that are to be demonstrated by your instructor. You will use 1 mL of solution in many of the reactions; estimate this by drops (typically 12-15 drops from the reagent bottle dispenser) or by measuring 1 mL once in your graduated cylinder and then transferring it to a test tube to determine how far it fills the test tube. Record your observations on the data page as you complete each reaction. Make sure that you observe the results of every reaction even if you didn’t actually mix the chemicals yourself. Then write a balanced equation for each reaction. Be sure to include the states of all compounds in your equations (solid, liquid, aqueous, or gas). If no reaction occurs write the words "no reaction" (or NR) instead of the products in your balanced equation and indicate why your think there was no reaction. Unless otherwise indicated dispose of all waste in the waste container provided. Do not put metal strips in the sink.
Part A: Combination Reactions
- Instructor Demonstration: Pour about 35 mL of a clear saturated solution containing calcium oxide into a 100 mL beaker. Allow the solution to stand for about 15 minutes. Observe. Use a straw to blow bubbles into the solution for a few seconds. Observe the solution again
- Hold a small strip of magnesium metal (used in flashbulbs and fireworks) in your crucible tongs and ignite the metal in the hot portion of a burner flame. Don't forget to note the color and composition of the residue left on the tongs.
Part B: Decomposition Reactions
- Instructor Demonstration: Perform this reaction in the fume hood. Fill a 100 mL beaker about one-third full of granulated sugar (sucrose, \(\ce{C12H22O11}\)). Add about 20 mL of concentrated (18 M) sulfuric acid and stir until mixed well. Continue stirring until the mixture darkens. Observe. Do not touch the reaction products or the beaker with your hands; use a stirring rod to guide the solid product that forms.
- Place a small amount (an amount that will fit on the end of a spatula) of solid copper(II) sulfate pentahydrate in a medium test tube. Use a test tube holder to hold the tube at about a 45 o angle and heat in a burner flame for a few minutes, remembering not to point the tube at anyone in the room. Note any changes in the appearance of the solid and anything else that appears in the test tube. Allow the solid to cool and add a few drops of water. Observe. Dispose of the copper compound in the waste container.
Part C: Displacement Reactions
Use 1 mL of each solution unless otherwise specified. For reactions involving metals, use just one piece of metal. Do not put the metal pieces in the sink. If no discernable initial change is noted, let the reaction mixture stand for at least five to ten minutes before observing again. Not all of the combinations will yield observable reactions. Repeat the reaction if there is any doubt about whether a reaction occurred or not.
- Instructor Demonstration: Cautiously add a small piece of sodium metal to water. Test the resulting solution with red and blue litmus papers (red litmus paper will turn blue in the presence of a base; blue litmus will turn red in the presence of an acid).
- Calcium metal and water (15 mL) in a large test tube
- Zinc metal and water
- Copper metal and 6 M hydrochloric acid
- Zinc metal and 6 M hydrochloric acid
- Zinc metal and 0.1 M copper(II) sulfate
- Copper metal and 0.1 M zinc sulfate
- Copper metal and 0.1 M silver nitrate
Part D: Exchange Reactions
Use 1 mL of each solution unless otherwise specified. Be sure to mix the solutions well.
- 0.1 M silver nitrate and 0.1 M sodium chloride
- 0.1 M nickel(II) nitrate and three drops of 6 M sodium hydroxide
- 0.1 M lead(II) nitrate and 0.1 M potassium chromate
- 1 M ammonium nitrate and 6 M sodium hydroxide. Warm the test tube gently by passing it back and forth through a burner flame. Hold a strip of moistened red litmus paper in the tube without letting it come in contact with the sides of the tube and note any color changes to the paper. Remove the tube from the flame and quickly and cautiously note the smell.
- Place 5 mL of 6 M hydrochloric acid in a 100 mL beaker. Carefully add several spatulas of solid sodium bicarbonate. Observe.
- Combine about 5 mL each of 6 M sodium hydroxide and 6 M sulfuric acid in a large test tube. Mix with a stirring rod. Cautiously feel the outside of the test tube. If you cannot detect anything, make sure that you used the correct concentrations of acid and base. Be very careful with the concentrated sulfuric acid; it is very caustic and can dissolve skin and clothing.
Pre-laboratory Assignment: Types of Reactions
- Many of the reactions use 1 mL of solution. How can you estimate this volume?
- Do you need to dry the test tubes before using them for the reactions in this experiment? Why or why not?
- The following reactions are performed, and the results are shown below. Use these results to determine the relative activities of the two elements involved in each reaction next to that reaction. Then place the elements gold, hydrogen, zinc and tin in an activity series in order of decreasing activity.
\[\ce{Sn(s) + HCl(aq) -> H2(g) + SnCl2(aq)} \quad \quad \quad \text{______ >______}\]
\[\ce{Au (s) + Sn(NO3)2 (aq) ->} \text{NR} \quad \quad \quad \quad \text{______ >______}\]
\[\ce{Au(s) + HCl ->} \text{NR} \quad \quad\quad \quad \text{______ >______}\]
\[\ce{Zn (s) + Sn(NO3)2 (aq) -> Zn(NO3)2 (aq) + Sn (s)} \quad \text{______ >______}\]
From most active (most easily oxidized) to least active:
______>______>______>______
Now use the above results to write products for the reactions below. Write NR if no reaction is expected.
\[\ce{Sn (s) + Zn(NO3)2 (aq) ->}\]
\[\ce{Zn (s) + Au(NO3)3 (aq) ->}\]
- Suppose that each of the following pairs of aqueous solutions is combined. For those where a reaction is expected, write a balanced formula equation, with state labels, for the reaction that occurs. If no reaction is expected, indicate this and explain why no reaction is expected.
- Barium chloride + potassium sulfate
- Aluminum nitrate + sodium chloride
- Sodium hydroxide + phosphoric acid
Lab Report: Types of Reactions
Record your observations on these data pages as you perform each reaction. Write a balanced formula equation with state labels for each reaction. If no reaction occurs, follow the instructions in the Procedure.
- Calcium oxide and carbon dioxide (demonstration)
- Magnesium and oxygen
- Sucrose and sulfuric acid catalyst (demonstration)
- Thermal decomposition of copper(II) sulfate pentahydrate, \(\ce{CuSO4*5H2O}\)
In addition to providing observations and an equation for each reaction, use your results to determine the relative activities of the two elements involved in each reaction.
- Sodium and water (demonstration)
- Calcium and water
- Zinc and water
- Copper and hydrochloric acid
- Zinc and hydrochloric acid
- Zinc and copper(II) sulfate
- Copper and zinc sulfate
- Copper and silver nitrate
Arrange copper, silver, calcium, zinc, and hydrogen in an activity series from most active to least active on the basis of the results from the displacement reactions that you performed. Recall that a more active metal displaces a less active metal, a more active metal to is needed to displace hydrogen from water than to displace it from an acid, and that a metal that displaces hydrogen from acid is ranked as more active than hydrogen.
________>________>________>________>________
most active (most easily oxidized) to least active
- Silver nitrate and sodium chloride
- Nickel(II) nitrate and sodium hydroxide
- Lead(II) nitrate and potassium chromate
- Ammonium nitrate and sodium hydroxide
- Hydrochloric acid and sodium bicarbonate
- Sulfuric acid and sodium hydroxide
Academia.edu no longer supports Internet Explorer.
To browse Academia.edu and the wider internet faster and more securely, please take a few seconds to upgrade your browser .
Enter the email address you signed up with and we'll email you a reset link.
- We're Hiring!
- Help Center
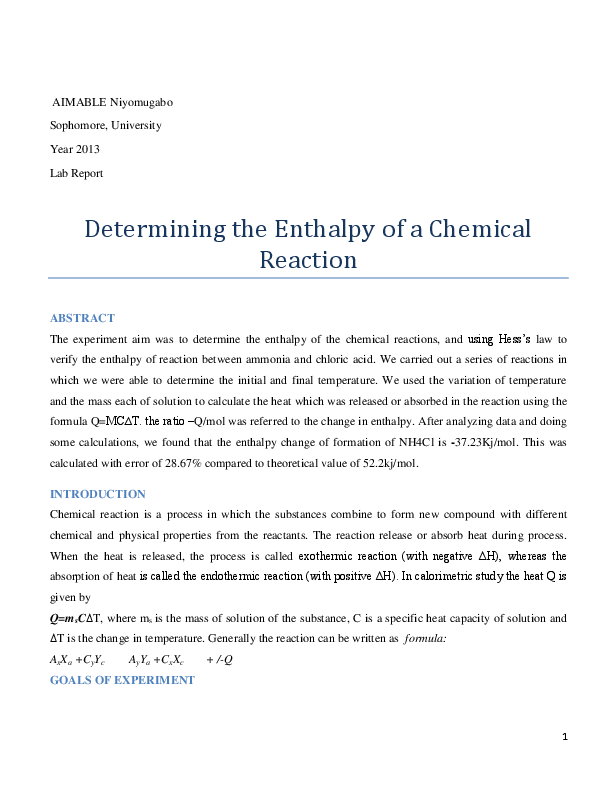
Physical Chemistry Lab: Determining the Enthalpy of a Chemical Reaction

The aim of experiment was to determine the enthalpy of the chemical reactions, and using Hess’s law to verify the enthalpy of reaction between ammonia and chloric acid. We carried out a series of reactions in which we were able to determine the initial and final temperature. We used the variation of temperature and the mass each of solution to calculate the heat which was released or absorbed in the reaction using the formula Q=MC∆T. the ratio –Q/mol was referred to the change in enthalpy. After analyzing data and doing some calculations, we found that the enthalpy change of formation of NH4Cl is -37.23Kj/mol. This was calculated with error of 28.67% compared to theoretical value of 52.2kj/mol.
Related Papers
aimi athirah
The purpose of the experiment was to investigate the enthalpy changes in several reaction and relationship of three exothermic reaction with hess’law.
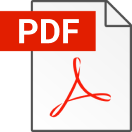
KRYSTEL IRIS DE CASTRO
The experiment aims to deduce the heat of formation of NaCl(s) by applying Hess’s Law. To complete the series of thermochemical equations, the enthalpies of neutralization of NaOH(aq) and HCl(aq) and dissolution of NaCl(s) were determined using the formula derived from the First Law of Thermodynamics. To compute for the enthalpy of the reaction, the number of moles of the reactant, the mass of the solution, the specific heat of water (4.18 J/g-°C), and the difference in temperature before and after the reaction were obtained. A Styrofoam cup calorimeter isolated the system and measured the temperature changes in the reaction. For Part A, the reaction was found to be exothermic, with an average enthalpy of neutralization of -55.835 kJ/mol. For Part B, the average enthalpy of dissolution of sodium chloride attained was +3.80 kJ/mol. This implies that the reaction was endothermic. The enthalpies of all the reactions in the series were then added to calculate the overall enthalpy, which corresponds to the heat of formation of NaCl(s). The sum yields a value for the heat of formation of NaCl(s) of -407.905 kJ/mol. This results to a 0.73% error when compared to the theoretical value of -410.9 kJ/mol.
Jeffren Chong Tat Loon
Maria Tania
RELATED TOPICS
- We're Hiring!
- Help Center
- Find new research papers in:
- Health Sciences
- Earth Sciences
- Cognitive Science
- Mathematics
- Computer Science
- Academia ©2024

Faraday Discussions
Six-dimensional quantum dynamics of an eley–rideal reaction between gaseous and adsorbed hydrogen atoms on cu(111).

* Corresponding authors
a Department of Chemical Physics, Key Laboratory of Precision and Intelligent Chemistry, Key Laboratory of Surface and Interface Chemistry and Energy Catalysis of Anhui Higher Education Institutes, University of Science and Technology of China, Hefei, Anhui 230026, China E-mail: [email protected]
b Department of Chemistry, Southern University of Science and Technology, Shenzhen, P. R. China E-mail: [email protected]
c Hefei National Laboratory, University of Science and Technology of China, Hefei, China
In the form of direct abstraction of a surface adsorbate by a gaseous projectile, the Eley–Rideal (ER) reaction at the gas–surface interface manifests interesting dynamics. Unfortunately, high-dimensional quantum dynamical (QD) studies for ER reactions remain very challenging, which demands a large configuration space and the coordinate transformation of wavefunctions. Here, we report the first six-dimensional (6D) fully coupled quantum scattering method for studying the ER reaction between gas phase H(D) atoms and adsorbed D(H) atoms on a rigid Cu(111) surface. Reaction probabilities and product rovibrational state distributions obtained by this 6D model are found to be quite different from those obtained by reduced-dimensional QD models, demonstrating the high-dimensional nature of the ER reaction. Using two distinct potential energy surfaces (PESs), we further discuss the influence of the PES on the calculated product vibrational and rotational state distributions, in comparison with experimental results. The lateral corrugation and the exothermicity of the PES are found to play a critical role in controlling the energy disposal in the ER reaction.
- This article is part of the themed collection: New directions in molecular scattering
Article information

Download Citation
Permissions.

L. Xiong, L. Zhang, B. Zhao and B. Jiang, Faraday Discuss. , 2024, Advance Article , DOI: 10.1039/D3FD00163F
This article is licensed under a Creative Commons Attribution-NonCommercial 3.0 Unported Licence . You can use material from this article in other publications, without requesting further permission from the RSC, provided that the correct acknowledgement is given and it is not used for commercial purposes.
To request permission to reproduce material from this article in a commercial publication , please go to the Copyright Clearance Center request page .
If you are an author contributing to an RSC publication, you do not need to request permission provided correct acknowledgement is given.
If you are the author of this article, you do not need to request permission to reproduce figures and diagrams provided correct acknowledgement is given. If you want to reproduce the whole article in a third-party commercial publication (excluding your thesis/dissertation for which permission is not required) please go to the Copyright Clearance Center request page .
Read more about how to correctly acknowledge RSC content .
Social activity
Search articles by author.
This article has not yet been cited.
Advertisements

IMAGES
VIDEO
COMMENTS
The state of matter of reactants and products is designated with the symbols (s) for solids, (l) for liquids, and (g) for gases. Chemical reaction, a process in which one or more substances, the reactants, are converted to one or more different substances, the products. Substances are either chemical elements or compounds.
Covers ~150 million reactions from 1840 to present including organometallics, total syntheses of natural products, and biotransformation reactions. ... as well as CODEN, ISBN, or ISSN codes. The CASSI database contains a listing of publications indexed by Chemical Abstracts Service (CAS) since 1907. Search CASSI. Common Chemistry Circle arrow ...
A chemical reaction is a process in which some substances, called reactants, change into different substances, called products. During the reaction, chemical bonds break in the reactants and new chemical bonds form in the products. Types of chemical reactions include synthesis, decomposition, replacement, and combustion reactions.
Browse 215 authentic chemical reaction abstract stock photos, high-res images, and pictures, or explore additional chain reaction or innovation stock images to find the right photo at the right size and resolution for your project.
Unlock the world of chemical reactions! Get ready to decode the language of chemistry, using symbols and equations to gain a deeper understanding of how substances interact and change at the atomic level. We'll also explore the underlying energy shifts that drive these reactions, laying a solid foundation for more advanced chemical concepts.
Abstract. Chemical kinetics is the study of the rates of chemical reactions, the factors that affect these rates, and the reaction mechanisms by which reactions occur. Reaction rates vary greatly ...
A chemical reaction is a chemical change, which means the starting materials are chemically different from the ending materials. In contrast, matter also changes form via physical changes. But, in a physical change, the chemical identity of matter does not change. For example, when you melt an ice cube into liquid water, the chemical identity ...
Understanding these reactions is not an abstract concept for a chemist in a far off laboratory, rather it is critical to understanding life and the world around us. To truly master chemical reactions, we need to understand the quantitative aspect of these reactions, something referred to as stoichiometry , and a concept we will discuss in ...
288282. Chemical change is a central concept in chemistry. The goal of chemists is to know how and why a substance changes in the presence of another substance or even by itself. Because there are tens of millions of known substances, there are a huge number of possible chemical reactions. In this chapter, we will find that many of these ...
Studying chemical reactions involves the knowledge of the reaction mechanism. Despite activation barriers describing the kinetics or reaction energies reflecting thermodynamic aspects, identifying the underlying physics and chemistry along the reaction path contributes essentially to the overall understanding of reaction mechanisms, especially for catalysis. In the past years the reaction ...
Growth of Chemical Abstracts. The early history of Chemical Abstracts is the story of Evan J. Crane, who served as editor for 43 years. Crane succeeded John Miller, editor for a brief time in 1914 following Austin Patterson's resignation. Crane joined Chemical Abstracts in 1911 at the age of 22 as an associate editor; he became acting editor in 1915 after Miller's resignation.
Chemical reaction data in journal articles, patents, and even electronic laboratory notebooks are currently stored in various formats, often unstructured, which presents a significant barrier to downstream applications, including the training of machine-learning models. We present the Open Reaction Database (ORD), an open-access schema and infrastructure for structuring and sharing organic ...
The chemical reaction was a simple one: hydrogen combining with oxygen to produce water. Many combustion reactions occur with a hydrocarbon, a compound made up solely of carbon and hydrogen. The products of the combustion of hydrocarbons are always carbon dioxide and water. Many hydrocarbons are used as fuel because their combustion releases ...
Chemical Abstracts - Chemistry - LibGuides at University of Texas at Austin. Chemical Abstracts was published in print from 1907 to 2009 by the American Chemical Society. In the decades before computer databases, large indexes like CA were the standard entry point into the scientific literature. Using them effectively was a skill that required ...
The endothermic and exothermic reactions experiment is an exciting and informative chemistry exploration that students should try. By observing and comparing the heat changes in different reactions, students can gain a deeper understanding of energy transfer and the concepts of endothermic and exothermic processes. Learn more: Education.com. 47.
Abstract. In chemical reaction engineering, one of the main tasks is the designing of chemical reactors. To achieve that, a rate law must be developed based on the analysis of rate data. Formulating rate laws from rate data is somewhat challenging in heterogeneous catalysis, mainly because these rate laws do not usually follow standard power ...
Abstract. For more than 20 years the science teachers, the researchers in science education, the educationists and the other pedagogues have been acquainted with the model of educational reconstruction. ... The chemical reaction rate is associated with the concept of chemical reaction in the way, that the conception of chemical reaction rate we ...
ABSTRACT . Over the last decade, increased attention to reaction dynamics, combined with the intensive application of computers in chemical studies, mathematical modeling of chemical processes, and mechanistic studies has brought graph theory to the forefront of research. ... Chemical Reaction Networks: A Graph-Theoretical Approach elegantly ...
Abstract. In this paper we consider the Brusselator and the Sel'kov model, which describes the irreversible reaction of glycolysis in the regime of self-sustained oscillations. We show that these two differently constructed models can be reduced to a single equation — a generalized Rayleigh equation. The physical basis for this generality is ...
Gas Forming Reactions typically go to completion because one or more of the products are removed from the reaction vessel via the formation of a gas, which leaves the reaction mixture as bubbles. Gases thus produced include hydrogen sulfide, sulfur dioxide, carbon dioxide and ammonia. Hydrogen sulfide, \(\ce{H2S}\), is formed by the direct combination of an acid (source of \(\ce{H^{+}}\)) and ...
Different thermally induced cyclization reactions of oligo- (phenylene-1,2-ethynylenes) were observed. Abstract Observing the intricate chemical transformation of an individual molecule as it undergoes a complex reaction is a long-standing challenge in molecular imaging.
Types of Chemical Reactions. William Albert Noyes; Cite this: J. Am. Chem. Soc. 1933, 55, 2, 656-661. Publication Date (Print): February 1, 1933. Publication History. ... Note: In lieu of an abstract, this is the article's first page. Cited By. This article has not yet been cited by other publications. Partners 1155 Sixteenth Street N.W. ...
AIMABLE Niyomugabo Sophomore, University Year 2013 Lab Report Determining the Enthalpy of a Chemical Reaction ABSTRACT The experiment aim was to determine the enthalpy of the chemical reactions, and using Hess's law to verify the enthalpy of reaction between ammonia and chloric acid. We carried out a series of reactions in which we were able ...
Abstract In the form of direct abstraction of a surface adsorbate by a gaseous projectile, the Eley-Rideal (ER) reaction at the gas-surface interface manifests interesting dynamics. Unfortunately, high-dimensional quantum dynamical (QD) studies for ER reactions remain very challenging, which demands a large configuration space and the ...
Aqueous zinc-ion batteries (AZIBs) are promising candidates for next-generation electrochemical energy storage systems owing to their abundant resources, high safety, great theoretical capacity, and environmental friendliness. Nonetheless, instability of Zn metal anodes caused by Zn dendrites and various side reactions poses a challenge to their practical feasibility. Optimizing the ...
Abstract. Introduction. Taxanes and platinums are first line treatment of breast cancer for early stages and metastatic disease and constitute the most commonly implicated chemotherapeutics in hypersensitivity reactions.1 Clinical manifestations range from mild skin reactions to anaphylaxis.2 Hypersensitivity reactions (HSR) to paclitaxel and carboplatin occur in a significant percentage of ...