
An official website of the United States government
The .gov means it’s official. Federal government websites often end in .gov or .mil. Before sharing sensitive information, make sure you’re on a federal government site.
The site is secure. The https:// ensures that you are connecting to the official website and that any information you provide is encrypted and transmitted securely.
- Publications
- Account settings
Preview improvements coming to the PMC website in October 2024. Learn More or Try it out now .
- Advanced Search
- Journal List
- Springer Nature - PMC COVID-19 Collection


An Introduction to COVID-19
Simon james fong.
4 Department of Computer and Information Science, University of Macau, Taipa, Macau, China
Nilanjan Dey
5 Department of Information Technology, Techno International New Town, Kolkata, West Bengal India
Jyotismita Chaki
6 School of Information Technology and Engineering, Vellore Institute of Technology, Vellore, Tamil Nadu India
A novel coronavirus (CoV) named ‘2019-nCoV’ or ‘2019 novel coronavirus’ or ‘COVID-19’ by the World Health Organization (WHO) is in charge of the current outbreak of pneumonia that began at the beginning of December 2019 near in Wuhan City, Hubei Province, China [1–4]. COVID-19 is a pathogenic virus. From the phylogenetic analysis carried out with obtainable full genome sequences, bats occur to be the COVID-19 virus reservoir, but the intermediate host(s) has not been detected till now.
A Brief History of the Coronavirus Outbreak
A novel coronavirus (CoV) named ‘2019-nCoV’ or ‘2019 novel coronavirus’ or ‘COVID-19’ by the World Health Organization (WHO) is in charge of the current outbreak of pneumonia that began at the beginning of December 2019 near in Wuhan City, Hubei Province, China [ 1 – 4 ]. COVID-19 is a pathogenic virus. From the phylogenetic analysis carried out with obtainable full genome sequences, bats occur to be the COVID-19 virus reservoir, but the intermediate host(s) has not been detected till now. Though three major areas of work already are ongoing in China to advise our awareness of the pathogenic origin of the outbreak. These include early inquiries of cases with symptoms occurring near in Wuhan during December 2019, ecological sampling from the Huanan Wholesale Seafood Market as well as other area markets, and the collection of detailed reports of the point of origin and type of wildlife species marketed on the Huanan market and the destination of those animals after the market has been closed [ 5 – 8 ].
Coronaviruses mostly cause gastrointestinal and respiratory tract infections and are inherently categorized into four major types: Gammacoronavirus, Deltacoronavirus, Betacoronavirus and Alphacoronavirus [ 9 – 11 ]. The first two types mainly infect birds, while the last two mostly infect mammals. Six types of human CoVs have been formally recognized. These comprise HCoVHKU1, HCoV-OC43, Middle East Respiratory Syndrome coronavirus (MERS-CoV), Severe Acute Respiratory Syndrome coronavirus (SARS-CoV) which is the type of the Betacoronavirus, HCoV229E and HCoV-NL63, which are the member of the Alphacoronavirus. Coronaviruses did not draw global concern until the 2003 SARS pandemic [ 12 – 14 ], preceded by the 2012 MERS [ 15 – 17 ] and most recently by the COVID-19 outbreaks. SARS-CoV and MERS-CoV are known to be extremely pathogenic and spread from bats to palm civets or dromedary camels and eventually to humans.
COVID-19 is spread by dust particles and fomites while close unsafe touch between the infector and the infected individual. Airborne distribution has not been recorded for COVID-19 and is not known to be a significant transmission engine based on empirical evidence; although it can be imagined if such aerosol-generating practices are carried out in medical facilities. Faecal spreading has been seen in certain patients, and the active virus has been reported in a small number of clinical studies [ 18 – 20 ]. Furthermore, the faecal-oral route does not seem to be a COVID-19 transmission engine; its function and relevance for COVID-19 need to be identified.
For about 18,738,58 laboratory-confirmed cases recorded as of 2nd week of April 2020, the maximum number of cases (77.8%) was between 30 and 69 years of age. Among the recorded cases, 21.6% are farmers or employees by profession, 51.1% are male and 77.0% are Hubei.
However, there are already many concerns regarding the latest coronavirus. Although it seems to be transferred to humans by animals, it is important to recognize individual animals and other sources, the path of transmission, the incubation cycle, and the features of the susceptible community and the survival rate. Nonetheless, very little clinical knowledge on COVID-19 disease is currently accessible and details on age span, the animal origin of the virus, incubation time, outbreak curve, viral spectroscopy, dissemination pathogenesis, autopsy observations, and any clinical responses to antivirals are lacking among the serious cases.
How Different and Deadly COVID-19 is Compared to Plagues in History
COVID-19 has reached to more than 150 nations, including China, and has caused WHO to call the disease a worldwide pandemic. By the time of 2nd week of April 2020, this COVID-19 cases exceeded 18,738,58, although more than 1,160,45 deaths were recorded worldwide and United States of America became the global epicentre of coronavirus. More than one-third of the COVID-19 instances are outside of China. Past pandemics that have existed in the past decade or so, like bird flu, swine flu, and SARS, it is hard to find out the comparison between those pandemics and this coronavirus. Following is a guide to compare coronavirus with such diseases and recent pandemics that have reformed the world community.
Coronavirus Versus Seasonal Influenza
Influenza, or seasonal flu, occurs globally every year–usually between December and February. It is impossible to determine the number of reports per year because it is not a reportable infection (so no need to be recorded to municipality), so often patients with minor symptoms do not go to a physician. Recent figures placed the Rate of Case Fatality at 0.1% [ 21 – 23 ].
There are approximately 3–5 million reports of serious influenza a year, and about 250,000–500,000 deaths globally. In most developed nations, the majority of deaths arise in persons over 65 years of age. Moreover, it is unsafe for pregnant mothers, children under 59 months of age and individuals with serious illnesses.
The annual vaccination eliminates infection and severe risks in most developing countries but is nevertheless a recognized yet uncomfortable aspect of the season.
In contrast to the seasonal influenza, coronavirus is not so common, has led to fewer cases till now, has a higher rate of case fatality and has no antidote.
Coronavirus Versus Bird Flu (H5N1 and H7N9)
Several cases of bird flu have existed over the years, with the most severe in 2013 and 2016. This is usually from two separate strains—H5N1 and H7N9 [ 24 – 26 ].
The H7N9 outbreak in 2016 accounted for one-third of all confirmed human cases but remained confined relative to both coronavirus and other pandemics/outbreak cases. After the first outbreak, about 1,233 laboratory-confirmed reports of bird flu have occurred. The disease has a Rate of Case Fatality of 20–40%.
Although the percentage is very high, the blowout from individual to individual is restricted, which, in effect, has minimized the number of related deaths. It is also impossible to monitor as birds do not necessarily expire from sickness.
In contrast to the bird flu, coronavirus becomes more common, travels more quickly through human to human interaction, has an inferior cardiothoracic ratio, resulting in further total fatalities and spread from the initial source.
Coronavirus Versus Ebola Epidemic
The Ebola epidemic of 2013 was primarily centred in 10 nations, including Sierra Leone, Guinea and Liberia have the greatest effects, but the extremely high Case Fatality Rate of 40% has created this as a significant problem for health professionals nationwide [ 27 – 29 ].
Around 2013 and 2016, there were about 28,646 suspicious incidents and about 11,323 fatalities, although these are expected to be overlooked. Those who survived from the original epidemic may still become sick months or even years later, because the infection may stay inactive for prolonged periods. Thankfully, a vaccination was launched in December 2016 and is perceived to be effective.
In contrast to the Ebola, coronavirus is more common globally, has caused in fewer fatalities, has a lesser case fatality rate, has no reported problems during treatment and after recovery, does not have an appropriate vaccination.
Coronavirus Versus Camel Flu (MERS)
Camel flu is a misnomer–though camels have MERS antibodies and may have been included in the transmission of the disease; it was originally transmitted to humans through bats [ 30 – 32 ]. Like Ebola, it infected only a limited number of nations, i.e. about 27, but about 858 fatalities from about 2,494 laboratory-confirmed reports suggested that it was a significant threat if no steps were taken in place to control it.
In contrast to the camel flu, coronavirus is more common globally, has occurred more fatalities, has a lesser case fatality rate, and spreads more easily among humans.
Coronavirus Versus Swine Flu (H1N1)
Swine flu is the same form of influenza that wiped 1.7% of the world population in 1918. This was deemed a pandemic again in June 2009 an approximately-21% of the global population infected by this [ 33 – 35 ].
Thankfully, the case fatality rate is substantially lower than in the last pandemic, with 0.1%–0.5% of events ending in death. About 18,500 of these fatalities have been laboratory-confirmed, but statistics range as high as 151,700–575,400 worldwide. 50–80% of severe occurrences have been reported in individuals with chronic illnesses like asthma, obesity, cardiovascular diseases and diabetes.
In contrast to the swine flu, coronavirus is not so common, has caused fewer fatalities, has more case fatality rate, has a longer growth time and less impact on young people.
Coronavirus Versus Severe Acute Respiratory Syndrome (SARS)
SARS was discovered in 2003 as it spread from bats to humans resulted in about 774 fatalities. By May there were eventually about 8,100 reports across 17 countries, with a 15% case fatality rate. The number is estimated to be closer to 9.6% as confirmed cases are counted, with 0.9% cardiothoracic ratio for people aged 20–29, rising to 28% for people aged 70–79. Similar to coronavirus, SARS had bad results for males than females in all age categories [ 36 – 38 ].
Coronavirus is more common relative to SARS, which ended in more overall fatalities, lower case fatality rate, the even higher case fatality rate in older ages, and poorer results for males.
Coronavirus Versus Hong Kong Flu (H3N2)
The Hong Kong flu pandemic erupted on 13 July 1968, with 1–4 million deaths globally by 1969. It was one of the greatest flu pandemics of the twentieth century, but thankfully the case fatality rate was smaller than the epidemic of 1918, resulting in fewer fatalities overall. That may have been attributed to the fact that citizens had generated immunity owing to a previous epidemic in 1957 and to better medical treatment [ 39 ].
In contrast to the Hong Kong flu, coronavirus is not so common, has caused in fewer fatalities and has a higher case fatality rate.
Coronavirus Versus Spanish Flu (H1N1)
The 1918 Spanish flu pandemic was one of the greatest occurrences of recorded history. During the first year of the pandemic, lifespan in the US dropped by 12 years, with more civilians killed than HIV/AIDS in 24 h [ 40 – 42 ].
Regardless of the name, the epidemic did not necessarily arise in Spain; wartime censors in Germany, the United States, the United Kingdom and France blocked news of the disease, but Spain did not, creating the misleading perception that more cases and fatalities had occurred relative to its neighbours
This strain of H1N1 eventually affected more than 500 million men, or 27% of the world’s population at the moment, and had deaths of between 40 and 50 million. At the end of 1920, 1.7% of the world’s people had expired of this illness, including an exceptionally high death rate for young adults aged between 20 and 40 years.
In contrast to the Spanish flu, coronavirus is not so common, has caused in fewer fatalities, has a higher case fatality rate, is more harmful to older ages and is less risky for individuals aged 20–40 years.
Coronavirus Versus Common Cold (Typically Rhinovirus)
Common cold is the most common illness impacting people—Typically, a person suffers from 2–3 colds each year and the average kid will catch 6–8 during the similar time span. Although there are more than 200 cold-associated virus types, infections are uncommon and fatalities are very rare and typically arise mainly in extremely old, extremely young or immunosuppressed cases [ 43 , 44 ].
In contrast to the common cold, coronavirus is not so prevalent, causes more fatalities, has more case fatality rate, is less infectious and is less likely to impact small children.
Reviews of Online Portals and Social Media for Epidemic Information Dissemination
As COVID-19 started to propagate across the globe, the outbreak contributed to a significant change in the broad technology platforms. Where they once declined to engage in the affairs of their systems, except though the possible danger to public safety became obvious, the advent of a novel coronavirus placed them in a different interventionist way of thought. Big tech firms and social media are taking concrete steps to guide users to relevant, credible details on the virus [ 45 – 48 ]. And some of the measures they’re doing proactively. Below are a few of them.
Facebook started adding a box in the news feed that led users to the Centers for Disease Control website regarding COVID-19. It reflects a significant departure from the company’s normal strategy of placing items in the News Feed. The purpose of the update, after all, is personalization—Facebook tries to give the posts you’re going to care about, whether it is because you’re connected with a person or like a post. In the virus package, Facebook has placed a remarkable algorithmic thumb on the scale, potentially pushing millions of people to accurate, authenticated knowledge from a reputable source.
Similar initiatives have been adopted by Twitter. Searching for COVID-19 will carry you to a page highlighting the latest reports from public health groups and credible national news outlets. The search also allows for common misspellings. Twitter has stated that although Russian-style initiatives to cause discontent by large-scale intelligence operations have not yet been observed, a zero-tolerance approach to network exploitation and all other attempts to exploit their service at this crucial juncture will be expected. The problem has the attention of the organization. It also offers promotional support to public service agencies and other non-profit groups.
Google has made a step in making it better for those who choose to operate or research from home, offering specialized streaming services to all paying G Suite customers. Google also confirmed that free access to ‘advanced’ Hangouts Meet apps will be rolled out to both G Suite and G Suite for Education clients worldwide through 1st July. It ensures that companies can hold meetings of up to 250 people, broadcast live to up to about 100,000 users within a single network, and archive and export meetings to Google Drive. Usually, Google pays an additional $13 per person per month for these services in comparison to G Suite’s ‘enterprise’ membership, which adds up to a total of about $25 per client each month.
Microsoft took a similar move, introducing the software ‘Chat Device’ to help public health and protection in the coronavirus epidemic, which enables collaborative collaboration via video and text messaging. There’s an aspect of self-interest in this. Tech firms are offering out their goods free of charge during periods of emergency for the same purpose as newspapers are reducing their paywalls: it’s nice to draw more paying consumers.
Pinterest, which has introduced much of the anti-misinformation strategies that Facebook and Twitter are already embracing, is now restricting the search results for ‘coronavirus’, ‘COVID-19’ and similar words for ‘internationally recognized health organizations’.
Google-owned YouTube, traditionally the most conspiratorial website, has recently introduced a connection to the World Health Organization virus epidemic page to the top of the search results. In the early days of the epidemic, BuzzFeed found famous coronavirus conspiratorial videos on YouTube—especially in India, where one ‘explain’ with a false interpretation of the sources of the disease racketeered 13 million views before YouTube deleted it. Yet in the United States, conspiratorial posts regarding the illness have failed to gain only 1 million views.
That’s not to suggest that misinformation doesn’t propagate on digital platforms—just as it travels through the broader Internet, even though interaction with friends and relatives. When there’s a site that appears to be under-performing in the global epidemic, it’s Facebook-owned WhatsApp, where the Washington Post reported ‘a torrent of disinformation’ in places like Nigeria, Indonesia, Peru, Pakistan and Ireland. Given the encrypted existence of the app, it is difficult to measure the severity of the problem. Misinformation is also spread in WhatsApp communities, where participation is restricted to about 250 individuals. Knowledge of one category may be readily exchanged with another; however, there is a considerable amount of complexity of rotating several groups to peddle affected healing remedies or propagate false rumours.
Preventative Measures and Policies Enforced by the World Health Organization (WHO) and Different Countries
Coronavirus is already an ongoing epidemic, so it is necessary to take precautions to minimize both the risk of being sick and the transmission of the disease.
WHO Advice [ 49 ]
- Wash hands regularly with alcohol-based hand wash or soap and water.
- Preserve contact space (at least 1 m/3 feet between you and someone who sneezes or coughs).
- Don’t touch your nose, head and ears.
- Cover your nose and mouth as you sneeze or cough, preferably with your bent elbow or tissue.
- Try to find early medical attention if you have fatigue, cough and trouble breathing.
- Take preventive precautions if you are in or have recently go to places where coronavirus spreads.
The first person believed to have become sick because of the latest virus was near in Wuhan on 1 December 2019. A formal warning of the epidemic was released on 31 December. The World Health Organization was informed of the epidemic on the same day. Through 7 January, the Chinese Government addressed the avoidance and regulation of COVID-19. A curfew was declared on 23 January to prohibit flying in and out of Wuhan. Private usage of cars has been banned in the region. Chinese New Year (25 January) festivities have been cancelled in many locations [ 50 ].
On 26 January, the Communist Party and the Government adopted more steps to contain the COVID-19 epidemic, including safety warnings for travellers and improvements to national holidays. The leading party has agreed to prolong the Spring Festival holiday to control the outbreak. Universities and schools across the world have already been locked down. Many steps have been taken by the Hong Kong and Macau governments, in particular concerning schools and colleges. Remote job initiatives have been placed in effect in many regions of China. Several immigration limits have been enforced.
Certain counties and cities outside Hubei also implemented travel limits. Public transit has been changed and museums in China have been partially removed. Some experts challenged the quality of the number of cases announced by the Chinese Government, which constantly modified the way coronavirus cases were recorded.
Italy, a member state of the European Union and a popular tourist attraction, entered the list of coronavirus-affected nations on 30 January, when two positive cases in COVID-19 were identified among Chinese tourists. Italy has the largest number of coronavirus infections both in Europe and outside of China [ 51 ].
Infections, originally limited to northern Italy, gradually spread to all other areas. Many other nations in Asia, Europe and the Americas have tracked their local cases to Italy. Several Italian travellers were even infected with coronavirus-positive in foreign nations.
Late in Italy, the most impacted coronavirus cities and counties are Lombardia, accompanied by Veneto, Emilia-Romagna, Marche and Piedmonte. Milan, the second most populated city in Italy, is situated in Lombardy. Other regions in Italy with coronavirus comprised Campania, Toscana, Liguria, Lazio, Sicilia, Friuli Venezia Giulia, Umbria, Puglia, Trento, Abruzzo, Calabria, Molise, Valle d’Aosta, Sardegna, Bolzano and Basilicata.
Italy ranks 19th of the top 30 nations getting high-risk coronavirus airline passengers in China, as per WorldPop’s provisional study of the spread of COVID-19.
The Italian State has taken steps like the inspection and termination of large cultural activities during the early days of the coronavirus epidemic and has gradually declared the closing of educational establishments and airport hygiene/disinfection initiatives.
The Italian National Institute of Health suggested social distancing and agreed that the broader community of the country’s elderly is a problem. In the meantime, several other nations, including the US, have recommended that travel to Italy should be avoided temporarily, unless necessary.
The Italian government has declared the closing (quarantine) of the impacted areas in the northern region of the nation so as not to spread to the rest of the world. Italy has declared the immediate suspension of all to-and-fro air travel with China following coronavirus discovery by a Chinese tourist to Italy. Italian airlines, like Ryan Air, have begun introducing protective steps and have begun calling for the declaration forms to be submitted by passengers flying to Poland, Slovakia and Lithuania.
The Italian government first declined to permit fans to compete in sporting activities until early April to prevent the potential transmission of coronavirus. The step ensured players of health and stopped event cancellations because of coronavirus fears. Two days of the declaration, the government cancelled all athletic activities owing to the emergence of the outbreak asking for an emergency. Sports activities in Veneto, Lombardy and Emilia-Romagna, which recorded coronavirus-positive infections, were confirmed to be temporarily suspended. Schools and colleges in Italy have also been forced to shut down.
Iran announced the first recorded cases of SARS-CoV-2 infection on 19 February when, as per the Medical Education and Ministry of Health, two persons died later that day. The Ministry of Islamic Culture and Guidance has declared the cancellation of all concerts and other cultural activities for one week. The Medical Education and Ministry of Health has also declared the closing of universities, higher education colleges and schools in many cities and regions. The Department of Sports and Culture has taken action to suspend athletic activities, including football matches [ 52 ].
On 2 March 2020, the government revealed plans to train about 300,000 troops and volunteers to fight the outbreak of the epidemic, and also send robots and water cannons to clean the cities. The State also developed an initiative and a webpage to counter the epidemic. On 9 March 2020, nearly 70,000 inmates were immediately released from jail owing to the epidemic, presumably to prevent the further dissemination of the disease inside jails. The Revolutionary Guards declared a campaign on 13 March 2020 to clear highways, stores and public areas in Iran. President Hassan Rouhani stated on 26 February 2020 that there were no arrangements to quarantine areas impacted by the epidemic and only persons should be quarantined. The temples of Shia in Qom stayed open to pilgrims.
South Korea
On 20 January, South Korea announced its first occurrence. There was a large rise in cases on 20 February, possibly due to the meeting in Daegu of a progressive faith community recognized as the Shincheonji Church of Christ. Any citizens believed that the hospital was propagating the disease. As of 22 February, 1,261 of the 9,336 members of the church registered symptoms. A petition was distributed calling for the abolition of the church. More than 2,000 verified cases were registered on 28 February, increasing to 3,150 on 29 February [ 53 ].
Several educational establishments have been partially closing down, including hundreds of kindergartens in Daegu and many primary schools in Seoul. As of 18 February, several South Korean colleges had confirmed intentions to delay the launch of the spring semester. That included 155 institutions deciding to postpone the start of the semester by two weeks until 16 March, and 22 institutions deciding to delay the start of the semester by one week until 9 March. Also, on 23 February 2020, all primary schools, kindergartens, middle schools and secondary schools were declared to postpone the start of the semester from 2 March to 9 March.
South Korea’s economy is expected to expand by 1.9%, down from 2.1%. The State has given 136.7 billion won funding to local councils. The State has also coordinated the purchase of masks and other sanitary supplies. Entertainment Company SM Entertainment is confirmed to have contributed five hundred million won in attempts to fight the disease.
In the kpop industry, the widespread dissemination of coronavirus within South Korea has contributed to the cancellation or postponement of concerts and other programmes for kpop activities inside and outside South Korea. For instance, circumstances such as the cancellation of the remaining Asian dates and the European leg for the Seventeen’s Ode To You Tour on 9 February 2020 and the cancellation of all Seoul dates for the BTS Soul Tour Map. As of 15 March, a maximum of 136 countries and regions provided entry restrictions and/or expired visas for passengers from South Korea.
The overall reported cases of coronavirus rose significantly in France on 12 March. The areas with reported cases include Paris, Amiens, Bordeaux and Eastern Haute-Savoie. The first coronaviral death happened in France on 15 February, marking it the first death in Europe. The second death of a 60-year-old French national in Paris was announced on 26 February [ 54 ].
On February 28, fashion designer Agnès B. (not to be mistaken with Agnès Buzyn) cancelled fashion shows at the Paris Fashion Week, expected to continue until 3 March. On a subsequent day, the Paris half-marathon, planned for Sunday 1 March with 44,000 entrants, was postponed as one of a series of steps declared by Health Minister Olivier Véran.
On 13 March, the Ligue de Football Professional disbanded Ligue 1 and Ligue 2 (France’s tier two professional divisions) permanently due to safety threats.
Germany has a popular Regional Pandemic Strategy detailing the roles and activities of the health care system participants in the case of a significant outbreak. Epidemic surveillance is carried out by the federal government, like the Robert Koch Center, and by the German governments. The German States have their preparations for an outbreak. The regional strategy for the treatment of the current coronavirus epidemic was expanded by March 2020. Four primary goals are contained in this plan: (1) to minimize mortality and morbidity; (2) to guarantee the safety of sick persons; (3) to protect vital health services and (4) to offer concise and reliable reports to decision-makers, the media and the public [ 55 ].
The programme has three phases that may potentially overlap: (1) isolation (situation of individual cases and clusters), (2) safety (situation of further dissemination of pathogens and suspected causes of infection), (3) prevention (situation of widespread infection). So far, Germany has not set up border controls or common health condition tests at airports. Instead, while at the isolation stage-health officials are concentrating on recognizing contact individuals that are subject to specific quarantine and are tracked and checked. Specific quarantine is regulated by municipal health authorities. By doing so, the officials are seeking to hold the chains of infection small, contributing to decreased clusters. At the safety stage, the policy should shift to prevent susceptible individuals from being harmed by direct action. By the end of the day, the prevention process should aim to prevent cycles of acute treatment to retain emergency facilities.
United States
The very first case of coronavirus in the United States was identified in Washington on 21 January 2020 by an individual who flew to Wuhan and returned to the United States. The second case was recorded in Illinois by another individual who had travelled to Wuhan. Some of the regions with reported novel coronavirus infections in the US are California, Arizona, Connecticut, Illinois, Texas, Wisconsin and Washington [ 56 ].
As the epidemic increased, requests for domestic air travel decreased dramatically. By 4 March, U.S. carriers, like United Airlines and JetBlue Airways, started growing their domestic flight schedules, providing generous unpaid leave to workers and suspending recruits.
A significant number of universities and colleges cancelled classes and reopened dormitories in response to the epidemic, like Cornell University, Harvard University and the University of South Carolina.
On 3 March 2020, the Federal Reserve reduced its goal interest rate from 1.75% to 1.25%, the biggest emergency rate cut following the 2008 global financial crash, in combat the effect of the recession on the American economy. In February 2020, US businesses, including Apple Inc. and Microsoft, started to reduce sales projections due to supply chain delays in China caused by the COVID-19.
The pandemic, together with the subsequent financial market collapse, also contributed to greater criticism of the crisis in the United States. Researchers disagree about when a recession is likely to take effect, with others suggesting that it is not unavoidable, while some claim that the world might already be in recession. On 3 March, Federal Reserve Chairman Jerome Powell reported a 0.5% (50 basis point) interest rate cut from the coronavirus in the context of the evolving threats to economic growth.
When ‘social distance’ penetrated the national lexicon, disaster response officials promoted the cancellation of broad events to slow down the risk of infection. Technical conferences like E3 2020, Apple Inc.’s Worldwide Developers Conference (WWDC), Google I/O, Facebook F8, and Cloud Next and Microsoft’s MVP Conference have been either having replaced or cancelled in-person events with internet streaming events.
On February 29, the American Physical Society postponed its annual March gathering, planned for March 2–6 in Denver, Colorado, even though most of the more than 11,000 physicist attendees already had arrived and engaged in the pre-conference day activities. On March 6, the annual South to Southwest (SXSW) seminar and festival planned to take place from March 13–22 in Austin, Texas, was postponed after the city council announced a local disaster and forced conferences to be shut down for the first time in 34 years.
Four of North America’s major professional sports leagues—the National Hockey League (NHL), National Basketball Association (NBA), Major League Soccer (MLS) and Major League Baseball (MLB) —jointly declared on March 9 that they would all limit the media access to player accommodations (such as locker rooms) to control probable exposure.
Emergency Funding to Fight the COVID-19
COVID-19 pandemic has become a common international concern. Different countries are donating funds to fight against it [ 57 – 60 ]. Some of them are mentioned here.
China has allocated about 110.48 billion yuan ($15.93 billion) in coronavirus-related funding.
Foreign Minister Mohammad Javad Zarif said that Iran has requested the International Monetary Fund (IMF) of about $5 billion in emergency funding to help to tackle the coronavirus epidemic that has struck the Islamic Republic hard.
President Donald Trump approved the Emergency Supplementary Budget Bill to support the US response to a novel coronavirus epidemic. The budget plan would include about $8.3 billion in discretionary funding to local health authorities to promote vaccine research for production. Trump originally requested just about $2 billion to combat the epidemic, but Congress quadrupled the number in its version of the bill. Mr. Trump formally announced a national emergency that he claimed it will give states and territories access to up to about $50 billion in federal funding to tackle the spread of the coronavirus outbreak.
California politicians approved a plan to donate about $1 billion on the state’s emergency medical responses as it readies hospitals to fight an expected attack of patients because of the COVID-19 pandemic. The plans, drawn up rapidly in reaction to the dramatic rise in reported cases of the virus, would include the requisite funds to establish two new hospitals in California, with the assumption that the state may not have the resources to take care of the rise in patients. The bill calls for an immediate response of about $500 million from the State General Fund, with an additional about $500 million possible if requested.
India committed about $10 million to the COVID-19 Emergency Fund and said it was setting up a rapid response team of physicians for the South Asian Association for Regional Cooperation (Saarc) countries.
South Korea unveiled an economic stimulus package of about 11.7 trillion won ($9.8 billion) to soften the effects of the biggest coronavirus epidemic outside China as attempts to curb the disease exacerbate supply shortages and drain demand. Of the 11,7 trillion won expected, about 3.2 trillion won would cover up the budget shortfall, while an additional fiscal infusion of about 8.5 trillion won. An estimated 10.3 trillion won in government bonds will be sold this year to fund the extra expenditure. About 2.3 trillion won will be distributed to medical establishments and would support quarantine operations, with another 3.0 trillion won heading to small and medium-sized companies unable to pay salaries to their employees and child care supports.
The Swedish Parliament announced a set of initiatives costing more than 300 billion Swedish crowns ($30.94 billion) to help the economy in the view of the coronavirus pandemic. The plan contained steps like the central government paying the entire expense of the company’s sick leave during April and May, and also the high cost of compulsory redundancies owing to the crisis.
In consideration of the developing scenario, an updating of this strategy is planned to take place before the end of March and will recognize considerably greater funding demands for the country response, R&D and WHO itself.
Artificial Intelligence, Data Science and Technological Solutions Against COVID-19
These days, Artificial Intelligence (AI) takes a major role in health care. Throughout a worldwide pandemic such as the COVID-19, technology, artificial intelligence and data analytics have been crucial in helping communities cope successfully with the epidemic [ 61 – 65 ]. Through the aid of data mining and analytical modelling, medical practitioners are willing to learn more about several diseases.
Public Health Surveillance
The biggest risk of coronavirus is the level of spreading. That’s why policymakers are introducing steps like quarantines around the world because they can’t adequately monitor local outbreaks. One of the simplest measures to identify ill patients through the study of CCTV images that are still around us and to locate and separate individuals that have serious signs of the disease and who have touched and disinfected the related surfaces. Smartphone applications are often used to keep a watch on people’s activities and to assess whether or not they have come in touch with an infected human.
Remote Biosignal Measurement
Many of the signs such as temperature or heartbeat are very essential to overlook and rely entirely on the visual image that may be misleading. However, of course, we can’t prevent someone from checking their blood pressure, heart or temperature. Also, several advances in computer vision can predict pulse and blood pressure based on facial skin examination. Besides, there are several advances in computer vision that can predict pulse and blood pressure based on facial skin examination.
Access to public records has contributed to the development of dashboards that constantly track the virus. Several companies are designing large data dashboards. Face recognition and infrared temperature monitoring technologies have been mounted in all major cities. Chinese AI companies including Hanwang Technology and SenseTime have reported having established a special facial recognition system that can correctly identify people even though they are covered.
IoT and Wearables
Measurements like pulse are much more natural and easier to obtain from tracking gadgets like activity trackers and smartwatches that nearly everybody has already. Some work suggests that the study of cardiac activity and its variations from the standard will reveal early signs of influenza and, in this case, coronavirus.
Chatbots and Communication
Apart from public screening, people’s knowledge and self-assessment may also be used to track their health. If you can check your temperature and pulse every day and monitor your coughs time-to-time, you can even submit that to your record. If the symptoms are too serious, either an algorithm or a doctor remotely may prescribe a person to stay home, take several other preventive measures, or recommend a visit from the doctor.
Al Jazeera announced that China Mobile had sent text messages to state media departments, telling them about the citizens who had been affected. The communications contained all the specifics of the person’s travel history.
Tencent runs WeChat, and via it, citizens can use free online health consultation services. Chatbots have already become important connectivity platforms for transport and tourism service providers to keep passengers up-to-date with the current transport protocols and disturbances.
Social Media and Open Data
There are several people who post their health diary with total strangers via Facebook or Twitter. Such data becomes helpful for more general research about how far the epidemic has progressed. For consumer knowledge, we may even evaluate the social network group to attempt to predict what specific networks are at risk of being viral.
Canadian company BlueDot analyses far more than just social network data: for instance, global activities of more than four billion passengers on international flights per year; animal, human and insect population data; satellite environment data and relevant knowledge from health professionals and journalists, across 100,000 news posts per day covering 65 languages. This strategy was so successful that the corporation was able to alert clients about coronavirus until the World Health Organization and the Centers for Disease Control and Prevention notified the public.
Automated Diagnostics
COVID-19 has brought up another healthcare issue today: it will not scale when the number of patients increases exponentially (actually stressed doctors are always doing worse) and the rate of false-negative diagnosis remains very high. Machine learning therapies don’t get bored and scale simply by growing computing forces.
Baidu, the Chinese Internet company, has made the Lineatrfold algorithm accessible to the outbreak-fighting teams, according to the MIT Technology Review. Unlike HIV, Ebola and Influenza, COVID-19 has just one strand of RNA and it can mutate easily. The algorithm is also simpler than other algorithms that help to determine the nature of the virus. Baidu has also developed software to efficiently track large populations. It has also developed an Ai-powered infrared device that can detect a difference in the body temperature of a human. This is currently being used in Beijing’s Qinghe Railway Station to classify possibly contaminated travellers where up to 200 individuals may be checked in one minute without affecting traffic movement, reports the MIT Review.
Singapore-based Veredus Laboratories, a supplier of revolutionary molecular diagnostic tools, has currently announced the launch of the VereCoV detector package, a compact Lab-on-Chip device able to detect MERS-CoV, SARS-CoV and COVID-19, i.e. Wuhan Coronavirus, in a single study.
The VereCoV identification package is focused on VereChip technology, a Lab-on-Chip device that incorporates two important molecular biological systems, Polymerase Chain Reaction (PCR) and a microarray, which will be able to classify and distinguish within 2 h MERS-CoV, SARS-CoV and COVID-19 with high precision and responsiveness.
This is not just the medical activities of healthcare facilities that are being charged, but also the corporate and financial departments when they cope with the increase in patients. Ant Financials’ blockchain technology helps speed-up the collection of reports and decreases the number of face-to-face encounters with patients and medical personnel.
Companies like the Israeli company Sonovia are aiming to provide healthcare systems and others with face masks manufactured from their anti-pathogenic, anti-bacterial cloth that depends on metal-oxide nanoparticles.
Drug Development Research
Aside from identifying and stopping the transmission of pathogens, the need to develop vaccinations on a scale is also needed. One of the crucial things to make that possible is to consider the origin and essence of the virus. Google’s DeepMind, with their expertise in protein folding research, has rendered a jump in identifying the protein structure of the virus and making it open-source.
BenevolentAI uses AI technologies to develop medicines that will combat the most dangerous diseases in the world and is also working to promote attempts to cure coronavirus, the first time the organization has based its product on infectious diseases. Within weeks of the epidemic, it used its analytical capability to recommend new medicines that might be beneficial.
Robots are not vulnerable to the infection, and they are used to conduct other activities, like cooking meals in hospitals, doubling up as waiters in hotels, spraying disinfectants and washing, selling rice and hand sanitizers, robots are on the front lines all over to deter coronavirus spread. Robots also conduct diagnostics and thermal imaging in several hospitals. Shenzhen-based firm Multicopter uses robotics to move surgical samples. UVD robots from Blue Ocean Robotics use ultraviolet light to destroy viruses and bacteria separately. In China, Pudu Technology has introduced its robots, which are usually used in the cooking industry, to more than 40 hospitals throughout the region. According to the Reuters article, a tiny robot named Little Peanut is distributing food to passengers who have been on a flight from Singapore to Hangzhou, China, and are presently being quarantined in a hotel.
Colour Coding
Using its advanced and vast public service monitoring network, the Chinese government has collaborated with software companies Alibaba and Tencent to establish a colour-coded health ranking scheme that monitors millions of citizens every day. The mobile device was first introduced in Hangzhou with the cooperation of Alibaba. This applies three colours to people—red, green or yellow—based on their transportation and medical records. Tencent also developed related applications in the manufacturing centre of Shenzhen.
The decision of whether an individual will be quarantined or permitted in public spaces is dependent on the colour code. Citizens will sign into the system using pay wallet systems such as Alibaba’s Alipay and Ant’s wallet. Just those citizens who have been issued a green colour code will be permitted to use the QR code in public spaces at metro stations, workplaces, and other public areas. Checkpoints are in most public areas where the body temperature and the code of individual are tested. This programme is being used by more than 200 Chinese communities and will eventually be expanded nationwide.
In some of the seriously infected regions where people remain at risk of contracting the infection, drones are used to rescue. One of the easiest and quickest ways to bring emergency supplies where they need to go while on an epidemic of disease is by drone transportation. Drones carry all surgical instruments and patient samples. This saves time, improves the pace of distribution and reduces the chance of contamination of medical samples. Drones often operate QR code placards that can be checked to record health records. There are also agricultural drones distributing disinfectants in the farmland. Drones, operated by facial recognition, are often used to warn people not to leave their homes and to chide them for not using face masks. Terra Drone uses its unmanned drones to move patient samples and vaccination content at reduced risk between the Xinchang County Disease Control Center and the People’s Hospital. Drones are often used to monitor public areas, document non-compliance with quarantine laws and thermal imaging.
Autonomous Vehicles
At a period of considerable uncertainty to medical professionals and the danger to people-to-people communication, automated vehicles are proving to be of tremendous benefit in the transport of vital products, such as medications and foodstuffs. Apollo, the Baidu Autonomous Vehicle Project, has joined hands with the Neolix self-driving company to distribute food and supplies to a big hospital in Beijing. Baidu Apollo has also provided its micro-car packages and automated cloud driving systems accessible free of charge to virus-fighting organizations.
Idriverplus, a Chinese self-driving organization that runs electrical street cleaning vehicles, is also part of the project. The company’s signature trucks are used to clean hospitals.
This chapter provides an introduction to the coronavirus outbreak (COVID-19). A brief history of this virus along with the symptoms are reported in this chapter. Then the comparison between COVID-19 and other plagues like seasonal influenza, bird flu (H5N1 and H7N9), Ebola epidemic, camel flu (MERS), swine flu (H1N1), severe acute respiratory syndrome, Hong Kong flu (H3N2), Spanish flu and the common cold are included in this chapter. Reviews of online portal and social media like Facebook, Twitter, Google, Microsoft, Pinterest, YouTube and WhatsApp concerning COVID-19 are reported in this chapter. Also, the preventive measures and policies enforced by WHO and different countries such as China, Italy, Iran, South Korea, France, Germany and the United States for COVID-19 are included in this chapter. Emergency funding provided by different countries to fight the COVID-19 is mentioned in this chapter. Lastly, artificial intelligence, data science and technological solutions like public health surveillance, remote biosignal measurement, IoT and wearables, chatbots and communication, social media and open data, automated diagnostics, drug development research, robotics, colour coding, drones and autonomous vehicles are included in this chapter.
REVIEW article
Coronavirus disease (covid-19): comprehensive review of clinical presentation.
- 1 Department of Medicine, King Edward Medical University/ Mayo Hospital, Lahore, Pakistan
- 2 Department of Anesthesia and Intensive Care, Post-Graduate Medical Institute/LGH, Lahore, Pakistan
- 3 Rajarshee Chhatrapati Shahu Maharaj Government Medical College, Kolhapur, India
- 4 Department of Medicine, Faculty of Medicine, University of Tlemcen, Tlemcen, Algeria
- 5 School of Tropical Medicine and Global Health, Nagasaki University, Nagasaki, Japan
- 6 Institute of Research and Development, Duy Tan University, Da Nang, Vietnam
COVID-19 is a rapidly growing pandemic with its first case identified during December 2019 in Wuhan, Hubei Province, China. Due to the rampant rise in the number of cases in China and globally, WHO declared COVID-19 as a pandemic on 11th March 2020. The disease is transmitted via respiratory droplets of infected patients during coughing or sneezing and affects primarily the lung parenchyma. The spectrum of clinical manifestations can be seen in COVID-19 patients ranging from asymptomatic infections to severe disease resulting in mortality. Although respiratory involvement is most common in COVID-19 patients, the virus can affect other organ systems as well. The systemic inflammation induced by the disease along with multisystem expression of Angiotensin Converting Enzyme 2 (ACE2), a receptor which allows viral entry into cells, explains the manifestation of extra-pulmonary symptoms affecting the gastrointestinal, cardiovascular, hematological, renal, musculoskeletal, and endocrine system. Here, we have reviewed the extensive literature available on COVID-19 about various clinical presentations based on the organ system involved as well as clinical presentation in specific population including children, pregnant women, and immunocompromised patients. We have also briefly discussed about the Multisystemic Inflammatory Syndrome occurring in children and adults with COVID-19. Understanding the various clinical presentations can help clinicians diagnose COVID-19 in an early stage and ensure appropriate measures to be undertaken in order to prevent further spread of the disease.
Introduction
COVID-19 is a growing pandemic with initial cases identified in Wuhan, Hubei province, China toward the end of December 2019. Labeled as Novel Coronavirus 2019 (2019-nCoV) initially by the Chinese Center for Disease Control and Prevention (CDC) which was subsequently renamed as Severe Acute Respiratory Syndrome-Coronavirus-2 (SARS-CoV-2) due to its homology with SARS-CoV by the International Committee on Taxonomy of Viruses (ICTV) ( 1 , 2 ). The World Health Organization (WHO) later renamed the disease caused by SARS-CoV-2 as Coronavirus Disease-2019 (COVID-19) ( 3 ). COVID-19 is primarily a disease of the respiratory system affecting lung parenchyma with fever, cough, and shortness of breath as the predominant symptoms. Recent studies have shown that it can affect multiple organ systems and cause development of extra-pulmonary symptoms. Presence of extra-pulmonary symptoms can often lead to late diagnosis and sometimes even mis-diagnosis of COVID-19 which can be detrimental to patients. As researchers globally continue to understand COVID-19 and its implications on the human body, knowledge about the various clinical presentations of COVID-19 is paramount in early diagnosing and treatment in order to decrease the morbidity and mortality caused by the disease.
Epidemiology and Pathophysiology
While studying the early transmission dynamics of COVID-19 outbreak in Wuhan, many cases were found to be linked to the Huanan wholesale seafood market. Further investigation revealed <10% of the total cases could be linked to the market which led to the conclusion of human-to-human transmission of the virus occurring through respiratory droplets and contact transmission contributing to the rise in the number of affected individuals ( 4 ). The exponential rise in the number of cases in China and reporting of cases outside China in multiple countries led WHO to declare COVID-19 as a pandemic on 11th March 2020 ( 5 ).
SARS-CoV-2 tends to infect all age groups and is transmitted via direct contact or respiratory droplets generated during coughing or sneezing by the infected patient during both symptomatic or pre-symptomatic phase of infection. Other routes of transmission include fecal-oral route and fomites along with small risk of vertical transmission from mother to child if infection occurs during third trimester of pregnancy ( 6 , 7 ). There has also been evidence of asymptomatic transmission of COVID-19 ( 8 ). The concept of super spreaders in relation to COVID-19 is emerging where a single individual either symptomatic or asymptomatic can infect a disproportionately large number of individuals in an appropriate super spreading conditions such as mass gathering due to production of large number of infectious agent for prolonged duration of time ( 9 ). As per the literature, the incubation period of COVID-19 ranges from 2 to 14 days with a mean incubation period of 3 days ( 10 ). The basic Reproduction number (Ro) of SARS-CoV-2 is 2–2.5. Each individual infected with COVID-19 can infect 2–2.5 other individuals in a naïve population which also explains the exponential growth in the number of cases ( 10 ). The disease tends to be of mild to moderate severity in roughly 80% of patients, and severe disease is associated with infants, elderly patients above 65 years, and patients with other comorbidities such as diabetes mellitus, hypertension, coronary artery disease, and other chronic conditions ( 1 , 2 ). COVID-19 has also been found to be more severe in males than in females with a case fatality rate of 2.8% in males and 1.7% in females ( 11 ). The major organ system affected by the virus is the respiratory system, but it can affect other organ systems either directly or by the effect of host immune response. SARS-CoV-2, the causative agent of COVID-19, after entering the human host initially replicates in the epithelial mucosa of the upper respiratory tract (nose and pharynx) followed by migration to the lungs where further replication of virus occurs causing transient viraemia. The virus uses Angiotensin Converting Enzyme 2 (ACE2) receptor as a primary entry to cells. ACE2 is found abundantly in the mucosal lining of the respiratory tract, vascular endothelial cells, heart, intestine, and kidney. Thus, the virus has potential for replication in all these organs. After entry into cells, the virus undergoes further rapid replication within the target cells and induces extensive epithelial and endothelial dysfunction leading to exponential inflammatory response with the production of a large amount of proinflammatory cytokines and chemokines. Activation of proinflammatory cytokines and chemokines leads to neutrophil activation and migrations and results in the characteristic cytokine storm. The immunological downregulation of ACE2 by the virus contributes to acute lung injury in COVID-19. ACE2 also regulates the renin angiotensin system (RAS); thus, downregulation of ACE2 also causes dysfunction of RAS which contributes to enhanced inflammation ( 2 , 11 – 15 ). These entire factors contribute to symptoms of COVID-19 with sepsis, multi-organ dysfunction, acute respiratory distress syndrome (ARDS), and prothrombotic state leading to an exacerbation of organ dysfunction.
Clinical Manifestation
We review here the system based clinical features of COVID-19.
Respiratory
According to report from WHO-China-Joint Mission on COVID-19, 55,924 laboratories confirmed cases of COVID-19 had fever (87.9%), dry cough (67.7%), fatigue (38.1%), sputum production (33.4%), difficulty breathing (18.6%), sore throat (13.9%), chills (11.4%), nasal congestion (4.8%), and hemoptysis (0.9%) ( 1 ).
Some patients may rapidly progress to acute lung injury and ARDS with septic shock. The median interval between the onset of initial symptoms to development of dyspnea, hospital admission, and ARDS was 5, 7, and 8 days respectively ( 10 ). Some patients with COVID-19 may have reduced oxygen saturation in blood (≤ 93%) with oxygen saturation down to 50 or 60% but remained stable without significant distress, and as such, were termed as salient hypoxia or happy hypoxia ( 16 , 17 ). Trial of oxygen therapy, prone positioning, high flow continuous positive airway pressure, non-re-breathable mask alongside trial of anticoagulation are often used to manage these patients ( 16 , 17 ). However, further study is required to define the role of these strategies in management.
The most frequent radiological abnormality among 975 patients with COVID-19 in computed tomography (CT) scan of chest was ground glass opacity (56.4%) and bilateral patchy shadowing (51.8%) ( 18 ). A scientific review of 2,814 patients have shown that the most common chest CT finding in COVID-19 patients was ground glass opacity followed by consolidation. However, the findings can vary in different patients and at various stages of diseases. Other CT findings include interlobular septal thickening, reticular pattern, crazy paving, etc. Atypical findings like air bronchogram, bronchial wall thickening, nodule, pleural effusion, and lymphadenopathy have also been noted in some studies ( 19 ). A study showed that among 877 patients with non-severe diseases and 173 patients with severe diseases, 17.9 and 2.9% of the patients did not have any detectable radiological abnormalities, respectively ( 18 ).
ENT (Ear, Nose, and Throat)
ENT manifestations are one of the most frequent symptoms encountered by physicians in COVID-19. A peculiar clinical presentation in some COVID-19 patients includes the deterioration of sense, taste (dysgeusia), and loss of smell (anosmia). A systematic review and meta-analysis of 10 studies with 1,627 participants surveyed for olfactory deterioration and 9 studies with 1,390 participants examined for gustatory symptoms demonstrated prevalence of 52.73 and 43.93% of these symptoms among COVID-19 patients, respectively. These clinical features may often present at earlier stages of the disease ( 20 ). Additionally, sore throat, rhinorrhea, nasal congestion, tonsil edema, and enlarged cervical lymph nodes are commonly seen among otolaryngological dysfunctions in patients ( 21 ). A large observational study of 1,099 COVID-19 patients reported tonsils swelling in 23 patients (2.1%), throat congestion in 19 patients (1.7%) and enlarged lymph nodes in 2 patients (0.2%) ( 18 ). This can be explained by the fact that there is a high expression of ACE2 receptors on the epithelial cells of the oral and nasal mucosa including the tongue. It has been known that the novel coronavirus has a strong binding affinity to ACE2 receptors through which it invades host cells ( 22 ). This theory may explain the exhibition of extra-respiratory symptoms including ENT manifestations as part of COVID-19 symptoms.
Cardiovascular
Cardiac manifestation in patient with COVID-19 can occur due to cardiac strain secondary to hypoxia and respiratory failure, direct effect of SARS-CoV-2 on heart or secondary to inflammation and cytokine storm, metabolic derangements, rupture of plaque and coronary occlusion by thrombus, and consequences of drugs used for treatment ( 23 – 25 ). The need for intensive care admission, non-invasive ventilation (46.3 vs. 3.9%), and invasive mechanical ventilation (22 vs. 4.2%) were higher among patients with cardiac ailments as compared to those without cardiac involvement as well as higher hospital mortality than those without myocardial involvement (51.2 vs. 4.5%) ( 26 ). These patients tend to have electrocardiographic (ECG) changes as well as elevations in high sensitivity cardiac troponin (hsCTn) and N- terminal pro-B-type natriuretic peptide (NT proBNP) which corresponded to raised inflammatory markers. Hypertension, acute and fulminant myocarditis, ventricular arrhythmias, atrial fibrillation, stress cardiomyopathy, hypotension and heart failure, acute coronary syndrome (ACS) with ST elevation or depression MI with normal coronaries have been reported ( 23 , 27 ). In a Chinese cohort of 138 patients, 16.7% had arrhythmias with risk higher among those needing ICU care with no mention of the type of arrhythmia that was present ( 28 ). Less frequently, cardiac symptoms like chest pain or tightness and palpitation can be the initial presenting features without fever producing a diagnostic dilemma. Some of these patients eventually go on to develop respiratory symptoms as diseases progress ( 29 ). Patients who have recovered from acute illness may develop arrhythmias as a result of myocardial scar and need future monitoring ( 27 ). One important point to note is use of Renin Angiotensin Aldosterone System (RAAS) modulators in patients with COVID-19. Guidelines from ACC/AHA/HFSA recommends continuing them in high risk patient based on goal directed therapy approach supported by a recent systematic review and meta-analysis conducted by Hasan et. Al. which demonstrated use of ACEI/ARB in COVID-19 patients is associated with lower odds/ hazards of mortality and development of severe/critical diseases as compared to no use of ACEI/ARB ( 30 , 31 ).
Gastrointestinal
In the initial cohort of patients from China, nausea or vomiting and diarrhea were present in 5 and 3.7% of patients ( 1 ). Review of data from 2,023 patients showed anorexia to be the most frequently occurring gastrointestinal symptom in adults. Diarrhea was the most common presenting gastrointestinal symptom in both adults and children while vomiting was found to be more common in children ( 32 ). Other rare symptoms included nausea, abdominal pain, and gastrointestinal bleeding. There have been few instances where COVID-19 patients presented with only gastrointestinal symptoms without the development of fever or respiratory symptoms at the onset and during disease progression ( 33 ). In a smaller cohort of 204 patients, 50.5% had some form of intestinal symptoms and of those, 5.8% had only intestinal symptoms while the remaining patients developed respiratory symptoms subsequently. The most common symptoms reported among them was anorexia (78.64%), non-dehydrating diarrhea (34%), vomiting (3.9%), and abdominal pain (1.94%) ( 34 ). In addition, those with GI symptoms tend to have a longer interval between symptom onset and hospital admission (9 vs. 7.3 days) possibly due to lack of clinical suspicion and delay in diagnosis. Patients with gastrointestinal symptoms tend to have higher elevation in AST and ALT indicating coexistent liver injury ( 34 ). The mechanism behind GI illness is not clearly known but could be due to direct invasion of virus via ACE2 receptor in the intestinal mucosa. This can be supported by the fact that viral RNA can be detected in stool samples of COVID-19 patients which may also hint toward possible fecal-oral transmission ( 35 ). Liver dysfunction is likely secondary to the use of hepatotoxic drugs, hypoxia induced liver injury, systemic inflammation, and multi organ failure ( 36 ).
Renal manifestation in patients with COVID-19 can occur due to direct invasion of podocytes and proximal tubular cells by SARS-CoV-2 virus, secondary endothelial dysfunction causing effacement of foot process with vacuolation and detachment of podocytes, and acute proximal tubular dysfunction ( 37 ). Furthermore, hypoxia, cytokine storm, rhabdomyolysis, nephrotoxic drugs, and overlying infections can all exacerbate renal injury ( 38 ). Based on initial reports, prevalence of Acute Kidney Injury (AKI) among COVID-19 hospitalized patients range from 0.5 to 29%. In a cohort of 701 patients, proteinuria (43.9%), hematuria (26.7%), elevated creatinine (14.4%), elevated blood urea nitrogen (13.1%), and low glomerular filtration rate (≤ 60 ml/min/1.73 m 2 ) (13.1%) were present at the time of hospital admission with 5.1% developing AKI during the illness. AKI was more prevalent among those with baseline renal impairment ( 39 ). In another large cohort of 5,449 patients, 36.6% had AKI with prevalence higher among mechanically ventilated patients compared to non-ventilated patients (89.7 vs. 21.7%) ( 40 ). Patients developing renal impairment are prone to have higher mortality within the hospital. Another point to highlight is the presentation of COVID-19 in renal transplant recipients. Due to immunosuppression, these patients are likely to have low fever at presentation with swift clinical decline and requirement for mechanical ventilation with high mortality as compared to the general population ( 41 ).
Neurological
Most patients with COVID-19 develop neurological symptoms along with respiratory symptoms during the course of illness; however, several case reports in review of literature document patient presentation of neurological dysfunction without typical symptoms of fever, cough, and difficulty breathing ( 42 ). There is a 2.5-fold enhanced risk of severe illness and increased death in patients with a history of previous stroke with similar findings among those with Parkinson's diseases. The prevalence of neurological features ranges from 6 to 36% along with hypoxic ischemic encephalopathy up to 20% in some series of patients ( 43 ). Neurological symptoms tend to occur early in the course of illness (median 1–2 days) with most common neurological features being headache, confusion, delirium, anosmia or hyposmia, dysgeusia or ageusia, altered mental status, ataxia, and seizures ( 44 ). Among patients admitted with COVID-19, the prevalence of ischemic stroke ranges from 2.5 to 5% despite receiving prophylaxis for venous thromboembolism. Patients prone to have established cardiovascular risk factors are likely to have a more severe diseases ( 43 ). Other presentations include viral encephalitis, acute necrotizing encephalopathy (ANE), infectious toxic encephalopathy, meningitis, Guillain Barre Syndrome (GBS), Miller Fisher syndrome, and polyneuritis cranialis with GBS being the first feature of COVID-19 in few cases ( 42 , 43 , 45 ). In COVID-19 patients, CNS features are possibly due to direct invasion of neurons and glial cells by SARS-CoV-2 as well as by endothelial dysfunction of blood brain barrier (BBB). Virus can gain access to CNS via hematogenous spread or retrograde movement across the olfactory bulb. The virus can be detected in CSF by RT-PCR and on brain parenchyma during autopsy. The fact that most patients develop anosmia or hyposmia during illness support this theory ( 45 ). After entry, the virus can cause reactive gliosis with activation of the inflammatory cascade. The combination of systemic inflammation, cytokine storm, and coagulation dysfunction can impair BBB function and alter brain equilibrium causing neuronal death ( 42 ).
Ocular manifestations can vary from conjunctival injection to frank conjunctivitis. In a Chinese cohort of 38 patients, 31.6% had ocular symptoms consisting primarily of conjunctivitis while conjunctival hyperemia, foreign body sensation in eye, chemosis, tearing or epiphora were more common among severe COVID-19 patients. Among them SARS-CoV-2 can be demonstrated in conjunctival as well as nasopharyngeal swab in 5.2% of patients, indicating a potential route for viral transmission ( 46 ). Conjunctivitis or tearing can be the initial presenting symptoms of COVID-19. Despite this fact, there is no documented case of severe ocular features relating to COVID-19.
Similar to other viral infections, SARS-CoV-2 can also produce varied dermatological features. A study of 88 patients from Italy showed that about 20.4% had some form of skin manifestations with 44.4% developing features at onset and duration of the disease progression ( 47 ). Maculopapular exanthem (36.1%) was identified as most common dermatological features followed by papulovesicular rash (34.7%), painful acral red purple papules (15.3%), urticaria (9.7%), livedo reticularis (2.8%), and petechiae (1.4%) ( 48 ). A study of 375 COVID-19 cases in Spain identified five different patterns of cutaneous manifestations in patients: acral areas of erythema with vesicles or pustules (pseudo-chilblain) (19%), other vesicular eruptions (9%), urticarial lesions (19%), maculopapular eruptions (47%), and livedo or necrosis (6%) ( 49 ). Majority of patients had lesions on the trunk with some experiencing lesions on hands and feet. There are case reports of COVID-19 associated with erythema multiforme and Kawasaki Disease in children ( 50 , 51 ). Pathogenesis behind skin involvement remains unclear with some features explained by small vessel vasculitis, thrombotic events like DIC, hyaline thrombus formation, acral ischemia, or the direct effect of the virus like other viral illnesses ( 52 ).
Musculoskeletal
The initial report from China revealed 14.8% of patients had myalgia or arthralgia among 55,924 COVID-19 patients. A review article reports that of 12,046 patients, fatigue was identified in 25.6% and myalgia and/or arthralgia in 15.5% with most patients reporting symptoms from the start of illness ( 53 ). There are reports suggesting myositis and rhabdomyolysis with markedly elevated creatinine kinase can occur during COVID-19 illness especially in patients with severe diseases and multi organ failure. Additionally, in some patients, rhabdomyolysis has been documented as the initial presentation of COVID-19 illness without typical respiratory symptoms ( 54 , 55 ). A case series of four patients developing acute arthritis during hospital admission for COVID-19 has been reported with exacerbation of crystal arthropathy (gout and calcium pyrophosphate diseases) but negative for SARS-CoV-2 RT-PCR in synovial fluid ( 56 ). Treatment with steroids and colchicine was used in all four cases. An important consideration to note was that all four patients developed arthritis despite previous treatment with immunomodulatory therapy (hydroxychloroquine, tocilizumab, and pulse methylprednisolone).
Hematological
As stated, COVID-19 is a systemic disease inducing systemic inflammation and occasionally cytokine storm. This can significantly impact the process of hematopoiesis and hemostasis. During early disease, normal or decreased leukocyte and lymphocyte counts were documented with marked lymphopenia as the diseases progressed, especially in those with cytokine storms and severe disease. In a study of 1,099 patients, lymphopenia, thrombocytopenia, and leukopenia were present in 83.2, 36.2, and 33.7%, respectively, with findings more marked in those with severe diseases ( 18 ). Leukocytosis in COVID-19 patients might suggest a bacterial infection or a superinfection with leukocytosis found more commonly in severe cases (11.4%) as compared to mild and moderate cases (4.8%) ( 18 ). Similarly, thrombocytopenia has been found to be more common (57.7%) in severe cases in contrast to mild and moderate cases (31.6%) ( 18 ). Lymphopenia was also linked with an increased necessity for ICU admission and the risk of ARDS. Thrombocytosis with elevated platelet to lymphocyte ratio may indicate a more marked cytokine storm ( 57 ).
Also, coagulation abnormality can manifest in the form of thrombocytopenia, prolonged prothrombin time (PT), low serum fibrinogen level, and raised D-dimer suggesting Disseminated Intravascular Coagulation (DIC) with these changes more marked in those with severe diseases ( 58 ). Raised lactate dehydrogenase (LDH) and serum ferritin were also present and correlated with the degree of systemic inflammation. In a study of 426 COVID-19 patients, C-Reactive Protein (CRP) was noted to be increased in 75–93% of patients, more commonly in patients with severe disease. Serum procalcitonin levels might not be altered at admission, but progressive increase in its value can suggest a worsening prognosis. Severe disease is linked to increased ALT, bilirubin, serum urea, creatinine, and lowered serum albumin ( 59 ). A study of 1,426 patients showed that Interleukin-6 (IL-6) were raised more in patients with severe COVID-19 than non-severe COVID-19 with progressive rise indicating an increased risk of mortality. Thus, its levels could be regarded as an important prognostic indicator for the extensive inflammation and cytokine storm in COVID-19 patients ( 60 ). Other plasma cytokines and chemokines like IL1B, IFNγ, IP10, MCP, etc. have also been found to be elevated in patients with COVID-19 both in severe and non-severe diseases. Additionally, GCSF, IP10, IL2, IL7, IL10, MCP1, MIP1A, and TNFα were increased in patients who require ICU admission which indicates that cytokine storm is associated with a severe disease ( 61 ).
Endocrine and Reproductive
From the available literature there is no doubt that diabetes mellitus is an important risk factor for COVID-19 illness and is associated with increased risk of development of severe disease. Additionally, there are case reports of subacute thyroiditis linked to SARS-CoV-2 infection ( 62 , 63 ). Based on the statement released from European Society of Endocrinology, patients with primary adrenal failure and congenital adrenal hyperplasia may have theoretically increased susceptibility to infection with higher risk of complications and ultimately mortality but there is no current evidence to support this ( 64 ). The dose of steroids may need to be doubled if there is a clinical suspicion of infection in these patients.
Several claims have been made regarding the impact of COVID-19 on male reproductive function, hypothesizing that COVID-19 can cause potential testicular damage either by binding directly to testicular ACE2 receptors, which are highly expressed in the testicles or by damaging the testis indirectly by exciting local immune system ( 65 ). A study comparing 81 male COVID-19 patients with 100 age matched healthy adults highlighted the presence of low testosterone levels, high levels of luteinizing hormone (LH), low testosterone/LH ratios, low Follicle stimulating hormone (FSH) to LH ratio, and raised serum prolactin. This may suggest a potential COVID-19 testicular damage affecting the Leydig cells in the testis ( 66 ). COVID-19 infected male patients may have reduced sperm count and decreased motility leading to diminished male fertility for 3 months post-infection ( 67 ).
Clinical Presentation in Specific Population
In children.
A case series of 72,314 cases published by the Chinese Center for Disease Control and Prevention reported that 0.9% of the total patients were between 0 and 9 years of age, and 1.2% of the total patients were between 10 and 19 years of age ( 68 ). The most common symptoms found in children are fever, (59%), cough (46%), few cases (12%) of gastrointestinal symptoms, and some cases (26%) showed no specific symptoms initially with patchy consolidation and ground glass opacities in CT chest findings ( 69 ). Chilblain-like acral eruptions, purpuric, and erythema multiforme-like lesions have been found to be more common in children and young adult patients mainly with asymptomatic or mild disease ( 70 ). Lymphopenia in children is relatively less common which is in direct contrast in cases of SARS in children where lymphopenia was more commonly noted ( 69 ).
Multisystem inflammatory syndrome (MIS) is another feared complication of Covid-19 seen in children. Abrams et al. systematically summarized the clinical evidence of 8 studies reporting MIS in 440 children. The median age of patients ranged from 7.3 to 10 years with 59% of all patients being male. The greatest proportion of patients had gastrointestinal symptoms (87%) followed by mucocutaneous symptoms (73%) and cardiovascular symptoms (71%) while fewer patients reported respiratory (47%), neurologic (22%), and musculoskeletal (21%) symptoms. Ferritin and d-dimer were elevated in 50% of patients, and C-reactive protein, interleukin-6, and fibrinogen were elevated in at least 75% of patients. Additionally, 100% of children with cardiovascular involvement reported elevated cardiac-damage markers such as Troponin. Although respiratory manifestation is most frequently expressed in adults, children with MIS exhibited less pulmonary symptoms and more of the other manifestations ( 71 ).
In Pregnant Women
The most common symptoms reported in pregnant women are fever (61.96%), cough (38.04%), malaise (30.49%), myalgia (21.43%), sore throat (12%), and dyspnea (12.05%). Other symptoms found in pregnant women are diarrhea and nasal congestion ( 72 ). In a systematic review including 92 patients, 67.4% manifested diseases at presentation with 31.7% having negative RT-PCR though they had features of viral pneumonia. Only one patient required admission to intensive care and 0% mortality. Fetal outcomes were reported as: 63.8% preterm delivery, 61.1% fetal distress, 80% Cesarean section delivery, 76.92% neonatal intensive care admission, 42.8% low birth weight, and 66.67% had lymphopenia ( 72 ). There was no evidence of vertical transmission. A study of 41 pregnant women with COVID-19 showed that consolidation was more commonly found in CT of pregnant women in contrast to ground-glass opacities in CT of non-pregnant adults ( 73 ). WHO also recommends encouraging lactating mothers with confirmed or suspected COVID-19 to begin or continue breastfeeding including 24-h rooming in, skin to skin contact, and kangaroo mother care especially in immediate postnatal period ( 74 ). On July 14th, 2020, Vivanti et al. published the first case of transplacental transmission of COVID-19 from a 23-years-old pregnant woman to her baby ( 75 ). Thereafter, more studies reported the possibility of the vertical transmission of COVID-19. In this context, Kotlayer et al. published a systematic review of 38 studies. Out of 936 neonates from COVID-19 mothers, 27 tested positive for the virus indicating a pooled proportion of 3.2% (2.2–4.3) for vertical transmission ( 7 ).
In Immuno-Compromised Population
Due to their impaired immune response, it is not surprising that immunocompromised patients with COVID-19 infection might be at greater risk of developing severe forms of the disease and co-infections in comparison to normal populations. Nevertheless, recent studies showed the association between cytokine storm syndrome and the overreaction of the immune system with COVID-19 raising the possibility that immunodeficient states might alleviate the overexpression of the host immune system and thereby prevent deadly forms of the disease ( 76 ). After the RECOVERY trial ( 77 ) that showed the efficacy of dexamethasone in lowering the mortality in severe forms of the disease, many questions were raised regarding whether immunocompromised patients have a greater or lower risk of developing severe forms of the disease. In order to address these questions, Minotti et al. recently published a systematic review that included 16 studies with 110 patients presenting mostly with cancer along with transplantation and immunodeficiency. Out of the 110 patients, 72 (65.5%) recovered without being admitted to the intensive care unit while 23 (20.9%) died ( 76 ). The authors concluded that immunosuppression in both children and adults seem to have a better disease course in comparison to normal population. One of the limitations of this study is that the conclusion was made only based on qualitative synthesis and no meta-analysis was performed. On the other hand, Gao et al. performed a meta-analysis on 8 relevant studies with 4,007 patients. The study showed that immunosuppression and immunodeficiency were associated with non-statistically significant increased risk of severe COVID-19 disease ( 78 ). Additionally, Mirzaei et al. summarized the clinical evidence of 252 HIV positive patients co-infected with COVID-19. The clinical manifestation did not differ from that of the general population. However, out of the 252 patients, 204 (80.9%) were male. Low CD4 count (<200 cells/mm 3 ) were reported for 23 of 176 patients (13.1%). COVID-19 symptoms were present in 223 patients with the most common symptoms of fever in 165 (74.0%) patients, cough in 130 (58.3%), headache in 44 (19.7%), arthralgia and myalgia in 33 (14.8%), gastrointestinal symptoms in 29 (13.0%) followed by sore throat in 18 (8.1%) patients ( 79 ). The number of deaths accounted for 36 (14.3%). Similar to the general population, immunocompromised, and HIV patients were no different in terms of clinical manifestation or severity. However, the results from these studies should be interpreted with caution and more studies are recommended to establish the link between this particular group of patients with severity of the disease.
Multisystem Involvement in COVID-19
As evident from the discussion above, SARS-CoV-2 can affect multiple organ systems and produce a wide array of clinical presentation of COVID-19. Certain studies conducted in Europe and United States have shown that COVID-19 can also have a multi-systemic presentation in individuals in form of a multi-system inflammatory syndrome (MIS) which has been found in both children and adults and is known as MIS-C and MIS-A, respectively ( 80 – 83 ).
According to a recent CDC report about MIS-A, it was found that only half of the patients with MIS-A had preceding respiratory symptoms of COVID-19 ~2–5 weeks before ( 80 ). The most common clinical signs and symptoms included fever, chest pain, palpitations, diarrhea, abdominal pain, vomiting, skin rash, etc. Nearly all patients had electro-cardiological abnormalities like arrythmias, elevated troponin levels, and electrocardiography evidence of left or right ventricular dysfunction. Even though most patients had minimal respiratory symptoms, chest imaging had features of ground glass opacity and pleural effusion. All patients had signs of elevated laboratory markers of inflammation, coagulation markers, and lymphopenia ( 80 ).
MIS-C can clinically mimic Kawasaki Disease ( 81 ). By the end of July, about 570 cases of MIS-C with COVID-19 were found in the United States ( 81 ). In MIS-C, there is involvement of at least four organ systems, most commonly the gastrointestinal system followed by cardiovascular and dermatological systems ( 81 ). Prominent signs and symptoms found in children with MIS-C were abdominal pain, vomiting, skin rash, diarrhea, hypotension, and conjunctival injection. The majority of the children needed ICU admission due to the development of severe complications including cardiac dysfunction, shock, myocarditis, coronary artery aneurysm, and acute kidney injury ( 81 ).
Association Between Clinical Presentations, COVID-19 Severity and Prognosis
Evaluation of 55,924 laboratory confirmed COVID-19 cases in China, the presence of dyspnea, respiratory rate ≥ 30/min, blood saturation levels ≤ 93%, PaO2/FiO2 ratio ≤ 300, lung infiltrates ≥ 50% of the lung fields between 12 and 48 h were associated with severe COVID-19 infection ( 1 ). Clinical signs suggestive of respiratory failure, septic shock, or multiple organ dysfunction/failure were associated with critical disease and poor prognosis ( 1 ). Individuals at highest risk of severe disease and deaths were patients with age > 80 years and associated co-morbidities such as underlying cardiovascular disease, diabetes, hypertension, chronic respiratory disease, and cancer ( 1 ). Another study done with 418 patients in Catalonia (Spain) showed that dyspnea was an important predictor of severe disease while confusion was an important predictor of death, and the presence of cough was strongly associated with good prognosis ( 84 ). Advanced age, male sex, and obesity were independent markers of poor prognosis while eosinophilia was a marker of less severe disease ( 84 ). The mortality was lower in patients with symptoms of diarrhea, arthromyalgia, headache, and loss of smell and taste sensations while low oxygen saturation, high CRP levels, and higher number of lung quadrants affected on Xray were found to be associated with severe disease and death ( 84 ).
COVID-19 is a viral illness which can cause multi-systemic manifestations. Review of existing literature concludes that SARS-CoV-2 can affect any organ system either directly or indirectly leading to a myriad of clinical presentation. The most commonly affected system is the respiratory system with presenting symptoms of fever, cough, and shortness of breath, etc. Other systems which can be affected in COVID-19 include ENT (sore throat, loss of taste, smell, and sensations, and rhinorrhea), cardiovascular system (chest pain, chest tightness, palpitations, and arrhythmias), gastrointestinal system (anorexia, diarrhea, vomiting, nausea, and abdominal pain), renal (proteinuria, hematuria, and acute kidney injury), neurological (headache, confusion, delirium, and altered mental status), ocular (conjunctival hyperemia, foreign body sensation in the eye, chemosis, and tearing), cutaneous (rash, papules, and urticaria), musculoskeletal system (myalgia and arthralgia), hematological (lymphopenia, thrombocytopenia, leukopenia, elevated inflammatory markers, and elevated coagulation markers), endocrine (low testosterone, low FSH, and high LH) and reproductive system (decreased sperm count and decreased sperm motility). Clinical presentation in specific populations like children, pregnant women, and immunocompromised people may vary which emphasizes the importance of further investigation in order to avoid late diagnosis of COVID-19. Severe multi-systemic involvement in COVID-19 in the form of MIS-C and MIS-A can cause significant morbidity and mortality if undiagnosed. The clinical presentations of respiratory failure, acute kidney injury, septic shock, cardiovascular arrest is associated with severe COVID-19 disease and can result in poor prognosis. In the light of exponentially growing pandemic, every patient presenting to hospital must be tested for SARS-CoV-2 by RT-PCR if resources are available to detect early presentations of diseases even if the features are atypical. Understanding of the various clinical presentations of COVID-19 will help the clinicians in early detection, treatment, and isolation of patients in order to contain the virus and slow down the pandemic.
Author Contributions
All authors have contributed equally to the work, and all agreed to be accountable for the content of the work.
Conflict of Interest
The authors declare that the research was conducted in the absence of any commercial or financial relationships that could be construed as a potential conflict of interest.
Acknowledgments
We would like to thank Ms. Sairah Zia (American University of Caribbean, School of Medicine, Sint Maarten), a native speaker of English, for proofreading the manuscript.
Abbreviations
ACC/AHA/HFSA, American College of Cardiology/American Heart Association/Heart Failure Society of America; IL1B, Interleukin 1B; IFNγ, Interferon Gamma; IP10, Interferon-inducible Protein 10; MCP1, Monocyte Chemoattractant Protein 1; GCSF, Granulocyte Colony Stimulating Factor; IL2, Interleukin 2; IL7, Interleukin 7; IL10, Interleukin 10; MIP1A, Macrophage Inflammatory Protein-1 alpha; TNFα, Tumor Necrosis Factor alpha.
1. Who-China-Joint-Mission-on-Covid-19-Final-Report.pdf . Available online at: https://www.who.int/docs/default-source/coronaviruse/who-china-joint-mission-on-covid-19-final-report.pdf (accessed June 1, 2020).
2. Jin Y, Yang H, Ji W, Wu W, Chen S, Zhang W, et al. Virology, epidemiology, pathogenesis, and control of COVID-19. Viruses. (2020) 12:372. doi: 10.3390/v12040372
CrossRef Full Text | Google Scholar
3. Naming the Coronavirus Disease (COVID-19) and the Virus That Causes it . (2020). Available online at: https://www.who.int/emergencies/diseases/novel-coronavirus-2019/technical-guidance/naming-the-coronavirus-disease-(covid-2019)-and-the-virus-that-causes-it (accessed June 7, 2020).
Google Scholar
4. Harapan H, Itoh N, Yufika A, Winardi W, Keam S, Te H, et al. Coronavirus disease 2019 (COVID-19): a literature review. J Infect Public Health. (2020) 13:667–73. doi: 10.1016/j.jiph.2020.03.019
PubMed Abstract | CrossRef Full Text | Google Scholar
5. WHO Director-General's opening remarks at the media briefing on COVID-19 . (2020). Available online at: https://www.who.int/dg/speeches/detail/who-director-general-s-opening-remarks-at-the-media-briefing-on-covid-19-−11-march-2020 (accessed June 7, 2020).
6. Rothan HA, Byrareddy SN. The epidemiology and pathogenesis of coronavirus disease (COVID-19) outbreak. J Autoimmun. (2020) 109:102433. doi: 10.1016/j.jaut.2020.102433
7. Kotlyar AM, Grechukhina O, Chen A, Popkhadze S, Grimshaw A, Tal O, et al. Vertical transmission of coronavirus disease 2019: a systematic review and meta-analysis. Am J Obstet Gynecol. (2020). doi: 10.1016/j.ajog.2020.07.049. [Epub ahead of print].
8. Tian S, Hu N, Lou J, Chen K, Kang X, Xiang Z, et al. Characteristics of COVID-19 infection in Beijing. J Infect. (2020) 80:401–6. doi: 10.1016/j.jinf.2020.02.018
9. Cave E. COVID-19 super-spreaders: definitional quandaries and implications. Asian Bioeth Rev. (2020) 12:235–42. doi: 10.1007/s41649-020-00118-2
10. Kakodkar P, Kaka N, Baig M. A comprehensive literature review on the clinical presentation, and management of the pandemic coronavirus disease 2019 (COVID-19). Cureus. (2020) 12:e7560. doi: 10.7759/cureus.7560
11. Tay MZ, Poh CM, Rénia L, MacAry PA, Ng LFP. The trinity of COVID-19: immunity, inflammation and intervention. Nat Rev Immunol. (2020) 20:363–74. doi: 10.1038/s41577-020-0311-8
PubMed Abstract | CrossRef Full Text
12. Veerdonk F, van de Netea MG, Deuren M, van Meer JWM, van der Mast Q, de Bruggemann RJ, et al. Kinins and cytokines in COVID-19: a comprehensive pathophysiological approach. eLife. (2020) 9:e57555. doi: 10.7554/eLife.57555
13. Ye Q, Wang B, Mao J. The pathogenesis and treatment of the ‘Cytokine Storm’ in COVID-19. J Infect. (2020) 80:607–13. doi: 10.1016/j.jinf.2020.03.037
14. Magro C, Mulvey JJ, Berlin D, Nuovo G, Salvatore S, Harp J, et al. Complement associated microvascular injury and thrombosis in the pathogenesis of severe COVID-19 infection: a report of five cases. Transl Res. (2020) 220, 1–13. doi: 10.1016/j.trsl.2020.04.007
15. Lin L, Lu L, Cao W, Li T. Hypothesis for potential pathogenesis of SARS-CoV-2 infection–a review of immune changes in patients with viral pneumonia. Emerg Microbes Infect. (2020) 9:727–32. doi: 10.1080/22221751.2020.1746199
16. Ottestad W, Seim M, Mæhlen JO. COVID-19 with silent hypoxemia. Tidsskr Den NorLegeforening. (2020) 140. doi: 10.4045/tidsskr.20.0299
17. Couzin-Frankel J. The mystery of the pandemic's “happy hypoxia.” Science . (2020) 368:455–6. doi: 10.1126/science.368.6490.455
18. Guan W, Ni Z, Hu Y, Liang W, Ou C, He J, et al. Clinical characteristics of coronavirus disease 2019 in China. N Engl J Med. (2020) 382:1708–20. doi: 10.1056/NEJMoa2002032
19. Ye Z, Zhang Y, Wang Y, Huang Z, Song B. Chest CT manifestations of new coronavirus disease 2019 (COVID-19): a pictorial review. Eur Radiol. (2020) 30:4381–9. doi: 10.1007/s00330-020-06801-0
20. Tong JY, Wong A, Zhu D, Fastenberg JH, Tham T. The prevalence of olfactory and gustatory dysfunction in COVID-19 patients: a systematic review and meta-analysis. Otolaryngol Neck Surg. (2020) 163:3–11. doi: 10.1177/0194599820926473
CrossRef Full Text
21. Krajewska J, Krajewski W, Zub K, Zatoński T. COVID-19 in otolaryngologist practice: a review of current knowledge. Eur Arch Otorhinolaryngol. (2020) 277:1885–97. doi: 10.1007/s00405-020-05968-y
22. Xu H, Zhong L, Deng J, Peng J, Dan H, Zeng X, et al. High expression of ACE2 receptor of 2019-nCoV on the epithelial cells of oral mucosa. Int J Oral Sci. (2020) 12:8. doi: 10.1038/s41368-020-0074-x
23. Akhmerov A, Marbán E. COVID-19 and the Heart. Circ Res. (2020) 126:1443–55. doi: 10.1161/CIRCRESAHA.120.317055
24. Clerkin Kevin J, Fried Justin A, Raikhelkar J, Sayer G, Griffin JM, Masoumi A, et al. COVID-19 and cardiovascular disease. Circulation. (2020) 141:1648–55. doi: 10.1161/CIRCULATIONAHA.120.046941
25. Bansal M. Cardiovascular disease and COVID-19. Diabetes Metab Syndr Clin Res Rev. (2020) 14:247–50. doi: 10.1016/j.dsx.2020.03.013
26. Shi S, Qin M, Shen B, Cai Y, Liu T, Yang F, et al. Association of cardiac injury with mortality in hospitalized patients with COVID-19 in Wuhan, China. JAMA Cardiol. (2020) 5:802–10. doi: 10.1001/jamacardio.2020.0950
27. Kochi AN, Tagliari AP, Forleo GB, Fassini GM, Tondo C. Cardiac and arrhythmic complications in patients with COVID-19. J Cardiovasc Electrophysiol. (2020) 31:1003–8. doi: 10.1111/jce.14479
28. Wang D, Hu B, Hu C, Zhu F, Liu X, Zhang J, et al. Clinical characteristics of 138 hospitalized patients with 2019 novel coronavirus–infected pneumonia in Wuhan, China. JAMA. (2020) 323:1061–9. doi: 10.1001/jama.2020.1585
29. Zheng Y-Y, Ma Y-T, Zhang J-Y, Xie X. COVID-19 and the cardiovascular system. Nat Rev Cardiol. (2020) 17:259–60. doi: 10.1038/s41569-020-0360-5
30. Patients Taking ACE-i and ARBs who Contract COVID-19 Should Continue Treatment Unless Otherwise Advised by Their Physician . American Heart Association (2020). Available online at: https://newsroom.heart.org/news/patients-taking-ace-i-and-arbs-who-contract-covid-19-should-continue-treatment-unless-otherwise-advised-by-their-physician (accessed June 27, 2020).
31. Hasan SS, Kow CS, Hadi MA, Zaidi STR, Merchant HA. Mortality and disease severity among COVID-19 patients receiving renin-angiotensin system inhibitors: a systematic review and meta-analysis. Am J Cardiovasc Drugs. (2020). doi: 10.22541/au.158880148.84250526. [Epub ahead of print].
32. Tian Y, Rong L, Nian W, He Y. Review article: gastrointestinal features in COVID-19 and the possibility of faecal transmission. Aliment Pharmacol Ther. (2020) 51:843–51. doi: 10.1111/apt.15731
33. An P, Chen H, Jiang X, Su J, Xiao Y, Ding Y, et al. Clinical Features of 2019 Novel Coronavirus Pneumonia Presented Gastrointestinal Symptoms But Without Fever Onset . Rochester, NY: Social Science Research Network (2020). Available online at: https://papers.ssrn.com/abstract=3532530 (accessed June 27, 2020). doi: 10.2139/ssrn.3532530
34. Pan L, Mu M, Yang P, Sun Y, Wang R, Yan J, et al. Clinical characteristics of covid-19 patients with digestive symptoms in hubei, china: a descriptive, cross-sectional, multicenter study. Am J Gastroenterol. (2020) 115, 766–73. doi: 10.14309/ajg.0000000000000620
35. Xiao F, Tang M, Zheng X, Liu Y, Li X, Shan H. Evidence for gastrointestinal infection of SARS-CoV-2. Gastroenterology. (2020) 158:1831–3.e3. doi: 10.1053/j.gastro.2020.02.055
36. Feng G, Zheng KI, Yan Q-Q, Rios RS, Targher G, Byrne CD, et al. COVID-19 and liver dysfunction: current insights and emergent therapeutic strategies. J Clin Transl Hepatol. (2020) 8:18–24. doi: 10.14218/JCTH.2020.00018
37. Su H, Yang M, Wan C, Yi L-X, Tang F, Zhu H-Y, et al. Renal histopathological analysis of 26 postmortem findings of patients with COVID-19 in China. Kidney Int. (2020) 98:219–27. doi: 10.1016/j.kint.2020.04.003
38. Ronco C, Reis T. Kidney involvement in COVID-19 and rationale for extracorporeal therapies. Nat Rev Nephrol. (2020) 16:308–10. doi: 10.1038/s41581-020-0284-7
39. Cheng Y, Luo R, Wang K, Zhang M, Wang Z, Dong L, et al. Kidney disease is associated with in-hospital death of patients with COVID-19. Kidney Int. (2020) 97:829–38. doi: 10.1016/j.kint.2020.03.005
40. Hirsch JS, Ng JH, Ross DW, Sharma P, Shah HH, Barnett RL, et al. Acute kidney injury in patients hospitalized with COVID-19. Kidney Int. (2020) 98:209–18. doi: 10.1016/j.kint.2020.05.006
41. Akalin E, Azzi Y, Bartash R, Seethamraju H, Parides M, Hemmige V, et al. Covid-19 and kidney transplantation. N Engl J Med. (2020) 382:2475–7. doi: 10.1056/NEJMc2011117
42. Sheraton M, Deo N, Kashyap R, Surani S. A review of neurological complications of COVID-19. Cureus. (2020) 12:e8192. doi: 10.7759/cureus.8192
43. Bridwell R, Long B, Gottlieb M. Neurologic complications of COVID-19. Am J Emerg Med. (2020) 38:1549.e3–7. doi: 10.1016/j.ajem.2020.05.024
44. Mao L, Jin H, Wang M, Hu Y, Chen S, He Q, et al. Neurologic manifestations of hospitalized patients with coronavirus disease 2019 in Wuhan, China. JAMA Neurol. (2020) 77:683–90. doi: 10.1001/jamaneurol.2020.1127
45. Ghannam M, Alshaer Q, Al-Chalabi M, Zakarna L, Robertson J, Manousakis G. Neurological involvement of coronavirus disease 2019: a systematic review. J Neurol. (2020). doi: 10.21203/rs.3.rs-31183/v1. [Epub ahead of print].
46. Dockery DM, Rowe SG, Murphy MA, Krzystolik MG. The ocular manifestations and transmission of COVID-19; recommendations for prevention. J Emerg Med. (2020) 59:137–40. doi: 10.1016/j.jemermed.2020.04.060
47. Recalcati S. Cutaneous manifestations in COVID-19: a first perspective. J Eur Acad Dermatol Venereol. (2020) 34:e212–3. doi: 10.1111/jdv.16387
48. Sachdeva M, Gianotti R, Shah M, Lucia B, Tosi D, Veraldi S, et al. Cutaneous manifestations of COVID-19: Report of three cases and a review of literature. J Dermatol Sci. (2020) 98:75–81. doi: 10.1016/j.jdermsci.2020.04.011
49. Casas CG, Català A, Hernández GC, Rodríguez-Jiménez P, Fernández-Nieto D, Lario AR-V, et al. Classification of the cutaneous manifestations of COVID-19: a rapid prospective nationwide consensus study in Spain with 375 cases. Br J Dermatol. (2020) 183:71–7. doi: 10.1111/bjd.19163
50. Jones VG, Mills M, Suarez D, Hogan CA, Yeh D, Segal JB, et al. COVID-19 and kawasaki disease: novel virus and novel case. Hosp Pediatr. (2020) 10:537–40. doi: 10.1542/hpeds.2020-0123
51. Labé P, Ly A, Sin C, Nasser M, Chapelon-Fromont E, Saïd PB, et al. Erythema multiforme and Kawasaki disease associated with COVID-19 infection in children. J Eur Acad Dermatol Venereol. (2020) 34:e539–41. doi: 10.1111/jdv.16666
52. Tang K, Wang Y, Zhang H, Zheng Q, Fang R, Sun Q. Cutaneous manifestations of the Coronavirus Disease 2019 (COVID-19): a brief review. Dermatol Ther. (2020) 33:e13528. doi: 10.1111/dth.13528
53. Cipollaro L, Giordano L, Padulo J, Oliva F, Maffulli N. Musculoskeletal symptoms in SARS-CoV-2 (COVID-19) patients. J Orthop Surg. (2020) 15:178. doi: 10.1186/s13018-020-01702-w
54. Suwanwongse K, Shabarek N. Rhabdomyolysis as a presentation of 2019 novel coronavirus disease. Cureus. (2020) 12:e7561. doi: 10.7759/cureus.7561
55. Chan KH, Farouji I, Hanoud AA, Slim J. Weakness and elevated creatinine kinase as the initial presentation of coronavirus disease 2019 (COVID-19). Am J Emerg Med. (2020) 38:1548.e1–3. doi: 10.1016/j.ajem.2020.05.015
56. López-González M-C, Peral-Garrido ML, Calabuig I, Tovar-Sugrañes E, Jovani V, Bernabeu P, et al. Case series of acute arthritis during COVID-19 admission. Ann Rheum Dis. (2020) doi: 10.1136/annrheumdis-2020-217914. [Epub ahead of print].
57. Qu R, Ling Y, Zhang Y, Wei L, Chen X, Li X, et al. Platelet-to-lymphocyte ratio is associated with prognosis in patients with coronavirus disease-19. J Med Virol. (2020) 92:1533–41. doi: 10.1002/jmv.25767
58. Levi M, Thachil J, Iba T, Levy JH. Coagulation abnormalities and thrombosis in patients with COVID-19. Lancet Haematol. (2020) 7:e438–40. doi: 10.1016/S2352-3026(20)30145-9
59. Lippi G, Plebani M. Laboratory abnormalities in patients with COVID-2019 infection. Clin Chem Lab Med CCLM. (2020) 58:1131–4. doi: 10.1515/cclm-2020-0198
60. Aziz M, Fatima R, Assaly R. Elevated interleukin-6 and severe COVID-19: a meta-analysis. J Med Virol. (2020) 92:2283–5. doi: 10.1002/jmv.25948
61. Huang C, Wang Y, Li X, Ren L, Zhao J, Hu Y, et al. Clinical features of patients infected with 2019 novel coronavirus in Wuhan, China. Lancet Lond Engl. (2020) 395:497–506. doi: 10.1016/S0140-6736(20)30183-5
62. Brancatella A, Ricci D, Viola N, Sgrò D, Santini F, Latrofa F. Subacute thyroiditis after Sars-COV-2 infection. J Clin Endocrinol Metab. (2020) 105:dgaa276. doi: 10.1210/clinem/dgaa276
63. AsfurogluKalkan E, Ates I. A case of subacute thyroiditis associated with Covid-19 infection. J Endocrinol Invest. (2020) 43:1173–4. doi: 10.1007/s40618-020-01316-3
64. Puig-Domingo M, Marazuela M, Giustina A. COVID-19 and endocrine diseases a statement from the European society of endocrinology. Endocrine. (2020) 68:2–5. doi: 10.1007/s12020-020-02294-5
65. Illiano E, Trama F, Costantini E. Could COVID-19 have an impact on male fertility? Andrologia. (2020) 52:e13654. doi: 10.1111/and.13654
66. Ma L, Xie W, Li D, Shi L, Mao Y, Xiong Y, et al. Effect of SARS-CoV-2 infection upon male gonadal function: a single center-based study. medRxiv [Preprint]. (2020). doi: 10.1101/2020.03.21.20037267
67. Segars J, Katler Q, McQueen DB, Kotlyar A, Glenn T, Knight Z, et al. Prior and novel coronaviruses, Coronavirus disease 2019 (COVID-19), and human reproduction: what is known? FertilSteril. (2020) 113:1140–9. doi: 10.1016/j.fertnstert.2020.04.025
68. CDC Weekly C, The Novel Coronavirus Pneumonia Emergency Response Epidemiology Team. The epidemiological characteristics of an outbreak of 2019 novel coronavirus diseases (COVID-19) — China, 2020. China CDC Wkly . (2020) 2:113–22. doi: 10.46234/ccdcw2020.032
69. Chang T-H, Wu J-L, Chang L-Y. Clinical characteristics and diagnostic challenges of pediatric COVID-19: a systematic review and meta-analysis. J Formos Med Assoc. (2020) 119:982–9. doi: 10.1016/j.jfma.2020.04.007
70. Wollina U, Karadag AS, Rowland-Payne C, Chiriac A, Lotti T. Cutaneous signs in COVID-19 patients: a review. Dermatol Ther. (2020) 33:e13549. doi: 10.1111/dth.13549
71. Abrams JY, Godfred-Cato SE, Oster ME, Chow EJ, Koumans EH, Bryant B, et al. Multisystem inflammatory syndrome in children associated with severe acute respiratory syndrome coronavirus 2: a systematic review. J Pediatr. (2020) 226:45–54.e1. doi: 10.1016/j.jpeds.2020.08.003
72. Smith V, Seo D, Warty R, Payne O, Salih M, Chin KL, et al. Maternal and neonatal outcomes associated with COVID-19 infection: a systematic review. PLoS ONE. (2020) 15:e0234187. doi: 10.1371/journal.pone.0234187
73. Liu H, Liu F, Li J, Zhang T, Wang D, Lan W. Clinical and CT imaging features of the COVID-19 pneumonia: Focus on pregnant women and children. J Infect. (2020) 80:e7–13. doi: 10.1016/j.jinf.2020.03.007
74. Breastfeeding and COVID-19 . (2020). Available online at: https://www.who.int/news-room/commentaries/detail/breastfeeding-and-covid-19 (accessed Jun 25, 2020).
75. Vivanti AJ, Vauloup-Fellous C, Prevot S, Zupan V, Suffee C, Do Cao J, et al. Transplacental transmission of SARS-CoV-2 infection. Nat Commun. (2020) 11:3572. doi: 10.1038/s41467-020-17436-6
76. Minotti C, Tirelli F, Barbieri E, Giaquinto C, Donà D. How is immunosuppressive status affecting children and adults in SARS-CoV-2 infection? a systematic review. J Infect. (2020) 81:e61–6. doi: 10.1016/j.jinf.2020.04.026
77. Europe PMC . (2020). Available online at: https://europepmc.org/articles/pmc7383595/bin/nejmoa2021436_appendix.pdf (accessed November 20, 2020).
78. Gao Y, Chen Y, Liu M, Shi S, Tian J. Impacts of immunosuppression and immunodeficiency on COVID-19: a systematic review and meta-analysis. J Infect. (2020) 81:e93–5. doi: 10.1016/j.jinf.2020.05.017
79. Mirzaei H, McFarland W, Karamouzian M, Sharifi H. COVID-19 among people living with HIV: a systematic review. AIDS Behav. (2020) 1–8. doi: 10.1007/s10461-020-02983-2. [Epub ahead of print].
80. Morris SB, Schwartz NG, Patel P, Abbo L, Beauchamps L, Balan S, et al. Case series of multisystem inflammatory syndrome in adults associated with SARS-CoV-2 infection — United Kingdom and United States, March–August 2020. Morb Mortal Wkly Rep. (2020) 69:1450–6. doi: 10.15585/mmwr.mm6940e1
81. Godfred-Cato S, Bryant B, Leung J, Oster ME, Conklin L, Abrams J, et al. COVID-19–Associated Multisystem Inflammatory Syndrome in Children — United States, March–July 2020. Morb Mortal Wkly Rep. (2020) 69:1074–80. doi: 10.15585/mmwr.mm6932e2
82. Belot A, Antona D, Renolleau S, Javouhey E, Hentgen V, Angoulvant F, et al. SARS-CoV-2-related paediatric inflammatory multisystem syndrome, an epidemiological study, France, 1 March to 17 May (2020. Eurosurveillance. (2020) 25:2001010. doi: 10.2807/1560-7917.ES.2020.25.22.2001010
83. Whittaker E, Bamford A, Kenny J, Kaforou M, Jones CE, Shah P, et al. Clinical characteristics of 58 children with a pediatric inflammatory multisystem syndrome temporally associated with SARS-CoV-2. JAMA. (2020) 324:259. doi: 10.1001/jama.2020.10369
84. Rodríguez-Molinero A, Gálvez-Barrón C, Miñarro A, Macho O, López GF, Robles MT, et al. Association between COVID-19 prognosis and disease presentation, comorbidities and chronic treatment of hospitalized patients. PLoS ONE. (2020) 15:e0239571. doi: 10.1371/journal.pone.0239571
Keywords: SARS-CoV-2, Covid-19, symptomatology, clinical presentation, signs and symptoms, clinical features, coronavirus
Citation: Mehta OP, Bhandari P, Raut A, Kacimi SEO and Huy NT (2021) Coronavirus Disease (COVID-19): Comprehensive Review of Clinical Presentation. Front. Public Health 8:582932. doi: 10.3389/fpubh.2020.582932
Received: 13 July 2020; Accepted: 15 December 2020; Published: 15 January 2021.
Reviewed by:
Copyright © 2021 Mehta, Bhandari, Raut, Kacimi and Huy. This is an open-access article distributed under the terms of the Creative Commons Attribution License (CC BY) . The use, distribution or reproduction in other forums is permitted, provided the original author(s) and the copyright owner(s) are credited and that the original publication in this journal is cited, in accordance with accepted academic practice. No use, distribution or reproduction is permitted which does not comply with these terms.
*Correspondence: Nguyen Tien Huy, tienhuy@nagasaki-u.ac.jp
Disclaimer: All claims expressed in this article are solely those of the authors and do not necessarily represent those of their affiliated organizations, or those of the publisher, the editors and the reviewers. Any product that may be evaluated in this article or claim that may be made by its manufacturer is not guaranteed or endorsed by the publisher.
Thank you for visiting nature.com. You are using a browser version with limited support for CSS. To obtain the best experience, we recommend you use a more up to date browser (or turn off compatibility mode in Internet Explorer). In the meantime, to ensure continued support, we are displaying the site without styles and JavaScript.
- View all journals
- Explore content
- About the journal
- Publish with us
- Sign up for alerts
- NATURE PODCAST
- 17 December 2020
Coronapod: The big COVID research papers of 2020
- Benjamin Thompson ,
- Noah Baker &
- Traci Watson
You can also search for this author in PubMed Google Scholar
Benjamin Thompson, Noah Baker and Traci Watson discuss some of 2020's most significant coronavirus research papers.
In the final Coronapod of 2020, we dive into the scientific literature to reflect on the COVID-19 pandemic. Researchers have discovered so much about SARS-CoV-2 – information that has been vital for public health responses and the rapid development of effective vaccines. But we also look forward to 2021, and the critical questions that remain to be answered about the pandemic.
Papers discussed
A Novel Coronavirus from Patients with Pneumonia in China, 2019 - New England Journal of Medicine, 24 January
Clinical features of patients infected with 2019 novel coronavirus in Wuhan, China - The Lancet , 24 January
A pneumonia outbreak associated with a new coronavirus of probable bat origin - Nature , 3 February
A new coronavirus associated with human respiratory disease in China - Nature , 3 February
Temporal dynamics in viral shedding and transmissibility of COVID-19 - Nature Medicine , 15 April
Spread of SARS-CoV-2 in the Icelandic Population - New England Journal of Medicine , 11 June
High SARS-CoV-2 Attack Rate Following Exposure at a Choir Practice — Skagit County, Washington, March 2020 - Morbidity & Mortality Weekly Report , 15 August
Respiratory virus shedding in exhaled breath and efficacy of face masks - Nature Medicine , 3 April
Aerosol and Surface Stability of SARS-CoV-2 as Compared with SARS-CoV-1 - New England Journal of Medicine , 13 April
Projecting the transmission dynamics of SARS-CoV-2 through the postpandemic period - Science , 22 May
Estimating the effects of non-pharmaceutical interventions on COVID-19 in Europe - Nature, 8 June
The effect of large-scale anti-contagion policies on the COVID-19 pandemic - Nature , 8 June
Retraction—Hydroxychloroquine or chloroquine with or without a macrolide for treatment of COVID-19: a multinational registry analysis - The Lancet, 20 June
A Randomized Trial of Hydroxychloroquine as Postexposure Prophylaxis for Covid-19 - New England Journal of Medicine , 3 June
Association Between Administration of Systemic Corticosteroids and Mortality Among Critically Ill Patients With COVID-19 - JAMA , 2 September
Immunological memory to SARS-CoV-2 assessed for greater than six months after infection - bioRxiv, 16 November
Coronavirus Disease 2019 (COVID-19) Re-infection by a Phylogenetically Distinct Severe Acute Respiratory Syndrome Coronavirus 2 Strain Confirmed by Whole Genome Sequencing - Clinical Infectious Diseases , 25 August
Nature’s COVID research updates – summarising key coronavirus papers as they appear
Never miss an episode: Subscribe to the Nature Podcast on Apple Podcasts , Google Podcasts , Spotify or your favourite podcast app. Head here for the Nature Podcast RSS feed .
doi: https://doi.org/10.1038/d41586-020-03609-2
Related Articles
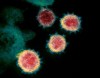
- Public health
Trials that infected people with common colds can inform today’s COVID-19 challenge trials
Correspondence 21 MAY 24
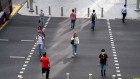
A global pandemic treaty is in sight: don’t scupper it
Editorial 21 MAY 24
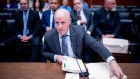
US halts funding to controversial virus-hunting group: what researchers think
News 16 MAY 24
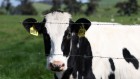
Could bird flu in cows lead to a human outbreak? Slow response worries scientists
News 17 MAY 24
Internet use and teen mental health: it’s about more than just screen time
Social-media influence on teen mental health goes beyond just cause and effect
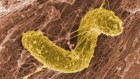
Gut microbes linked to fatty diet drive tumour growth
Vice President, Nature Communications Portfolio
This is an exciting opportunity to play a key leadership role in the market-leading journal Nature Portfolio and help drive its overall contribution.
New York City, New York (US), Berlin, or Heidelberg
Springer Nature Ltd
Senior Postdoctoral Research Fellow
Senior Postdoctoral Research Fellow required to lead exciting projects in Cancer Cell Cycle Biology and Cancer Epigenetics.
Melbourne University, Melbourne (AU)
University of Melbourne & Peter MacCallum Cancer Centre
Overseas Talent, Embarking on a New Journey Together at Tianjin University
We cordially invite outstanding young individuals from overseas to apply for the Excellent Young Scientists Fund Program (Overseas).
Tianjin, China
Tianjin University (TJU)
Chair Professor Positions in the School of Pharmaceutical Science and Technology
SPST seeks top Faculty scholars in Pharmaceutical Sciences.
Chair Professor Positions in the School of Precision Instruments and Optoelectronic Engineering
We are committed to accomplishing the mission of achieving a world-top-class engineering school.
Sign up for the Nature Briefing newsletter — what matters in science, free to your inbox daily.
Quick links
- Explore articles by subject
- Guide to authors
- Editorial policies
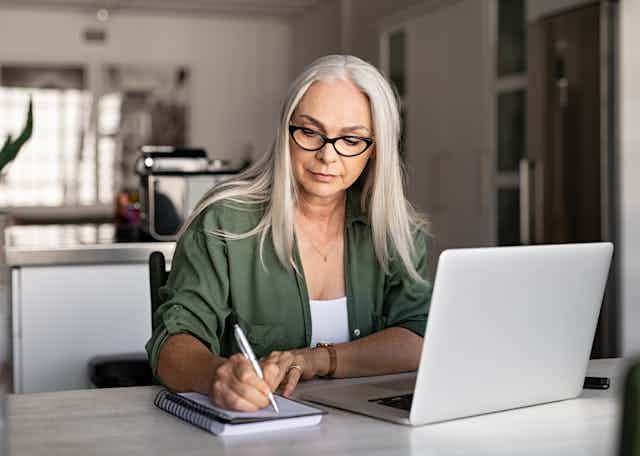
Confused about COVID? Here’s how to read a research paper
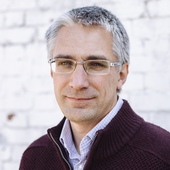
Senior Lecturer in Evidence-Based Healthcare and University Ethics Advisor, University of Portsmouth
Disclosure statement
Simon Kolstoe does not work for, consult, own shares in or receive funding from any company or organisation that would benefit from this article, and has disclosed no relevant affiliations beyond their academic appointment.
University of Portsmouth provides funding as a member of The Conversation UK.
View all partners
Scientific evidence can be difficult to understand. Normally we can rely on experts to interpret it for us, or the media to accurately report any interesting new discoveries, but the pandemic has challenged this.
Almost daily we are faced with contradictory views claiming to be “based on the scientific evidence”. But if you’re not an academic, how can you go about checking the evidence for yourself?
Scientific research is communicated in the form of “research papers” published in professional journals. To ensure accuracy, each paper is carefully checked by both editors and outside academic experts in a process called “peer review”. Although peer review is not perfect , it does tend to ensure articles are more reliable compared with those produced in other types of publishing .
Therefore, to judge the scientific evidence for yourself, you need to read and understand peer-reviewed papers. This can be daunting, but if you approach research papers with the right strategy they can be easier to digest.
1. Find the research paper
Following the publication of new research, the results are often summarised by the media. Frustratingly, these summaries seldom provide a link to the original peer-reviewed paper itself.
To find the original paper, one good strategy is to track down the original press release from the university or company releasing the research. You can also use an academic search engine like Google scholar or PubMed to search for recent papers published by the authors, who are normally (although not always) named by journalists.
Historically readers have had to pay to read academic papers, but increasingly research papers are free to readers through “ open access ” arrangements. Unfortunately, if a paper is not open access, there is not much you can do to read it without paying a fee to the publisher.
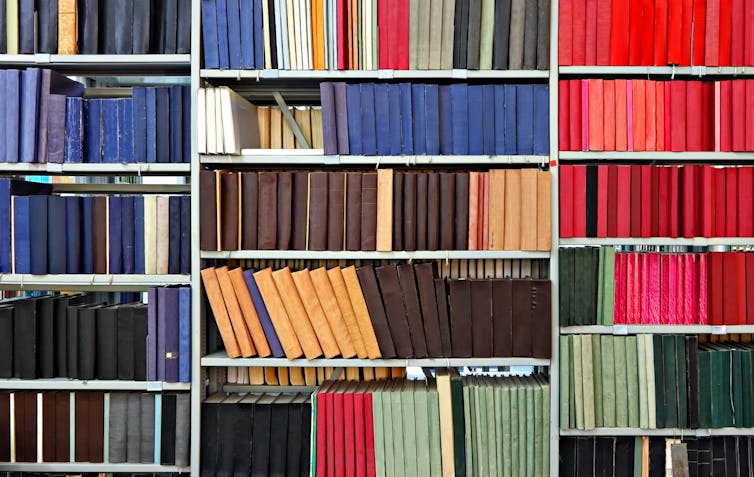
2. Read the abstract and look at the pictures
Research papers are long and dense with a very different structure compared with articles in the normal media. Media articles start with the most important information in the first few lines and then add background or contextual information as the article progresses.
Research papers start off with an introduction describing the background, then sections describing the methods and results, a discussion (highlighting strengths and weaknesses of the research), and finally the conclusion – often only in the very last few sentences. However, to help speed up reading, a summary or “abstract” is always provided at the beginning.
The abstract is the best place to start (and is almost always available for free). If you are not an expert in the subject area, make sure you look up any words you do not understand, because everything mentioned in the abstract will be key to understanding the paper as a whole.
After reading the abstract you may find you have gathered all the information you need about the research, but if after reading it you still would like to find out more, have a quick look at the pictures, figures and diagrams (if available) to get a better idea of the experiments being reported.
3. Determine how good the journal is and who wrote the paper
After reading the abstract I normally look at who the authors are, what university or company they work for, and how good the journal publishing the paper is.
Academics with a track record of producing high-quality research are a good sign. The first and last authors listed in research papers are often the most important , so look them up to see what else they have produced.
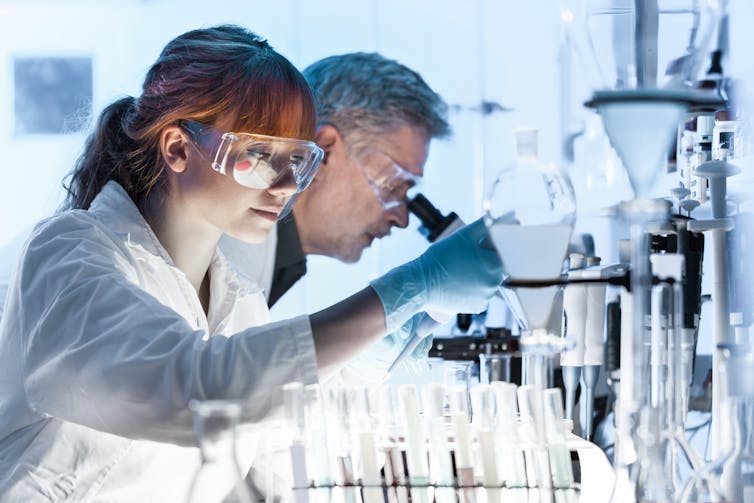
Having the research published in a good journal is also important, because the better journals are able to access more experienced peer reviewers and editors. Here the “impact factor” of a journal is often quoted, which relates to how many other researchers refer to the papers published in it.
However, in recent years impact factors have been strongly criticised as a way of judging journals, even though it’s still true that the best research is published in a fairly small number of journals. One alternative to relying on the impact factor is to simply look up the journal title online to see what researchers say about it. As researchers spend a lot of time discussing which journals are best, this should allow you to find out fairly quickly whether the journal you’re looking at is a reputable one.
4. Read the discussion
If you have got this far you are probably convinced that the research paper is interesting and worth a bit more effort to read. So next, find the part of the paper that discusses the results (often called the discussion) and read through this carefully, flicking back to the methods or results sections if you need to understand in more detail how the experiments were done. Again, look up any terms you do not understand.
5. Read the introduction and check out some of the references
Once you have a good idea of what the paper is reporting, finish off by reading the introduction – this normally provides an overview of why the experiments were conducted in the first place. You should now have a very good idea of what the paper is reporting and some of the wider context.
If you are particularly interested in the topic, look too at some of the key references that the paper quotes. If the paper isn’t brand new, go back to an academic search engine to see whether others have since referenced (or cited) it, and what they are saying about the research.
6. When a paper is not a paper
A word of warning: not every article published in a journal reports new research. Journals also contain news articles, opinion pieces and reviews. These are seldom peer reviewed, and although still written for a professional audience, are not considered primary research.
Another thing to watch out for are versions of research papers that are made available online in advance of being checked by peer reviewers, in a form called “preprints”. Preprints can be very useful for finding out about new results quickly because the peer review and journal publication process can take up to a year. This has been necessary during the pandemic, for example. These preprints are normally clearly labelled, just as a warning that the information in them should not be relied upon in the same way as a full, peer-reviewed research paper.
- Coronavirus
- Academic research
- Coronavirus insights

Content Coordinator

Lecturer / Senior Lecturer - Marketing

Assistant Editor - 1 year cadetship

Executive Dean, Faculty of Health

Lecturer/Senior Lecturer, Earth System Science (School of Science)
Click through the PLOS taxonomy to find articles in your field.
For more information about PLOS Subject Areas, click here .
Loading metrics
Open Access
Peer-reviewed
Research Article

One-year in: COVID-19 research at the international level in CORD-19 data
Roles Conceptualization, Data curation, Formal analysis, Project administration, Writing – original draft
* E-mail: [email protected]
Affiliation John Glenn College of Public Affairs, The Ohio State University, Columbus, Ohio, United States of America

Roles Data curation, Formal analysis, Investigation, Methodology, Writing – review & editing
Affiliation School of Public Affairs, Zhejiang University, Hangzhou, Zhejiang, China
Roles Conceptualization, Formal analysis, Software, Validation, Visualization
Affiliation Australian Artificial Intelligence Institute, University of Technology Sydney, Ultimo, Australia
Roles Conceptualization, Data curation, Formal analysis, Methodology, Writing – original draft, Writing – review & editing
Affiliation Shidler College of Business, University of Hawaiʻi at Mānoa, Honolulu, Hawaiʻi, United States of America
- Caroline S. Wagner,
- Xiaojing Cai,
- Yi Zhang,
- Caroline V. Fry
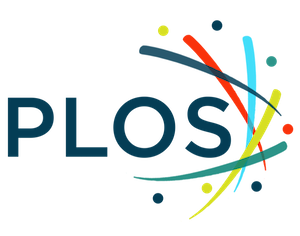
- Published: May 25, 2022
- https://doi.org/10.1371/journal.pone.0261624
- Peer Review
- Reader Comments
The appearance of a novel coronavirus in late 2019 radically changed the community of researchers working on coronaviruses since the 2002 SARS epidemic. In 2020, coronavirus-related publications grew by 20 times over the previous two years, with 130,000 more researchers publishing on related topics. The United States, the United Kingdom and China led dozens of nations working on coronavirus prior to the pandemic, but leadership consolidated among these three nations in 2020, which collectively accounted for 50% of all papers, garnering well more than 60% of citations. China took an early lead on COVID-19 research, but dropped rapidly in production and international participation through the year. Europe showed an opposite pattern, beginning slowly in publications but growing in contributions during the year. The share of internationally collaborative publications dropped from pre-pandemic rates; single-authored publications grew. For all nations, including China, the number of publications about COVID track closely with the outbreak of COVID-19 cases. Lower-income nations participate very little in COVID-19 research in 2020. Topic maps of internationally collaborative work show the rise of patient care and public health clusters—two topics that were largely absent from coronavirus research in the two years prior to 2020. Findings are consistent with global science as a self-organizing system operating on a reputation-based dynamic.
Citation: Wagner CS, Cai X, Zhang Y, Fry CV (2022) One-year in: COVID-19 research at the international level in CORD-19 data. PLoS ONE 17(5): e0261624. https://doi.org/10.1371/journal.pone.0261624
Editor: Alberto Baccini, University of Siena, Italy, ITALY
Received: July 14, 2021; Accepted: December 6, 2021; Published: May 25, 2022
Copyright: © 2022 Wagner et al. This is an open access article distributed under the terms of the Creative Commons Attribution License , which permits unrestricted use, distribution, and reproduction in any medium, provided the original author and source are credited.
Data Availability: https://doi.org/10.6084/m9.figshare.16620274.v1 .
Funding: The authors received no specific funding for this work.
Competing interests: The authors have declared that no competing interests exist.
Introduction
The COVID-19 pandemic upended many normal practices around the conduct of research and development (R&D); the extent of disruption is revealed across measures of scientific research output [ 1 – 3 ]. This paper revisits the extent to which patterns of international collaboration in coronavirus research during the COVID-19 pandemic depart from ‘normal’ times. We present publication patterns using one full year of publications data from the CORD-19 database, and observations on non-COVID peer-reviewed publications using the Web of Science, to examine national and international publication rates and network patterns. We examine topics of research on COVID-19, and reflect on lessons learned about international collaboration from the disruption. The analysis may be useful to research administrators, international affairs professionals and science studies scholars.
We study the international collaborative linkages as a network. In the absence of a global governing body, international collaborations operate by network dynamics. Scientific connections at the global level reflect collective decisions of hundreds of individuals who seek to connect to each other. Connections are not random; they are influenced by five factors: two personal and three contextual. Personal choices tend towards those previously known or known by reputation or introduction. Contextual choices are 1) resources available, 2) geopolitical factors, and 3) time and attention. Network dynamics emerge from interplay of these factors, although there is little research on how a disaster, such as a pandemic, will affect productivity, collaboration, and topic focus. Moreover, it is difficult to determine expectations of network dynamics in a pandemic because global exogenous disruptions are rare and studies about science in a disaster are sparse. This paper seeks to fill some of these gaps.
This paper is organized to describe the literature supporting our inquiries, and to present hypotheses derived from the literature. We then describe coronavirus research prior to the pandemic, and early policy responses to the crisis. A section on data and methodology presents approaches designed to answer the questions emerging from the hypotheses. A results section describes outcomes of the analyses, followed by limitations of the data and approaches presented here. A discussion section details responses to the hypotheses as well as observations about the research project and avenues for further research. An S1 Appendix provides additional technical details.
Literature review and hypotheses
In the decades preceding the pandemic, R&D spending and output grew rapidly. OECD data shows that, among member nations, R&D spending was 25% higher in 2017 than a decade earlier. The US National Science Foundation (NSF) reports that from 2008–2018 the annual number of citable publications (articles, notes and letters, hereafter, “publications”) worldwide grew by 3.83% per year from 1.8 million to 2.6 million [ 4 ]. Increases in spending, trained practitioners, and publications contributed to an overall growth of the research enterprise in natural sciences and engineering, social sciences, and arts and humanities. Within the research enterprise, among scientifically advanced nations, international collaborative publications grew at a faster rate than national publications, accounting for as much as one-quarter of all publications in 2018, with variations observed across fields, according to the National Science Foundation [ 4 ]. Those fields that rely on large-scale equipment are more highly globalized, but increases in international linkages is observed in most fields, tied, not to funding or equipment, but to the interests of researchers to work together. The size of these teams has grown larger over time [ 5 ].
International collaborative patterns have been dominated by scientifically advanced nations, although, over time, many low-income, emerging and developing nations have become more active, and have partnered with more advanced nations [ 6 ]. Some tendency to collaborate among nations with former colonial ties is observed [ 7 ], but this is likely due to incentivized funding provided by the former colonial power. Political differences do not appear to hinder collaboration, evidenced most notably by the rise of China to be the number one collaborating nation with the United States. Abramo et al. [ 8 ] added to literature on tendency of neighbors to work together, but Choi [ 6 ] shows this tendency to be decreasing over time.
Prior research into collaboration around viral disease events found that, during the 2014 West African Ebola epidemic, collaboration grew between scientists from scientifically advanced nations and the most affected nations [ 9 ], suggesting that connections were made based upon disease location. Ebola outbreaks brought in researchers from scientifically advanced nations to work with local researchers on specific events. Collaborative ties did not persist past the disease event.
A global community of coronavirus researchers predated the advent of the 2019 novel coronavirus; this community formed after the 2002 SARS coronavirus epidemic [ 1 ]. As the new threat emerged in 2019, governments provided emergency R&D funding to encourage targeted research on the novel coronavirus. Most of these funds were committed by governments in scientifically advanced countries and were allocated to national institutions, although the European Union (EU) and the US National Institutes of Health (NIH) fund both national and foreign applicants. National actors receiving funds may then choose, in some instances, to connect to foreign collaborators, creating an international connection. The resulting connections can be studied through coauthorship attributions on paper and interpreted as a self-organizing network of connectivity [ 10 ]. In Fry et al. [ 1 ], we showed that, during the early months of the COVID-19 pandemic, international collaborations in coronavirus research emerged among just a few nations, and, on average, publications had fewer coauthors per paper than pre-pandemic levels. Most nations did not publish on the novel coronavirus in early pandemic research.
We expect that, as funds became available to researchers, and as more knowledge was generated through the first year of the pandemic, cross-national collaborative ties will grow. That said, because of travel limitations and a need for urgent results, we expect the rate of international collaboration and network ties to remain lower than pre-pandemic levels. This expectation is also informed by the research of Rotolo and Frickel [ 11 ] who found that there were fewer ties and smaller teams among researchers just after a hurricane disaster. Further, based upon findings in the wake of the Fukushima disaster [ 12 ] and a survey by Myers et al. [ 2 ] we expect that attention to pandemic-related R&D (including basic science, patient care, and public health) has lessened the output of other scientific research as well as reduced the rate of international collaborations in other fields. In addition to changed collaborative patterns, we expect to see changes in topics throughout the first year of the pandemic with topics becoming more focused as knowledge about events grows, which we explore in a separate article. In Zhang et al. [ 3 ], we showed that, at the beginning of the pandemic, the disrupted knowledge system exhibited very little topic focus. As the pandemic progresses, we expect to see greater topic focus. We further expect to see international collaboration focus on basic science and less on patient care and public health which may have a local, regional, or national characteristics. We expect continued consolidation among leading nations and elite institutions through the pandemic year due to pressures for rapid results and the lack of mobility to begin new collaborations. Further, we expect that geographic distance will mean less during the pandemic because remote collaborators will rely on communications technologies rather than face-to-face consultations.
Science during the COVID-19 pandemic
Coronavirus research predated the COVID-19 crisis, but it was a community of 22,000 researchers working on SARs, MERs, and the porcine diarrhea epidemic [ 1 , 3 ]. Coronavirus research output doubled in number over the decade between 2008–2018, in keeping with numbers in the biological sciences. As the new threat of a novel coronavirus emerged in 2019, governments provided emergency R&D funding to encourage targeted research, which attracted many new researchers from a wide range of fields. More than 156,000 researchers published on COVID-19 in 2020, growing the original community that had worked on coronaviruses by over 130,000.
The United States Government committed the largest amount of funds to the novel coronavirus, through the CARES Act and other legislation, allocating at least $5 billion to basic research, applied research, and development of vaccines, diagnostics, mapping of disease occurrence, analytics, public health, and medicine. The bulk of funds were appropriated by the US Congress to the U.S. National Institutes of Health (NIH), and through them to BARDA, the Biomedical Advanced Research and Development Authority. Other agencies also received additional R&D funds over and above their annual appropriations, including the National Science Foundation ($74 million) and the Department of Energy ($99.5 million). The U.S. government also provided funds to private companies to aid in vaccine development and procurement. For example, Moderna, a pharmaceutical company headquartered in Massachusetts, received $1 billion of R&D funds and in $1.5 billion in advanced purchase agreements.
Germany provided $891 million in R&D funds into coronavirus as well as to vaccine development. The European Commission provided €469 million in R&D funds, along with permission to recipients to reallocate funds originally slotted for other topics. The UK government reports spending £554 million on 3,600 initiatives related to COVID-19. In China, the Ministry of Science and Technology invested $100 million for emergency projects and unknown millions of funds for vaccine development, and the National Natural Science Foundation of China also reallocated approximately $15 million for projects related to COVID-19.
Many research organizations and researchers from various disciplines shifted to focus on aspects of the pandemic and received grant funds to do so. Just as with any other R&D funding, the expectation is that funded research will result in published works, enhanced equipment, and medicines and vaccines. Very early in the pandemic, preprints [ 13 ] (non-peer-reviewed articles) and peer reviewed articles began flooding into publishing venues. The number of scholarly publications related to the crisis grew spectacularly in the early months of the pandemic [ 1 ].
The rush to publish is expected: Zhang et al. [ 14 ] note that historical patterns show that researchers have, in previous cases, responded quickly to public health emergencies with publications, which is the same pattern we see with COVID-19 research. In updating our earlier work [ 15 ], we found that the number of coronavirus publications in CORD-19 grew considerably in the early days of the novel coronavirus, rising at a spectacular rate from a total of 4,875 articles produced on the topic (preprint and peer reviewed) between January and mid-April to an overall sum of 44,013 by mid-July, and accumulated to 87,515 by the start of October 2020. (In comparison, nanoscale science was a rapidly growing field in the 1990s, but it took more than 19 years to go from 4,000 to 90,000 articles [ 16 ]).
The dissemination of publications changed during the pandemic. COVID-19 peer-reviewed and edited publications became available to other researchers through new (CORD-19) and pre-existing (National Library of Medicine) web platforms. COVID-19 publications were much more likely than other works to be published as open access in 2020 [ 17 ]. In 2020, open-access, peer-reviewed publications related to COVID-19 accounted for 76.6% of all publications compared to 51% of all non-COVID publications. Highly cited papers—those in the top 1% most highly cited, with over 500 citations—were more likely than other work to be published in subscription-based journals such as The Lancet , Science , New England Journal of Medicine or Nature but these works were placed into open Web portals for rapid access. The National Library of Medicine served as a repository for most new publications related to COVID-19. The publishing house Elsevier—which publishes many subscription-based journals—created a "Public Health Emergency Collection" to make COVID-19 articles rapidly available regardless of the access status of the original work (subscription or open access). Similarly, CORD-19 (the database which provided data for this article) through Semantic Scholar, made relevant research (including historical work) rapidly and readily available and allowed researchers to deposit work they viewed as relevant.
Researchers from China and the USA increased the rate of collaborative publications on coronavirus in the earliest days of the pandemic Fry et al. [ 1 ]. Liu et al. [ 18 ] showed a surge of what they call ‘parachuting collaborations’–new connections not seen prior to the pandemic–which dramatically increased during the pandemic. Together with the findings in Fry et al. [ 1 ], these findings suggests that search and team formation changed to adapt to the needs of COVID-19 research, a finding also reported by Lee & Haupt [ 19 ]. Liu et al. [ 18 ] found that COVID-19 research papers were less likely to involve international collaboration than non-COVID-19 papers during the same time period, a finding reported by Aviv-Reuven & Rosenfeld [ 20 ] as well, a finding we can confirm.
Several research articles note the absence of emerging and developing nations in early COVID-19 research. Fry et al. [ 1 ] and Lee & Haupt [ 19 ] showed that very few developing nations were involved in the earliest day of the crisis. Zhang et al. [ 3 ] confirmed Fry et al. in finding that the USA, China, and the UK were the three countries with the largest number of articles by mid-year. Several articles report that fewer coauthors appear on article bylines [ 1 , 20]. This is likely due to the need for rapidity in responding to the crisis: fewer coauthors reduces the time needed to communicate, synthesize and submit results.
Data and methodology
Data for this study were extracted in March 2021 from the Covid-19 Open Research Dataset, “CORD-19,” an open resource of scientific papers on COVID-19 and related historical coronavirus research. CORD-19 is designed to facilitate the development of text mining and information retrieval systems for COVID-19 research over its rich collection of metadata and structured full-text papers. It is accessible through the National Library of Medicine, National Institutes of Health, USA. In addition, we accessed the whole of Scopus 2020 data to examine non-COVID publications over the year. To search for evidence of government funding for COVID-19 research, we searched Web of Science, which has a field for funding acknowledgements. (Non-COVID publications were any peer-reviewed, published work that did not include one of the keywords for the COVID search below).
To maintain consistency across our studies, we applied the same search terms as used in Fry et al. [ 1 ], Cai et al. [ 15 ], and Zhang et al. [ 3 ] and limited the search to the dates January 2020 to December 2020 and citation data to March 2021. The following search terms were applied to titles and abstracts to obtain an initial dataset of coronavirus publications:
- coronavirus
- corona virus
- Severe Acute Respiratory Syndrome
- Middle East Respiratory Syndrome
The initial dataset was cleaned to remove the following artifacts: conference papers, preprints, collections of abstracts, symposia results, articles pre-dating 2020, and meeting notes. Preprints were excluded in this report to avoid double-counting in cases where a work is subsequently peer-reviewed and published. The author names, institutional affiliation, and addresses were extracted for analysis. For articles derived from the PubMed Central website, the citation count was extracted up to March 2021. The resulting dataset provided us with 106,993 publications for the calendar year 2020. The final dataset was further divided into four quarters, shown in Table 1 , according to “Published Date”, i.e., the electronic publication dates (if any) or else print publication date: January to March (2020 Q1), April to June (2020 Q2), July to September (2020 Q3), and October to December (2020 Q4). Full counting is used to count the number of publications of a specific country or institution. Among all the publications with at least one author and address, 8,158 (8.9%) are single-author articles, with the rest involving coauthors at the national (78%) or international levels, with 20,203 (22.0%).
- PPT PowerPoint slide
- PNG larger image
- TIFF original image
https://doi.org/10.1371/journal.pone.0261624.t001
We analyzed the number of authors and coauthors per paper for descriptive statistics of people publishing on the novel coronavirus; we analyzed keyword usage and topics drawn from keywords and abstracts, and we analyzed geographic location of authors to study cooperative patterns at the international level. We collected additional data to answer questions about activities not available in CORD-19 in funding and on non-COVID research publications. We compared the CORD-19 data to a defined dataset of coronavirus research derived from scientific articles on coronavirus-related research on historical data we had earlier extracted from PubMed, Elsevier’s Scopus, and Web of Science (details of the construction of this data can be found in Fry et al. [ 1 ]). The datasets are available at https://figshare.com/articles/dataset/One_Year_of_COVID-19_Int_l_Collaboration/16620274 . For the CORD-19 articles that are also indexed in Clarivate’s Web of Science (WoS), we retrieved funding information to get a rough view about which funding agency is contributing to the coronavirus research in the pandemic period; 35% of CORD-19 articles acknowledged funding. Dimensions database was used to analyze the open access categories.
To test for change in the number of participants in research groups between pre-COVID-19 and COVID-19 periods, we use double-tailed T-tests to compare the average “team” structure between periods. (We employ the word “team” for convenience to describe coauthor groups even though we do not know the mechanism of cooperation among the group.) Statistical significance is assessed at 0.05 level. Team structure is measured as average number of authors per publication, average number of nations per publication, and the percentage share of internationally collaborated articles. We also use regression models to test the relationship between team structure and citation impact. Since the dependent variable, i.e., citations, is a non-negative integer, we apply count-data regression models (i.e., negative binomial regression) that can account for the nature of the data.
To test the relationship between geographic distance and international collaboration among nations, we calculate the geographic distance and collaboration strength between country pairs. The geographic distance between nations is defined as distance between capitals of each nation, based on geographic data about world cities in the R package “map”. Following the normalization approach used in previous research [ 6 , 24 , 25 ], we apply Salton’s cosine measure of international collaboration strength, which takes the publication size of nations into account. It is calculated as the number of collaborative publications divided by the square root of the product of the number of publications of the two collaborating nations (See Appendix Table 2 in S1 Appendix ).
The process of collecting and cleaning the CORD-19 database produced a set of 106,993 publications. The set used for this study is limited to work published in 2020, responding to the search string. We compared the CORD-19 results to Elsevier’s Scopus for 2020: the search of Scopus produced 73,000 COVID-19 publications, so fewer than CORD-19. Scopus limits its indexing of publications to specific journals, while CORD-19 encouraged open deposit of materials, which would include venues not indexed by Scopus—this likely accounts for the differences in numbers among databases.
For all research in 2020, Scopus shows a total of 2,584,701 publications, COVID and non-COVID topics (recall that non-COVID topics are not included in CORD-19). Against expectations, growth in life and health sciences output between 2019 and 2020 is shown in most disciplines of life and health sciences fields, both COVID and other topics. The number of publications on novel coronavirus and resulting disease is about 20 times higher in 2020 than coronavirus research published between 2018 and 2019, when work focused on SARS and MERS—earlier disease events that were not as devastating as COVID-19.
Fig 1 shows the number of coronavirus publications by month compared to the number of reported disease case outbreaks worldwide. Publication numbers grew quickly between February and May 2020 at the same time as the number of COVID-19 cases increased at an alarming rate. Since May 2020, the number of publications has remained stable at over 10,000 publications per month, while the rate of growth in COVID-19 cases declined slightly relative to the earliest months. The surge of publications on COVID-19 clearly result from thousands of ‘new’ researchers from various fields publishing on coronavirus in 2020. In pre-COVID-19 period, coronavirus researchers were drawn mainly from Life Sciences & Biomedical Sciences (e.g., Virology, Infectious Disease) and Natural Sciences (e.g., Multidisciplinary Chemistry and Organic Chemistry). The pandemic calls upon researchers from all research fields, with noticeable increased efforts from Social Sciences (including the authors of this work).
Data on publications and cases are collected from CORD-19 and WHO ( https://covid19.who.int/ ).
https://doi.org/10.1371/journal.pone.0261624.g001
Table 2 shows the top 25 life sciences fields in 2020 from Scopus, with the total number of COVID-19 and non-COVID articles in the same field. Fields that show the highest number of COVID-19 research are medicine, infectious disease, and public health. For all research in life and health sciences, highest growth is seen in surgery, plant science, and psychiatry and mental health. We can assume that the COVID-19 articles were written in 2020, since they are topical—“COVID” was not a keyword in 2019. Moreover, journal editors greatly sped up the processing time for COVID-related review and publication [ 26 , 27 ]. Conversely, the non-COVID articles may represent work conducted years prior, since it takes time to write, review and publish research results [ 28 ]. In fact, peer review in non-COVID related disciplines was delayed in 2020 due to the pandemic [ 26 ] so there may be insufficient time to fully assess the impact of the crisis on non-COVID research of publication output. Data in 2021 will be more telling of the impact of the pandemic year on non-COVID research publication patterns.
Data: Elsevier’s Scopus.
https://doi.org/10.1371/journal.pone.0261624.t002
Contributions by author location
COVID-19 publishing numbers differ considerably by regions of the world. Fig 2 shows regionally aggregated contributions on COVID-19. Asian countries contributed over one-third of world publications in early 2020, but this percentage share dropped in the later months of 2020 as China reduced its output. Europe showed the opposite trend: Europe’s share of world COVID publications increased since April 2020 and the number remained stable through the latter months of 2020. North America’s share of publications increased throughout 2020.
https://doi.org/10.1371/journal.pone.0261624.g002
As expected, scientifically advanced nations, including China, account for the majority of COVID-19 publications. Among all nations publishing related work, USA, China, and UK produced (together and separately) 50% of the coronavirus articles during 2020, shown in Table 3 . As of early 2021, their publications accumulated around 68% of citations made to global publications supporting the expectation of consolidation around expertise and reputation. In the earliest days of the pandemic, three articles from Chinese authors [ 29 – 31 ] contributed key findings that guided much of the ensuing research; each of these articles garnered thousands of citations. Italy, UK, India, and Spain were slower to begin publishing but became more prolific through 2020, and particularly so in the final quarter. Fig 3 shows the rapid growth of monthly publications for selected countries, which also tracks with the trend in national COVID-19 cases; this finding is similar to one found in the 2014 West African Ebola epidemic [ 9 ].
https://doi.org/10.1371/journal.pone.0261624.g003
https://doi.org/10.1371/journal.pone.0261624.t003
International collaborative publication rates in coronavirus research took six months to recover to pre-COVID levels. Collaborative research projects take longer to publish results, so the ‘recovery’ time may simply reflect more communication and production time needed due to the physical distances and time zone differences. As expected, during 2020, international collaborative papers showed fewer nations per paper in the early days, but this number increased through the year. This number stabilizes in the fourth quarter to pre-pandemic levels. Supporting other findings [ 18 ], about 65% of internationally coauthored papers include only two nations—this is a drop from usual patterns.
In earlier work, we noted that developing nations were largely absent from the publication records in the early COVID-19 period. We explored the participation of developing nations in global coronavirus research over the full year, expecting to see some recovery, but it was weak. Pre-COVID-19 coronavirus research in 2018–2019 shows that low-income nations [ 32 ] accounted for 26% of all nations participating in the research, publishing 4% of global articles. This drops during 2020: In the first two quarters of 2020, low-income nations accounted for 21% of active nations and produced 3.4% of global articles (Low-income countries (LIS) are defined by the World Bank, https://data.worldbank.org/country/XM . China, India and Brazil are not low-income countries.). That said, throughout 2020 low-income nations increase their contribution to the coronavirus research, contributing just slightly more in number of publications compared to their participation in pre-COVID-19 period, but much lower than scientifically advanced nations. Against expectations and in contrast to the trend before the pandemic and in the first few months of 2020, we find, by mid-year, Chinese institutions no longer appear in the list of top 10 producing institutions, supporting Liu et al. [ 18 ]. For example, the University of Hong Kong and the Chinese Academy of Agricultural Sciences ranked third and fourth in pre-COVID-19 research but dropped down the list in 2020. This drop tracks with the drop in number of COVID-19 cases in China.
Academic institutions worldwide were responsible for the largest share of publications about coronavirus during the pandemic, although private companies participated in research, usually through coauthorship with academic coauthors. We identified a list of 40,287 institutions involved in coronavirus research with the following rules: (1) we retrieved valid institution names with a list of key strings, such as “hospital”, “univers*”, and “instit*”; and (2) we consolidated variations of the same institutions, such as “MIT” and “Massachusetts Institute of Technology”, and “University of Sydney” and “Sydney University”. Fig 4 shows the institutions making top contributions to COVID-19 cooperation. As expected, the figure shows that highly reputed institutions—Harvard University (Massachusetts, USA), Huazhong University of Science and Technology (Wuhan, China), and the University of California System—produced the largest absolute numbers of publications on coronavirus in 2020. From the CORD-19 data, we find there are 2,232 articles (2.43%) involving authors from private corporations, which is average for corporate participation in Web of Science [ 33 ]. Nevertheless, this percentage is a drop from private sector participation in pre-COVID-19 dataset, where we found that 3.4% of articles involved the private sector, so it was higher than average and dropped to average in 2020.
The percentage shares of publications in global articles of top 10 prolific institutions in COVID-19 (2020) and pre-COVID-19 (2018–2019) are shown.
https://doi.org/10.1371/journal.pone.0261624.g004
Table 4 shows the most frequently acknowledged funding agencies in coronavirus research indexed in Web of Science in 2020. Funders from the USA, China, and UK (or Europe) are the most commonly acknowledged, which is consistent with the publication outputs as shown in Table 3 . The US National Institutes of Health (NIH), the largest scientific organization dedicated to health and medical research, tops the list and is acknowledged in 15.8% of funded articles. The dominant funder in China, National Natural Science Foundation of China (NSFC), ranks second and contributes to 10.4% of publications, despite decreased publication shares in later periods. European funding agencies, including European Commission, UK Research & Innovation (UKRI), and Medical Research Council UK (MRC), also play a vital role as COVID-19 cases and number of publications increase in Europe.
https://doi.org/10.1371/journal.pone.0261624.t004
Co-occurrence network on coronavirus research
Terms retrieved from titles and abstracts of research articles provide useable clues to understand topic focus (Data for network analysis is available at Wagner, Caroline (2021): Figshare_Network files.rar. figshare. Dataset. https://doi.org/10.6084/m9.figshare.16652752.v1 ). The co-occurrence between terms and entities (e.g., funding agencies), and among terms, reveals their semantic connections in research, and may answer questions such as which agencies support which topics. The connection is measured by how many times two terms appear in proximity in the entire dataset, within and across articles. We identified 4,865 terms from a raw set of 1.2 million terms retrieved from titles and abstracts of the 106,993 articles published in 2020 via natural language processing techniques and a term clumping process [ 34 ], with the aid of VantagePoint (VantagePoint is a text mining tool for analyzing bibliometric data. See the link: https://www.thevantagepoint.com/ ). Specifically, the term clumping process facilitated a set of thesauri to remove meaningless terms (e.g., conjunctions, pronouns, and prepositions) and common terms in academic articles (e.g., “method” and “conclusion”), and it then consolidated terms with the same stem (e.g., terms in singular and plural forms).
Fig 5 analyzes the co-occurrence between the top 20 high-frequency terms and the major funding sources, visualized by Circos [ 35 ]. The Chinese agency, National Science Foundation of China (NSFC), was much more likely to fund research related to “Wuhan” while the United States’ National Institutes of Health (NIH) is much more likely to fund research with the term “United States.” Small differences can be observed for “clinical characteristics” (proportionately more from NSFC), and “hospitalization” (proportionately more from NIH) but these two terms are quite similar, so differences may be due to semantics only. Aside from these two differences, it appears that each of the agencies fund similar term portfolios differentiated only in proportion to their contribution, so more focused on basic research, which was our expectation.
https://doi.org/10.1371/journal.pone.0261624.g005
To assess whether international collaborations had different topic focus from domestic collaborations—which we expected—we analyzed co-terms at both levels. Fig 6 shows the co-term network for the internationally collaborative research, and Fig 7 shows the co-term network for domestic-only collaborations. In both networks, one sees the topics that are shown in Fig 5 as focus areas for government funders. With data extracted using Vantagepoint’s Natural Language Processing function ported into VOSViewer, Fig 6 shows international collaboration dominated by three clusters: 1) research on the virus (red, bottom left), 2) on patient care (purple, top right), and 3) on public health (green, bottom left).
Interactive version accessible at https://app.vosviewer.com/?json=https://drive.google.com/uc?id=1MVWE1bsTGi6jJeeU7BNCcjKTd2yjTiv0 .
https://doi.org/10.1371/journal.pone.0261624.g006
Interactive version accessible at https://app.vosviewer.com/?json=https://drive.google.com/uc?id=1RraBpIYbLY5_DfOMC0YJ7IZoq3_sRiKa .
https://doi.org/10.1371/journal.pone.0261624.g007
Fig 7 shows domestic topics, highlighting greater emphasis on patient care and disease characteristics (gold, top center) than seen in Fig 6 . Moreover, a fourth cluster emerges (blue, center) with details about outbreaks, effects, viral loads, and other aspects of health are seen that are not as prevalent at the international level. A table in the S1 Appendix provides more details about the topics. As expected, the domestic publications focus on public health and patient care more than is seen at the international level, where basic science dominates the topics.
Collaboration rate
Table 5 compares collaboration rates in coronavirus publications before the COVID-19 pandemic and in four quarters of 2020. As expected, and in comparison to the number of co-authors in pre-COVID-19 coronavirus research, publications in 2020 show fewer authors, fewer nations per paper, and less frequent international collaboration overall. Team size—represented by the number of authors on a publication—shrank shortly after the outbreak of COVID-19, a finding we highlighted in Fry et al. [ 1 ], but by the end of the year, the number had recovered and risen to just above levels seen in pre-COVID-19 coronavirus research. As expected, the average number of nations per international publication remained at lower levels than pre-COVID-19 levels for USA articles; there was no significant difference in numbers of international partners for Chinese articles in pre-COVID-19 and COVID-19 periods.
https://doi.org/10.1371/journal.pone.0261624.t005
We explored the relationship between team structure as represented by coauthors on papers and the number of citations to COVID-19 publications for publications produced in 2020 (looking at citation records up until March 2021). Table 5 shows the regression results that explore the relationship between citations to publications and international collaborative team structure, for publications with authors from USA, China, and the UK respectively. As expected, a positive correlation is shown between numbers of citations and international collaboration. Further, also meeting expectations, there is a correlation between number of authors and citations, which may reflect an audience affect due to a larger reader network. Also as expected, international teams attracted more citations than domestic-only teams, again, with a possible audience affect. These findings held for all nations except the USA, where international articles are not cited more than domestic-only research when holding constant the number of authors ( Table 6 , column 6).
https://doi.org/10.1371/journal.pone.0261624.t006
Network analysis
Figs 8 and 9 compare internationally collaborative networks in the pre-COVID-19 (2018–2019) and COVID-19 (2020) periods. Recall that these numbers represent about 22% of all COVID research in 2020. Fig 8 shows pre-COVID coronavirus research with two large clusters: one, a European cluster, and two, a global cluster brokered by the USA. The US collaborated closely with China, the UK, France, the Netherlands, and Germany. The dominance of the USA is partly accounted for by the volume of research when compared to the output per nation; the USA leads in publications, citations, and connectivity in coronavirus research prior to the pandemic and remained the leader during the pandemic.
Interactive version accessible at https://app.vosviewer.com/?json=https://drive.google.com/uc?id=1_1ASbt-FheQE6_VQNc5eFhy578jot9co .
https://doi.org/10.1371/journal.pone.0261624.g008
Interactive version accessible at https://app.vosviewer.com/?json=https://drive.google.com/uc?id=1TLdcW-lNUQ1k0kDlpZYOUQL57E8MKNqM .
https://doi.org/10.1371/journal.pone.0261624.g009
Fig 9 shows the COVID-19 collaborative network, where, as expected based upon research by Rotolo & Frickel [ 11 ], we observe more clusters and more brokering hubs than pre-COVID-19. The number of clusters has grown, with four clusters revealing a broader set of countries acting as centralized nodes or hubs, with the UK, Italy, and Germany increasing their bridging role from positions shown in the pre-COVID-19 network. The European research clusters form into two large groups, one with Italy as the brokering hub and one with the UK in a central brokering hub. Italy intensively links to France, the USA, and Switzerland. African nations join the network through the UK connection. Australia is central to a cluster that includes Spain, Brazil, and many smaller nations from South America. As expected, geographic distance between country pairs is negatively related to the collaboration strength of the two countries in all cases (see Appendix Table 2 in S1 Appendix ). However, during COVID-19 period, the negative impact of geographic distance on collaboration strength was weakened as demonstrated by positive and significant coefficient of interaction of COVID and geographic distance. As expected, physical distance was less of a barrier to collaboration than in other scientific research. The result reveals that the pandemic weakened the role of geographic distance in international collaboration (OLS regression analysis of the relationship between geographic distance and collaboration strength).
Table 7 shows the network metrics for the above networks and for four quarters of 2020. (Visuals of the four quarterly networks are shown in the S1 Appendix ) Consistent with the growing number of internationally collaborative articles throughout 2020, the network statistics reveal expanded connections from the first period (January to March) to the third period, cumulative (July to September) in 2020, but then stabilizing.
https://doi.org/10.1371/journal.pone.0261624.t007
The network statistics suggest that COVID-19 research involved many more participants than those who worked on coronavirus in the years prior to the pandemic, as we find by examining the number of unique author names. From the pre-COVID-19 coronavirus network to the COVID-19 network, we see that number of nodes increases from 103 nations before COVID to 173 during COVID. More importantly, the number of links among nations more than triples, suggesting that many more connections were made at the international level than existed prior to the pandemic. Average degree doubles, supporting the observations of many new links. These links likely were forged remotely in a process that Liu et al. [ 18 ] call “parachuting collaborations” that post-date the pandemic. These types of collaborations may have emerged through friend-of-friend connections, since people could not meet face-to-face due to travel restrictions during 2020. Betweenness centrality drops over the year indicating a shift in influence of the initial, dominant hubs to include more participants from more nations over the pandemic year.
Table 8 shows the network metrics for top producers (USA, UK, and China) in the global network. As shown by the average degree of the three countries, the USA was a hub in the network in both periods, more so early in the pandemic, supporting our expectation of consolidation around expertise and reputation, but betweenness centrality drops as researchers from more countries joined into the research. The UK played a much more active role in the COVID network compared to China in the second half of the year. Despite being a hub in the pre-COVID network and in the first months of the pandemic [ 1 ], China played a less prominent role in the network in 2020.
https://doi.org/10.1371/journal.pone.0261624.t008
Limitations of this research
This research project had a number of limitations of data, time, analysis, and scope. Data limitations include constraints of what is measurable in published work (publications, networks, and citations). We decided to use CORD-19 data because it was the most expansive dataset for COVID-19 research, but we may have picked up lower quality work as a result; it is an open dataset with attendant problems. We extracted desired features, but there may be gaps and errors. In order to get a count of open-access publications, we used Dimensions data, but the total number of COVID-19 publications in Dimensions were lower than CORD-19, so open-access publications are likely under-counted. We present the percentages of COVID-19 research that is open access; these calculations are broadly representative, but they cannot be further verified. Moreover, we are unable to show extent of R&D occurring in private research laboratories if it is not published. We hoped to inform policymakers about COVID-19 research trends in a timely manner, which meant we worked with data available at the time (in Spring 2021) rather than waiting until data has been expanded, cleaned or validated. Elsevier and Clarivate databases were also examined; these databases are more carefully curated for quality. We tapped them for comparisons to CORD-19, and especially for non-COVID research. We had planned to test whether the pandemic had an impact on non-COVID research but we were unable to show this outcome: During 2020, peer reviews were delayed [ 36 ], researchers were not able to access labs or other resources, and scholarship was interrupted, but these obstacles are not yet evident in the data. Disruptions to 2020 research activities likely will not be seen in publication data until 2022 and after. We also exclude preprint publications from this analysis, which could present a limitation, given the important role of preprints during the pandemic.
Further, a limitation of this analysis is the reliance on nations as ‘super-nodes’ in a network that consists of individuals with associated cultural contexts that are not captured in network data. This reliance on nations is partly justified in that nations represent the underlying political and social systems that support scientific activities by offering funding, infrastructure, training, and dissemination of results. We acknowledge that the reliance on nations as a unit of measurement is a limitation, imposed on the analyst based upon the ways in which data are collected. Future research will need to ensure cleaner data to validate comparisons presented here. None of these datasets can truly represent the scope of activities that contributed to what is known about COVID-19, and mechanisms to assess knowledge flows are quite limited and time consuming to collect.
A review of one year of research publications about the novel coronavirus that emerged in Wuhan China in late 2019 shows the research community reacting rapidly and robustly to the challenge. The rapidity of response to COVID-19 suggests flexibility in the research system: thousands of researchers from many fields began working on the crisis. Research was disseminated initially in a flood of preprints; rapidly peer-reviewed publications were placed on open data platforms or shared openly on subscription-based platforms. COVID-related, peer-reviewed publications rose sharply in number in early 2020, and these publications were much more likely to be shared on open-access platforms or formats to enable rapid knowledge diffusion than is the norm in scholarly publishing [ 17 ]. The earlist COVID-19 research efforts were conducted by China, the USA, and the UK, and these three nations constitute close to 50% of all COVID publications on the subject in 2020. European nations started off slowly in research publications, but these nations continued to grow their output throughout 2020, as China’s output dropped.
As expected, international collaborations accounted for a smaller percentage of publications than is generally seen in ‘normal’ times, where internationally co-authored articles often account for more than one-quarter of articles [put new footnote here that was added on page 4 and delete this note] We expected the drop-off in international publications because a lack of mobility meant that people were unable to meet and discuss shared insights, or to devise, carry out or compare research results. Remote collaborations involve higher transaction costs and could be expected to slow progress. This possibly explains why international teams were smaller: to cut down on the time needed for communication. Further, the lower rate of international collaboration may be due to topics related to patient care and public health specific to particular regions or nations rendering them less suited to international collaboration [ 3 ]. We also noted that distance was less of a barrier to collaboration than is shown in other studies in times before the COVID-19 crisis.
Interestingly, the number of papers per nation tracks closely with the outbreak of COVID-19 cases in that nation. We expected to find numbers of publications to be more closely correlated to research funding. This may still be the case, but the data is too variable and incomparable to elicit a correlation between funding and output. We surmise that researchers were motived by a desire to be helpful to those suffering with the disease. It may also be the case that local COVID cases provided observational opportunities for researchers, and thus publication opportunities, as well, which produced data that resulted in more national publications.
The low rate of participation by lower-income nations was somewhat unexpected. Lower-income nations had a very low rate of participation in the early days of COVID, and only slowly joined the global publication counts. This may be due to a number of factors, including the need to publish locally to address the crisis, the inability of researchers to access laboratories or data during lock-down periods, the lack of access to one’s office, or the inability of national ministries to provide emergency research funds. People may not have had access to the Internet at home. The lower showing for developing nations is a concern, since these nations need the scientific knowledge to battle viral events just as much or more than advanced nations. This finding clearly requires more research and perhaps policy action.
We expected that the combined rush to work on COVID and the pandemic lock-downs would reduce non-COVID research activities. This may still be the case (reported in a survey by Myer et al. [ 2 ]), but it could not be detected in publication numbers at this writing. Publications in life and health sciences in non-COVID topics increased in number over 2019. As researchers become more comfortable with remote work, they may have persisted in publishing earlier results, however, this does not comport with the findings of Myer et al. [ 2 ]. As the pipeline catches up these activities, it will be worth revisiting the impact on productivity again at the end of 2021.
The stratification and consolidation comport with a model of global science as a reputation-based system creating a social hierarchy: the global network reverts to scientifically advanced nations and elite institutions in a crisis. We expected that the number of papers would align with reputation and resources. The role of reputation is confirmed by the consolidation of actors to fewer, elite institutions in scientifically advanced nations cooperating together more so than prior to the pandemic. The role played by access to resources is unclear—we observe that national output is closely tied to number of COVID-19, which could be due to a desire of scientists to help the effort. This requires more inquiry.
The influence of geopolitical factors also appears to play some role in research output, partnership and productivity. Arguably, Chinese publications initiated most of the COVID-19 research into the nature of the SARS-CoV-2 virus itself [ 29 – 31 ]. Nevertheless, in April 2020, the Chinese government changed requirements for review of articles related to the origins of COVID-19, requiring a more central review for work about the source of the novel coronavirus, which may have reduced the willingness of Chinese authors to cooperate internationally, although this requires more inquiry. Negative political comments made in the United States against China regarding the source of the virus may also have dampened international collaboration, although we did not test for this possibility.
Time and attention may have played a role in the drop in the share of internationally co-authored papers. Transaction costs of distance communications may have hampered some international connections. The drop-off in number of developing nations participating at the start of the pandemic may have contributed to the drop in international collaboration numbers, as well, by lowering the number of potential collaborators.
A remaining question arises around the mechanisms by which people, who had not already worked together before the pandemic, became connected to one another in a year when most people were physically isolated from each other. These connections are what Liu et al. [ 18 ] term “parachuting collaborations.” One would expect that people connect face-to-face: Research shows that the vast majority of collaborative projects start face-to-face or side-by-side. When that cannot take place, it is unclear whether people look for physically proximate partners, choose to work alone, become connected to new people through friend-of-a-friend, through social media, or perhaps just a ‘cold-call’ outreach to someone they do not know. It is clear that many of the connections made around COVID-19 may not have existed prior to the pandemic, so further research is needed to understand how people connected with each other under crisis conditions.
Supporting information
S1 appendix. network visuals..
https://doi.org/10.1371/journal.pone.0261624.s001
Acknowledgments
Thanks go to Clayton E. Tillman and Thomas Collins for help with data collection and formatting.
- View Article
- PubMed/NCBI
- Google Scholar
- 4. National Science Board. The state of U.S. science and engineering. Washington, DC: US Government Printing Office; 2020.
- 7. Glänzel W, Schubert A. Analysing scientific networks through co-authorship. In Handbook of quantitative science and technology research: The Use of Publication and Patent Statistics in Studies of S&T Systems, Moed H., ed., Kluwer Academic Publishers, Amsterdam, 2004. pp. 257–76.
- 13. Preprints contain the results of research activities, but they have not yet been subject to peer review.
- 17. Fry CV, MacGarvie M. Drinking from the firehose: Preprints, Chinese researchers, and the diffusion of knowledge in COVID-19. 2021.
- 18. Liu M, Bu Y, Chen C, Xu J, Li D, Leng Y, et al. Can pandemics transform scientific novelty? Evidence from COVID-19. arXiv. 2020.
- 20. Aviv-Reuven S, Rosenfeld A. Publication patterns’ changes due to the COVID-19 pandemic: A longitudinal and short-term scientometric analysis. arXiv. 2021:02594.
- 26. Horbach SPJM. No time for that now! Qualitative changes in manuscript peer review during the Covid-19 pandemic. Research Evaluation. 2021;(rvaa037).
- 32. DFID. Low-income countries (LIS) as defined by Department for International Development (DFID) of UK [cited 2021]. https://g2lm-lic.iza.org/call-phase-iv/list-of-lic/ .
How to Write About Coronavirus in a College Essay
Students can share how they navigated life during the coronavirus pandemic in a full-length essay or an optional supplement.
Writing About COVID-19 in College Essays

Getty Images
Experts say students should be honest and not limit themselves to merely their experiences with the pandemic.
The global impact of COVID-19, the disease caused by the novel coronavirus, means colleges and prospective students alike are in for an admissions cycle like no other. Both face unprecedented challenges and questions as they grapple with their respective futures amid the ongoing fallout of the pandemic.
Colleges must examine applicants without the aid of standardized test scores for many – a factor that prompted many schools to go test-optional for now . Even grades, a significant component of a college application, may be hard to interpret with some high schools adopting pass-fail classes last spring due to the pandemic. Major college admissions factors are suddenly skewed.
"I can't help but think other (admissions) factors are going to matter more," says Ethan Sawyer, founder of the College Essay Guy, a website that offers free and paid essay-writing resources.
College essays and letters of recommendation , Sawyer says, are likely to carry more weight than ever in this admissions cycle. And many essays will likely focus on how the pandemic shaped students' lives throughout an often tumultuous 2020.
But before writing a college essay focused on the coronavirus, students should explore whether it's the best topic for them.
Writing About COVID-19 for a College Application
Much of daily life has been colored by the coronavirus. Virtual learning is the norm at many colleges and high schools, many extracurriculars have vanished and social lives have stalled for students complying with measures to stop the spread of COVID-19.
"For some young people, the pandemic took away what they envisioned as their senior year," says Robert Alexander, dean of admissions, financial aid and enrollment management at the University of Rochester in New York. "Maybe that's a spot on a varsity athletic team or the lead role in the fall play. And it's OK for them to mourn what should have been and what they feel like they lost, but more important is how are they making the most of the opportunities they do have?"
That question, Alexander says, is what colleges want answered if students choose to address COVID-19 in their college essay.
But the question of whether a student should write about the coronavirus is tricky. The answer depends largely on the student.
"In general, I don't think students should write about COVID-19 in their main personal statement for their application," Robin Miller, master college admissions counselor at IvyWise, a college counseling company, wrote in an email.
"Certainly, there may be exceptions to this based on a student's individual experience, but since the personal essay is the main place in the application where the student can really allow their voice to be heard and share insight into who they are as an individual, there are likely many other topics they can choose to write about that are more distinctive and unique than COVID-19," Miller says.
Opinions among admissions experts vary on whether to write about the likely popular topic of the pandemic.
"If your essay communicates something positive, unique, and compelling about you in an interesting and eloquent way, go for it," Carolyn Pippen, principal college admissions counselor at IvyWise, wrote in an email. She adds that students shouldn't be dissuaded from writing about a topic merely because it's common, noting that "topics are bound to repeat, no matter how hard we try to avoid it."
Above all, she urges honesty.
"If your experience within the context of the pandemic has been truly unique, then write about that experience, and the standing out will take care of itself," Pippen says. "If your experience has been generally the same as most other students in your context, then trying to find a unique angle can easily cross the line into exploiting a tragedy, or at least appearing as though you have."
But focusing entirely on the pandemic can limit a student to a single story and narrow who they are in an application, Sawyer says. "There are so many wonderful possibilities for what you can say about yourself outside of your experience within the pandemic."
He notes that passions, strengths, career interests and personal identity are among the multitude of essay topic options available to applicants and encourages them to probe their values to help determine the topic that matters most to them – and write about it.
That doesn't mean the pandemic experience has to be ignored if applicants feel the need to write about it.
Writing About Coronavirus in Main and Supplemental Essays
Students can choose to write a full-length college essay on the coronavirus or summarize their experience in a shorter form.
To help students explain how the pandemic affected them, The Common App has added an optional section to address this topic. Applicants have 250 words to describe their pandemic experience and the personal and academic impact of COVID-19.
"That's not a trick question, and there's no right or wrong answer," Alexander says. Colleges want to know, he adds, how students navigated the pandemic, how they prioritized their time, what responsibilities they took on and what they learned along the way.
If students can distill all of the above information into 250 words, there's likely no need to write about it in a full-length college essay, experts say. And applicants whose lives were not heavily altered by the pandemic may even choose to skip the optional COVID-19 question.
"This space is best used to discuss hardship and/or significant challenges that the student and/or the student's family experienced as a result of COVID-19 and how they have responded to those difficulties," Miller notes. Using the section to acknowledge a lack of impact, she adds, "could be perceived as trite and lacking insight, despite the good intentions of the applicant."
To guard against this lack of awareness, Sawyer encourages students to tap someone they trust to review their writing , whether it's the 250-word Common App response or the full-length essay.
Experts tend to agree that the short-form approach to this as an essay topic works better, but there are exceptions. And if a student does have a coronavirus story that he or she feels must be told, Alexander encourages the writer to be authentic in the essay.
"My advice for an essay about COVID-19 is the same as my advice about an essay for any topic – and that is, don't write what you think we want to read or hear," Alexander says. "Write what really changed you and that story that now is yours and yours alone to tell."
Sawyer urges students to ask themselves, "What's the sentence that only I can write?" He also encourages students to remember that the pandemic is only a chapter of their lives and not the whole book.
Miller, who cautions against writing a full-length essay on the coronavirus, says that if students choose to do so they should have a conversation with their high school counselor about whether that's the right move. And if students choose to proceed with COVID-19 as a topic, she says they need to be clear, detailed and insightful about what they learned and how they adapted along the way.
"Approaching the essay in this manner will provide important balance while demonstrating personal growth and vulnerability," Miller says.
Pippen encourages students to remember that they are in an unprecedented time for college admissions.
"It is important to keep in mind with all of these (admission) factors that no colleges have ever had to consider them this way in the selection process, if at all," Pippen says. "They have had very little time to calibrate their evaluations of different application components within their offices, let alone across institutions. This means that colleges will all be handling the admissions process a little bit differently, and their approaches may even evolve over the course of the admissions cycle."
Searching for a college? Get our complete rankings of Best Colleges.
10 Ways to Discover College Essay Ideas

Tags: students , colleges , college admissions , college applications , college search , Coronavirus
2024 Best Colleges

Search for your perfect fit with the U.S. News rankings of colleges and universities.
College Admissions: Get a Step Ahead!
Sign up to receive the latest updates from U.S. News & World Report and our trusted partners and sponsors. By clicking submit, you are agreeing to our Terms and Conditions & Privacy Policy .
Ask an Alum: Making the Most Out of College
You May Also Like
Q&a: college alumni engagement.
LaMont Jones, Jr. May 20, 2024

10 Destination West Coast College Towns
Cole Claybourn May 16, 2024

Scholarships for Lesser-Known Sports
Sarah Wood May 15, 2024

Should Students Submit Test Scores?
Sarah Wood May 13, 2024

Poll: Antisemitism a Problem on Campus
Lauren Camera May 13, 2024

Federal vs. Private Parent Student Loans
Erika Giovanetti May 9, 2024

14 Colleges With Great Food Options
Sarah Wood May 8, 2024

Colleges With Religious Affiliations
Anayat Durrani May 8, 2024

Protests Threaten Campus Graduations
Aneeta Mathur-Ashton May 6, 2024

Protesting on Campus: What to Know
Sarah Wood May 6, 2024


COVID-19 and Higher Education
This RAPID award is made by the Improving Undergraduate STEM Education program in the Division of Undergraduate Education (Education and Human Resources Directorate), using funds from the Coronavirus Aid, Relief, and Economic Security (CARES) Act.
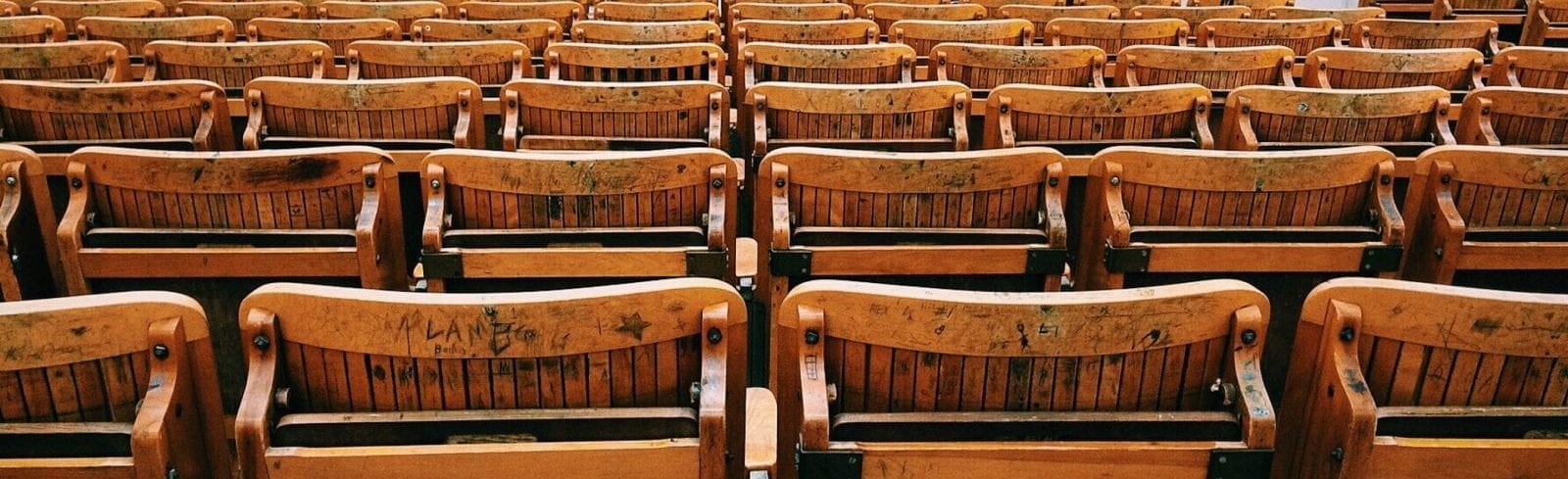
Project Abstract
How do institutions communicate about COVID-19? How do COVID-19 changes impact faculty and students? What resources are most important for the demonstration of resilience across undergraduate education? In order to answer these questions, we are randomly sampling institutions across the United States using the Postsecondary Education Data System (IPEDS) to represent various school sizes and types. Our hope is that this method of sampling will be inclusive and best represent experiences across the United States. We will be collecting crisis communication documents from the universities as well as conducting three waves of surveys and follow-up interviews. Through such a rigorous process we might be able to better measure experiences, reactions and how these unfold over time. This research is unique as we are studying the pandemic while it unfolds.
This research offers an opportunity to help the greater academic community that has been significantly uprooted and disrupted by COVID-19. There are dozens of other studies going on at the same time to unlock the secrets of this unprecedented event. Our approach is specifically designed in a way that will help us contribute a substantial piece of the puzzle. By opening a channel for the participating institutions, faculty, and students to systematically share their experience and their voices, we hope to contribute to a stronger, more resilient system of Higher Education that will emerge from this experience with the information and lessons needed to thrive in the future.
This project will examine the experiences faced by faculty and students in higher education during the COVID-19 pandemic. To understand the complexity of the situation we combine theory and best practices from the fields of I/O Psychology, Crisis Communication, Disaster Management, and Higher Education. Essentially, better understanding faculty and student perspectives will help us understand what sorts of directional changes Higher Ed might take in future adverse situations.
The majority of our data collection will take place in the summer and fall of 2020 and we will be working with the data for about one year.
Our recruitment process ensures that results will be generalizable to all higher education institutions in the United States, no matter whether they are public or private, large or small, located in urban or rural settings, or minority-serving.
- Open access
- Published: 14 May 2024
Rain, rain, go away, come again another day: do climate variations enhance the spread of COVID-19?
- Masha Menhat 1 ,
- Effi Helmy Ariffin ORCID: orcid.org/0000-0002-8534-0113 2 ,
- Wan Shiao Dong 3 ,
- Junainah Zakaria 2 ,
- Aminah Ismailluddin 3 ,
- Hayrol Azril Mohamed Shafril 4 ,
- Mahazan Muhammad 5 ,
- Ahmad Rosli Othman 6 ,
- Thavamaran Kanesan 7 ,
- Suzana Pil Ramli 8 ,
- Mohd Fadzil Akhir 2 &
- Amila Sandaruwan Ratnayake 9
Globalization and Health volume 20 , Article number: 43 ( 2024 ) Cite this article
353 Accesses
3 Altmetric
Metrics details
The spread of infectious diseases was further promoted due to busy cities, increased travel, and climate change, which led to outbreaks, epidemics, and even pandemics. The world experienced the severity of the 125 nm virus called the coronavirus disease 2019 (COVID-19), a pandemic declared by the World Health Organization (WHO) in 2019. Many investigations revealed a strong correlation between humidity and temperature relative to the kinetics of the virus’s spread into the hosts. This study aimed to solve the riddle of the correlation between environmental factors and COVID-19 by applying RepOrting standards for Systematic Evidence Syntheses (ROSES) with the designed research question. Five temperature and humidity-related themes were deduced via the review processes, namely 1) The link between solar activity and pandemic outbreaks, 2) Regional area, 3) Climate and weather, 4) Relationship between temperature and humidity, and 5) the Governmental disinfection actions and guidelines. A significant relationship between solar activities and pandemic outbreaks was reported throughout the review of past studies. The grand solar minima (1450-1830) and solar minima (1975-2020) coincided with the global pandemic. Meanwhile, the cooler, lower humidity, and low wind movement environment reported higher severity of cases. Moreover, COVID-19 confirmed cases and death cases were higher in countries located within the Northern Hemisphere. The Blackbox of COVID-19 was revealed through the work conducted in this paper that the virus thrives in cooler and low-humidity environments, with emphasis on potential treatments and government measures relative to temperature and humidity.
• The coronavirus disease 2019 (COIVD-19) is spreading faster in low temperatures and humid area.
• Weather and climate serve as environmental drivers in propagating COVID-19.
• Solar radiation influences the spreading of COVID-19.
• The correlation between weather and population as the factor in spreading of COVID-19.
Graphical abstract
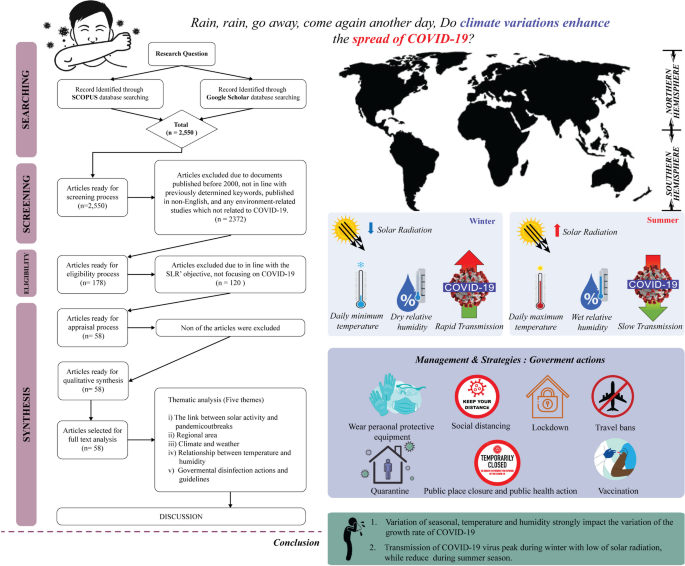
Introduction
The revolution and rotation of the Earth and the Sun supply heat and create differential heating on earth. The movements and the 23.5° inclination of the Earth [ 1 ] separate the oblate-ellipsoid-shaped earth into northern and southern hemispheres. Consequently, the division results in various climatic zones at different latitudes and dissimilar local temperatures (see Fig. 1 ) and affects the seasons and length of a day and night in a particular region [ 2 ]. Global differential heating and climate variability occur due to varying solar radiation received by each region [ 3 ]. According to Trenberth and Fasullo [ 4 ] and Hauschild et al. [ 5 ] the new perspective on the issue of climate change can be affected relative to the changes in solar radiation patterns. Since the study by Trenberth and Fasullo [ 4 ] focused on climate model changes from 1950 to 2100, it was found that the role of changing clouds and trapped sunlight can lead to an opening of the aperture for solar radiation.
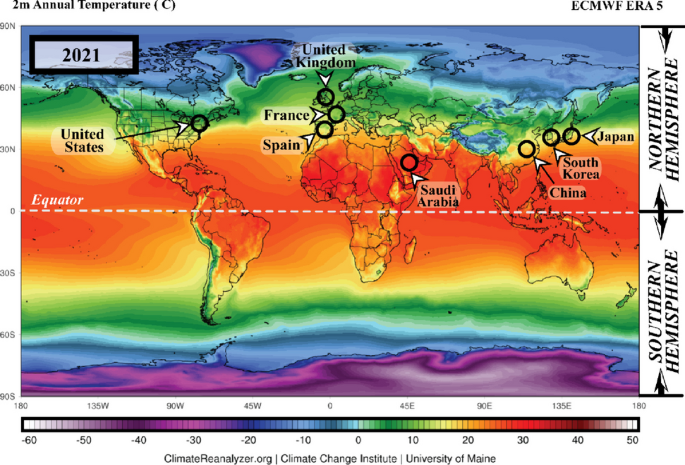
The annual average temperature data for 2021 in the northern and southern hemispheres ( Source: meteoblue.com ). Note: The black circles mark countries with high Coronavirus disease 2019 (COVID-19) infections
Furthermore, the heat from sunlight is essential to humans; several organisms could not survive without it. Conversely, the spread of any disease-carrying virus tends to increase with less sunlight exposure [ 6 ]. Historically, disease outbreaks that led to epidemic and pandemic eruptions were correlated to atmospheric changes. Pandemic diseases, such as the flu (1918), Asian flu (1956–1958), Hong Kong flu (1968), and recently, the coronavirus disease 2019 (COVID-19) (2019), recorded over a million death toll each during the winter season or minimum temperature conditions [ 7 ]. The total number of COVID-19 cases is illustrated in Fig. 2 .
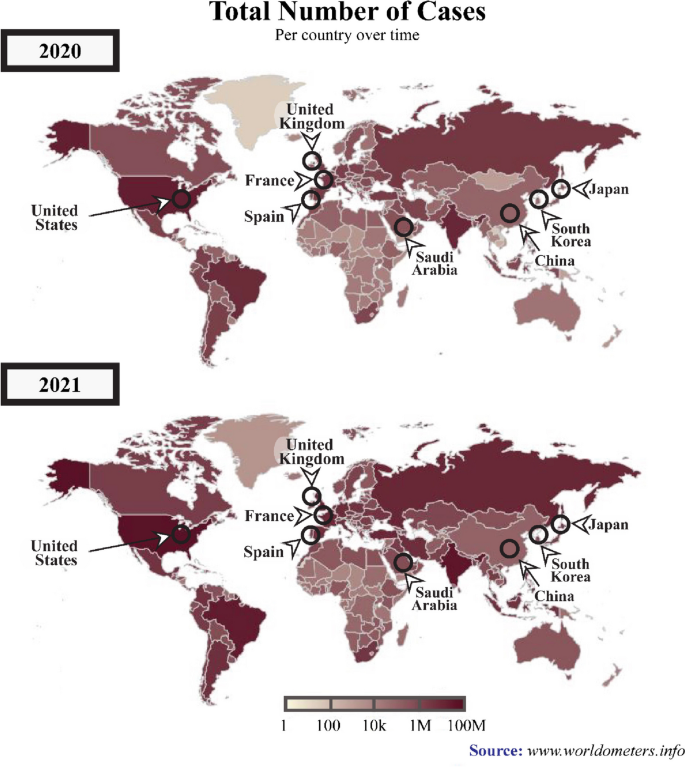
A graphical representation of the total number of COVID-19 cases across various periods between 2020 and 2021. ( Source : www.worldometers.info ). Note: The black circles indicate countries with high numbers COVID-19-infections
In several previous outbreaks, investigations revealed a significant association between temperature and humidity with a particular focus on the transmission dynamics of the infection from the virus into the hosts [ 8 , 9 , 10 ]. Moreover, disease outbreaks tended to heighten in cold temperatures and low humidity [ 11 ]. Optimal temperature and sufficient relative humidity during evaporation are necessary for cloud formation, resulting in the precipitated liquid falling to the ground as rain, snow, or hail due to the activity of solar radiation balancing [ 4 ].
Consequently, the radiation balancing processes in the atmosphere are directly linked to the living beings on the earth, including plants and animals, and as well as viruses and bacterias. According to Carvalho et al. [ 12 ]‘s study, the survival rate of the Coronaviridae Family can decrease during summer seasons. Nevertheless, numerous diseases were also developed from specific viruses, such as influenza, malaria, and rubella, and in November 2019, a severe health threat originated from a 125 nm size of coronavirus, had resulted in numerous deaths worldwide.
Transmission and symptoms of COVID-19
The COVID-19, or severe acute respiratory syndrome coronavirus 2 (SARS-CoV-2), is an infectious disease caused by a newly discovered pathogenic virus from the coronavirus family, the novel coronavirus (2019-nCoV) [ 13 ]. The first case was recorded in Wuhan, China, in December 2019 [ 14 ]. The pathogenic virus is transmitted among humans when they breathe in air contaminated with droplets and tiny airborne particles containing the virus [ 14 , 15 , 16 , 17 , 18 ].
According to the World Health Organization (WHO), the most common symptoms of COVID-19 infection include fever, dry cough, and tiredness. Nevertheless, older people and individuals with underlying health problems (lung and heart problems, high blood pressure, diabetes, or cancer) are at higher risk of becoming seriously ill and developing difficulty breathing [ 19 ]. The COVID-19 was initially only predominant in China but rapidly spread to other countries globally. The remarkably swift acceleration of the number of infections and mortality forced WHO to declare COVID-19 a global public health emergency on the 30th of January 2020, which was later declared as a pandemic on the 11th of March 2020 [ 20 ].
Since no vaccine was available then, WHO introduced the COVID-19 preventative measures to reduce the chances of virus transmission. The guideline for individual preventative included practising hand and respiratory hygiene by regularly cleaning hands with soap and water or alcohol-based sanitisers, wear a facemask and always maintaining at least a one-meter physical distance [ 21 ]. Nevertheless, the worldwide transmission of COVID-19 has resulted in fear and forced numerous countries to impose restrictions rules, such as lockdown, travel bans, closed country borders, restrictions on shipping activities, and movement limitations, to diminish the spread of COVID-19 [ 22 ].
According to WHO, by the 2nd of December 2020, 63,379,338 confirmed cases and 1,476,676 mortalities were recorded globally. On the 3rd of December 2021, 263,655,612 confirmed cases and deaths were recorded, reflecting increased COVID-19 infections compared to the previous year. The American and European regions documented the highest COVID-19 patients with 97,341,769 and 88,248,591 cases, respectively (see Fig. 2 ), followed by Southeast Asia with 44,607,287, Eastern Mediterranean accounted 16,822,791, Western Pacific recorded 6,322,034, and Africa reported the lowest number of cases at 6,322,034 [ 19 ].
Recently, an increasing number of studies are investigating the association between environmental factors (temperature and humidity) and the viability, transmission, and survival of the coronavirus [ 23 , 24 , 25 , 26 ]. The results primarily demonstrated that temperature was more significantly associated with the transmission of COVID-19 [ 27 , 28 , 29 ] and its survival period on the surfaces of objects [ 30 ]. Consequently, the disease was predominant in countries with low temperature and humidity [ 31 ], which was also proven by Diao et al. [ 32 ]‘s study demonstrating higher rates of COVID-19 transmission in China, England, Germany, and Japan.
A comprehensive systematic literature review (SLR) is still lacking despite numerous research on environmental factors linked to coronavirus. Accordingly, this article aimed to fill the gap in understanding and identifying the correlation between environmental factors and COVID-19 by analysing existing reports. Systematically reviewing existing literature is essential to contribute to the body of knowledge and provide beneficial information for public health policymakers.
Methodology
The present study reviewed the protocols, formulation of research questions, selection of studies, appraisal of quality, and data abstraction and analysis.
The protocol review
The present SLR was performed according to the reporting standards for systematic evidence syntheses (ROSES) and followed or adapted the guidelines as closely as possible. Thus, in this study, a systematic literature review was guided by the ROSES review protocol (Fig. 3 ). Compared to preferred reporting items for systematic review and meta-analysis (PRISMA), ROSES is a review protocol specifically designed for a systematic review in the conservation or environment management fields [ 33 ]. Compared to PRISMA, ROSES offers several advantages, as it is tailored to environmental systematic review, which reduces emphasis on quantitative synthesis (e.g. meta-analysis etc.) that is only reliable when used with appropriate data [ 34 ].
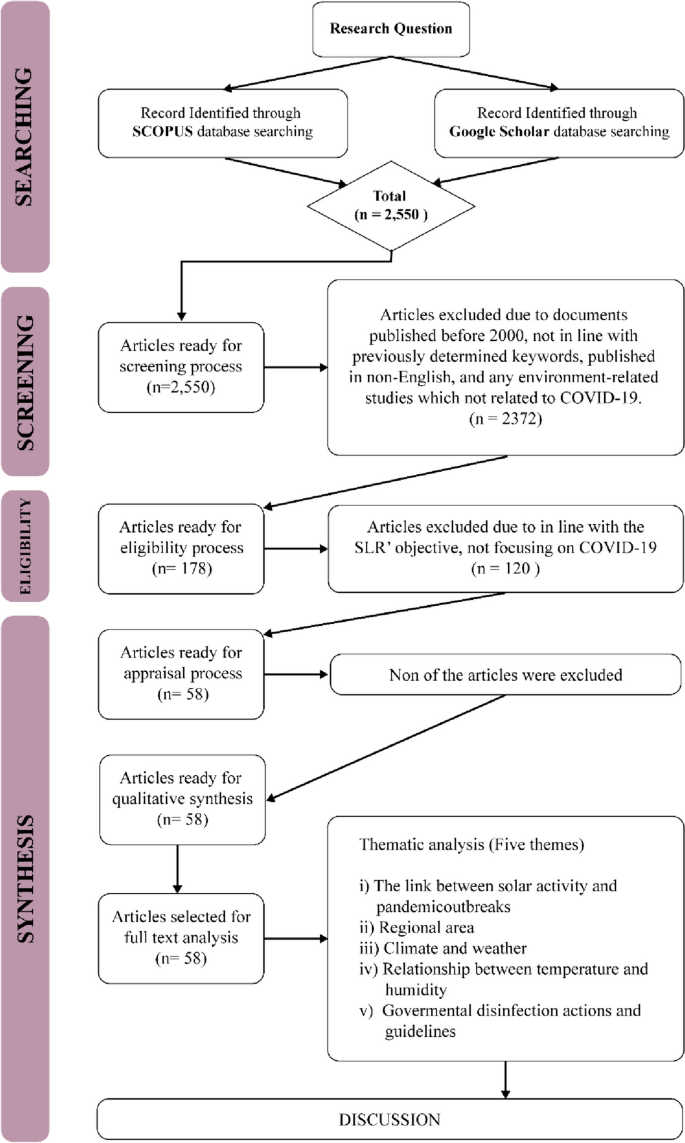
The flow diagram guide by ROSES protocol and Thematical Analysis
The current SLR started by determining the appropriate research questions, followed by the selection criteria, including the review, specifically on the keywords employed and the selection of journals database. Subsequently, the appraisal quality process and data abstraction and analysis were conducted.
Formulation of research questions
The entire process of this SLR was guided by the specific research questions, while sources to be reviewed and data abstraction and analysis were in line with the determined research question [ 35 , 36 ]. In the present article, a total of five research questions were formed, namely:
What the link between solar activity and COVID-19 pandemic outbreaks?
Which regions were more prone to COVID-19?
What were the temporal and spatial variabilities of high temperature and humidity during the spread of COVID-19?
What is the relationship between temperature and humidity in propagating COVID-19?
How did the government’s disinfection actions and guidelines can be reducing the spread of COVID-19?
Systematic searching strategies
Selection of studies.
In this stage of the study, the appropriate keywords to be employed in the searching process were determined. After referring to existing literature, six main keywords were chosen for the searching process, namely COVID-19, coronavirus, temperature, humidity, solar radiation and population density. The current study also utilised the boolean operators (OR, AND, AND NOT) and phrase searching.
Scopus was employed as the main database during the searching process, in line with the suggestion by Gusenbauer and Haddaway [ 37 ], who noted the strength of the database in terms of quality control and search and filtering functions. Furthermore, Google Scholar was selected as the supporting database. Although Halevi et al. [ 38 ] expressed concerns about its quality, Haddaway et al. [ 39 ] reported that due to its quantity, Google Scholar was suitable as a supporting database in SLR studies.
In the first stage of the search, 2550 articles were retrieved, which were then screened. The suitable criteria were also determined to control the quality of the articles reviewed [ 40 ]. The criteria are: any documents published between 2000 to 2022, documents that consist previously determined keywords, published in English, and any environment-related studies that focused on COVID-19. Based on these criteria, 2372 articles were excluded and 178 articles were proceeded to the next step namely eligibility. In the eligibility process, the title and the abstract of the articles were examined to ensure its relevancy to the SLR and in this process a total of 120 articles were excluded and only 58 articles were processed in the next stage.
Appraisal of the quality
The study ensured the rigor of the chosen articles based on best evidence synthesis. In the process, predefined inclusion criteria for the review were appraised by the systematic review team based on previously established guidelines and the studies were then judged as being scientifically admissible or not [ 40 ]. Hence, by controlling the quality based on the best evidence synthesis, the present SLR controls its quality by including articles that are in line with the inclusion criteria. It means that any article published within the timeline (in the year 2000 and above), composed of predetermined keywords, in English medium, and environment-related investigations focusing on COVID-19 are included in the review. Based on this process, all 58 articles fulfilled all the inclusion criteria and are considered of good quality and included in the review.
Data abstraction and analysis
The data abstraction process in this study was performed based on five research questions (please refer to 2.2, formulation of research questions). The data that was able to answer the questions were abstracted and placed in a table to ease the data analysis process. The primary data analysis technique employed in the current study was qualitative and relied on thematic analysis.
The thematic technique is a descriptive method that combines data flexibly with other information evaluation methods [ 41 ], aiming to identify the patterns in studies. Any similarities and relationships within the abstracted data emerge as patterns. Subsequently, suitable themes and sub-themes would be developed based on obtained patterns [ 42 ]. Following the thematic process, five themes were selected in this study.
Background of the selected articles
The current study selected 58 articles for the SLR. Five themes were developed based on the thematic analysis from the predetermined research questions: the link between solar activity and pandemic outbreaks, regional area, climate and weather, the relationship between temperature and humidity, and government disinfection action guidelines. Among the articles retrieved between 2000 and 2022; two were published in 2010, one in 2011, four in 2013, three in 2014, two in 2015, six in 2016 and 2017, respectively, one in 2018, six in 2019, twelve in 2020, eight in 2021, and seven in 2022.
Temperature- and humidity-related themes
The link between solar activity and pandemic outbreaks.
Numerous scientists have investigated the relationship between solar activities and pandemic outbreaks over the years ([ 43 ]; A [ 27 , 44 , 45 ].). Nuclear fusions from solar activities have resulted in minimum and maximum solar sunspots. Maximum solar activities are characterised by a high number of sunspots and elevated solar flare frequency and coronal mass injections. Minimum solar sunspot occurrences are identified by low interplanetary magnetic field values entering the earth [ 1 ].
A diminished magnetic field was suggested to be conducive for viruses and bacteria to mutate, hence the onset of pandemics. Nonetheless, Hoyle and Wickramasinghe [ 46 ] reported that the link between solar activity and pandemic outbreaks is only speculative. The literature noted that the data recorded between 1930 and 1970 demonstrated that virus transmissions and pandemic occurrences were coincidental. Moreover, no pandemic cases were reported in 1979, when minimum solar activity was recorded [ 47 ].
Chandra Wickramasinghe et al. [ 48 ] suggested a significant relationship between pandemic outbreaks and solar activities as several grand solar minima, including Sporer (1450–1550 AD), Mounder (1650–1700 AD), and Dalton (1800–1830) minimums, were recorded coinciding with global pandemics of diseases, such as smallpox, the English sweat, plague, and cholera pandemics. Furthermore, since the Dalton minimum, which recorded minimum sunspots, studies from 2002 to 2015 have documented the reappearance of previous pandemics. For example, influenza subtype H1N1 1918/1919 episodically returned in 2009, especially in India, China, and other Asian countries. Zika virus, which first appeared in 1950, flared and became endemic in 2015, transmitted sporadically, specifically in African countries. Similarly, SARS-CoV was first recorded in China in 2002 and emerged as an outbreak, MERS-CoV, in middle east countries a decade later, in 2012.
In 2020, the World Data Centre Sunspot Index and Long-term Solar Observations ( http://sidc.be ) confirmed that a new solar activity was initiated in December 2019, during which a novel coronavirus pandemic also occurred, and present a same as the previous hypothesis. Nevertheless, a higher number of pandemic outbreaks were documented during low minimum solar activities, including Ebola (1976), H5N1 (Nipah) (1967–1968), H1N1 (2009), and COVID-19 (2019–current). Furthermore, Wickramasinghe and Qu [ 49 ] reported that since 1918 or 1919, more devastating and recurrent pandemics tend to occur, particularly after a century. Consequently, within 100 years, a sudden surge of influenza was recorded, and novel influenza was hypothesised to emerge.
Figure 4 demonstrates that low minimum solar activity significantly reduced before 2020, hence substantiating the claim that pandemic events are closely related to solar activities. Moreover, numerous studies (i.e. [ 43 ], Chandra [ 46 , 47 , 48 ]) reported that during solar minimums, new viruses could penetrate the surfaces of the earth and high solar radiation would result in lower infection rates, supporting the hypothesis mentioned above.
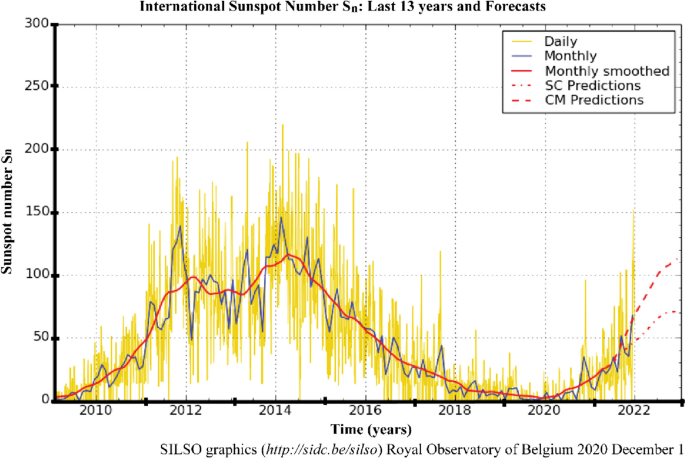
The number of sunspots in the last 13 years. Note : The yellow curve indicates the daily sunspot number and the 2010–2021 delineated curve illustrates the minimum solar activity recorded (source: http://sidc.be/silso )
Regional area
In early December 2019, Wuhan, China, was reported as the centre of the severe acute respiratory syndrome coronavirus 2 (SARS-CoV-2) outbreak [ 50 ]. Chinese health authorities immediately investigated and controlled the spread of the disease. Nevertheless, by late January 2020, the WHO announced that COVID-19 was a global public health emergency. The upgrade was due to the rapid rise in confirmed cases, which were no longer limited to Wuhan [ 28 ]. The disease had spread to 24 other countries, which were mainly in the northern hemisphere, particularly the European and Western Pacific regions, such as France, United Kingdom, Spain, South Korea, Japan, Malaysia, and Indonesia [ 51 , 52 ]. The migration or movement of humans was the leading agent in the spread of COVID-19, resulting in an almost worldwide COVID-19 pandemic [ 53 ].
The first hotspots of the epidemic outspread introduced by the Asian and Western Pacific regions possessed similar winter climates with an average temperature and humidity rate of 5–11 °C and 47–79%. Consequently, several publications reviewed in the current study associated the COVID-19 outbreak with regional climates (i.e. [ 1 , 29 , 54 , 55 ]) instead of its close connection to China. This review also discussed the effects of a range of specific climatological variables on the transmission and epidemiology of COVID-19 in regional climatic conditions.
America and Europe documented the highest COVID-19 cases, outnumbering the number reported in Asia [ 19 ] and on the 2nd of December 2020, the United States of America (USA) reported the highest number of confirmed COVID-19 infections, with over 13,234,551 cases and 264,808 mortalities (Da S [ 56 ].). The cases in the USA began emerging in March 2020 and peaked in late November 2020, during the wintertime in the northern hemisphere (December to March) [ 53 ]. Figure 5 demonstrates the evolution of the COVID-19 pandemic in several country which represent comparison two phase of summer and one phase of winter. Most of these countries tend to increase of COVID cases close to winter season. Then, it can be worsening on phase two of summer due to do not under control of human movement although the normal trend it is presenting during winter phase.
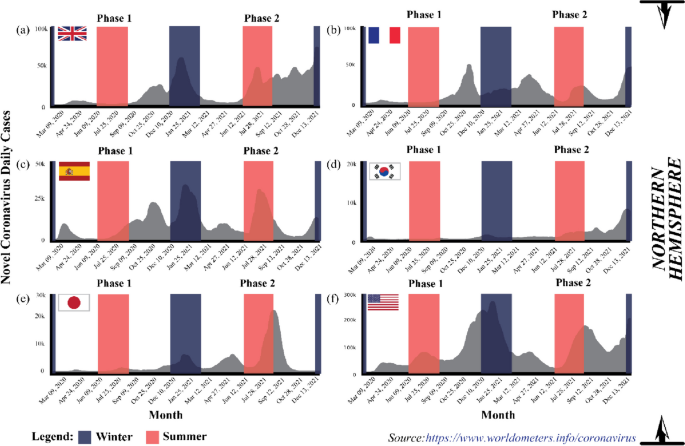
The evolution of the COVID-19 pandemic from the 15th of February 2020 to the 2nd of December 2020 ( Source: https://www.worldometers.info/coronavirus )
The coronavirus spread aggressively across the European region, which recorded the second highest COVID-19 confirmed cases after America. At the end of 2020, WHO reported 19,071,275 Covid-19 cases in the area, where France documented 2,183,275 cases, the European country with the highest number of confirmed cases, followed by the United Kingdom (1,629,661 cases) and Spain (1,652,801 cases) [ 19 ]. Europe is also located in the northern hemisphere and possesses a temperate climate.
The spatial and temporal transmission patterns of coronavirus infection in the European region were similar to America and the Eastern Mediterranean, where the winter season increased COVID-19 cases. Typically, winter in Europe occurs at the beginning of October and ends in March. Hardy et al. [ 57 ] also stated that temperature commonly drops below freezing (approximately − 1 °C) when snow accumulates between December to mid-March, resulting in an extreme environment. Figure 5 indicates that COVID-19 cases peaked in October when the temperature became colder [ 21 ]. Similarly, the cases were the highest in the middle of the year in Australia and South Asian countries, such as India, that experience winter and monsoon, respectively, during the period.
In African regions, the outbreak of COVID-19 escalated rapidly from June to October before falling from October to March, as summer in South Africa generally occurs from November to March, while winter from June to August. Nevertheless, heavy rainfall generally transpires during summer, hence the warm and humid conditions in South Africa and Namibia during summer, while the opposite happens during winter (cold and dry). Consequently, the outbreak in the region recorded an increasing trend during winter and subsided during the summer, supporting the report by Gunthe et al. [ 58 ]. Novel coronavirus disease presents unique and grave challenges in Africa, as it has for the rest of the world. However, the infrastructure and resources have limitations for Africa countries facing COVID-19 pandemic and the threat of other diseases [ 59 ].
Conclusively, seasonal and regional climate patterns were associated with COVID-19 outbreaks globally. According to Kraemer et al. [ 60 ], they used real-time mobility data in Wuhan and early measurement presented a positive correlation between human mobility and spread of COVID-19 cases. However, after the implementation of control measures, this correlation dropped and growth rates became negative in most locations, although shifts in the demographics of reported cases were still indicative of local chains of transmission outside of Wuhan.
Climate and weather
The term “weather” represents the changes in the environment that occur daily and in a short period, while “climate” is defined as atmospheric changes happening over a long time (over 3 months) in specific regions. Consequently, different locations would experience varying climates. Numerous reports suggested climate and weather variabilities as the main drivers that sped or slowed the transmission of SARS-CoV-2 worldwide [ 44 , 61 , 62 , 63 ].
From a meteorological perspective, a favourable environment has led to the continued existence of the COVID-19 virus in the atmosphere [ 64 ]. Studies demonstrated that various meteorological conditions, such as the rate of relative humidity (i.e. [ 28 ]), precipitation (i.e. [ 65 ]), temperature (i.e. [ 66 ]), and wind speed factors (i.e. [ 54 ]), were the crucial components that contributed to the dynamic response of the pandemic, influencing either the mitigation or exacerbation of novel coronavirus transmission. In other words, the environment was considered the medium for spreading the disease when other health considerations were put aside. Consequently, new opinions, knowledge, and findings are published and shared to increase awareness, thus encouraging preventive measures within the public.
The coronavirus could survive in temperatures under 30 °C with a relative humidity of less than 80% [ 67 ], suggesting that high temperatures and lower relative humidity contributed to the elicitation of COVID-19 cases [ 18 , 51 , 58 , 68 ]. Lagtayi et al. [ 7 ] highlighted temperature as a critical factor, evidently from the increased transmission rate of MERS-Cov in African states with a warm and dry climate. Similarly, the highest COVID-19 cases were recorded in dry temperate regions, especially in western Europe (France and Spain), China, and the USA, while the countries nearer to the equator were less affected. Nevertheless, the temperature factor relative to viral infections depends on the protein available in the viruses. According to Chen and Shakhnovich [ 69 ], there is a good correlation between decreasing temperature and the growth of proteins in virus. Consequently, preventive measures that take advantage of conducive environments for specific viruses are challenging.
Precipitation also correlates with influenza [ 43 ]. A report demonstrated that regions with at least 150 mm of monthly precipitation threshold level experienced fewer cases than regions with lower precipitation rates. According to Martins et al. [ 70 ], influenza and COVID-19 can be affected by climate, where virus can be spread through the respiratory especially during rainfall season. The daily spread of Covid-19 cases in tropical countries, which receive high precipitation levels, are far less than in temperate countries [ 27 ]. Likewise, high cases of COVID-19 were reported during the monsoon season (mid-year) in India during which high rainfall is recorded [ 71 ]. Moreover, the majority of the population in these regions has lower vitamin D levels, which may contribute to weakened immune responses during certain seasons [ 27 ].
Rainfall increases the relative atmospheric humidity, which is unfavourable to the coronaviruses as its transmission requires dry and cold weather. Moreover, several reports hypothesised that rain could wash away viruses on object surfaces, which is still questioned. Most people prefer staying home on rainy days, allowing less transmission or close contact. Conversely, [ 72 ] exhibited that precipitation did not significantly impact COVID-19 infectiousness in Oslo, Norway due the location in northern hemisphere which are during winter season presenting so cold.
Coşkun et al. [ 54 ] and Wu et al. [ 29 ] claimed that wind could strongly correlate with the rate of COVID-19 transmission. Atmospheric instability (turbulent occurrences) leads to increased wind speed and reduces the dispersion of particulate matter (PM 2.5 and PM 10 ) in the environment and among humans. An investigation performed in 55 cities in Italy during the COVID-19 outbreak proved that the areas with low wind movement (stable atmospheric conditions) possessed a higher correlation coefficient and exceeded the threshold value of the safe level of PM 2.5 and PM 10 . Resultantly, more individuals were recorded infected with the disease in the regions. As mentioned in Martins et al. [ 70 ] the COVID-19 can be affected by climate and the virus can be spread through respiratory which is the virus moving in the wind movement.
The relationship between temperature and humidity
Climatic parameters, such as temperature and humidity, were investigated as the crucial factors in the epidemiology of the respiratory virus survival and transmission of COVID-19 ([ 61 ]; S [ 73 , 74 ].). The rising number of confirmed cases indicated the strong transmission ability of COVID-19 and was related to meteorological parameters. Furthermore, several studies found that the disease transmission was associated with the temperature and humidity of the environment [ 55 , 64 , 68 , 75 ], while other investigations have examined and reviewed environmental factors that could influence the epidemiological aspects of Covid-19.
Generally, increased COVID-19 cases and deaths corresponded with temperature, humidity, and viral transmission and mortality. Various studies reported that colder and dryer environments favoured COVID-19 epidemiologically [ 45 , 76 , 77 ]. As example tropical region, the observations indicated that the summer (middle of year) and rainy seasons (end of the year) could effectively diminish the transmission and mortality from COVID-19. High precipitation statistically increases relative air humidity, which is unfavourable for the survival of coronavirus, which prefers dry and cold conditions [ 32 , 34 , 78 , 79 ]. Consequently, warmer conditions could reduce COVID-19 transmission. A 1 °C increase in the temperature recorded a decrease in confirmed cases by 8% increase [ 45 ].
Several reports established that the minimum, maximum, and average temperature and humidity correlated with COVID-19 occurrence and mortality [ 55 , 80 , 81 ]. The lowest and highest temperatures of 24 and 27.3 °C and a humidity between 76 and 91% were conducive to spreading the virulence agents. The propagation of the disease peaked at the average temperature of 26 °C and humidity of 55% before gradually decreasing with elevated temperature and humidity [ 78 ].
Researchers are still divided on the effects of temperature and humidity on coronavirus transmission. Xu et al. [ 26 ] confirmed that COVID-19 cases gradually increased with higher temperature and lower humidity, indicating that the virus was actively transmitted in warm and dry conditions. Nevertheless, several reports stated that the spread of COVID-19 was negatively correlated with temperature and humidity [ 10 , 29 , 63 ]. The conflicting findings require further investigation. Moreover, other factors, such as population density, elderly population, cultural aspects, and health interventions, might potentially influence the epidemiology of the disease and necessitate research.
Governmental disinfection actions and guidelines
The COVID-19 is a severe health threat that is still spreading worldwide. The epidemiology of the SAR-CoV-2 virus might be affected by several factors, including meteorological conditions (temperature and humidity), population density, and healthcare quality, that permit it to spread rapidly [ 16 , 17 ]. Nevertheless, in 2020, no effective pharmaceutical interventions or vaccines were available for the diagnosis, treatment, and epidemic prevention against COVID-19 [ 73 , 82 ]. Consequently, after 2020 the governments globally have designed and executed non-pharmacological public health measures, such as lockdown, travel bans, social distancing, quarantine, public place closure, and public health actions, to curb the spread of COVID-19 infections and several studies have reported on the effects of these plans [ 13 , 83 ].
The COVID-19 is mainly spread via respiratory droplets from an infected person’s mouth or nose to another in close contact [ 84 ]. Accordingly, WHO and most governments worldwide have recommended wearing facemasks in public areas to curb the transmission of COVID-19. The facemasks would prevent individuals from breathing COVID-19-contaminated air [ 85 ]. Furthermore, the masks could hinder the transmission of the virus from an infected person as the exhaled air is trapped in droplets collected on the masks, suspending it in the atmosphere for longer. The WHO also recommended adopting a proper hand hygiene routine to prevent transmission and employing protective equipment, such as gloves and body covers, especially for health workers [ 86 ].
Besides wearing protective equipment, social distancing was also employed to control the Covid-19 outbreak [ 74 , 87 ]. Social distancing hinders the human-to-human transmission of the coronavirus in the form of droplets from the mouth and nose, as evidenced by the report from Sun and Zhai [ 88 ]. Conversely, Nair & Selvaraj [ 89 ] demonstrated that social distancing was less effective in communities and cultures where gatherings are the norm. Nonetheless, the issue could be addressed by educating the public and implementing social distancing policies, such as working from home and any form of plague treatment.
Infected persons, individuals who had contact with confirmed or suspected COVID-19 patients, and persons living in areas with high transmission rates were recommended to undergo quarantine by WHO. The quarantine could be implemented voluntarily or legally enforced by authorities and applicable to individuals, groups, or communities (community containment) [ 90 ]. A person under mandatory quarantine must stay in a place for a recommended 14-day period, based on the estimated incubation period of the SARS-CoV-2 [ 19 , 91 ]. According to Stasi et al. [ 92 ], 14-days period for mandatory quarantine it is presenting a clinical improvement after they found 5-day group and 10-day group can be decrease number of patient whose getting effect of COVID-19 from 64 to 54% respectively. This also proven by Ahmadi et al. [ 43 ] and Foad et al. [ 93 ], quarantining could reduce the transmission of COVID-19.
Lockdown and travel bans, especially in China, the centre of the coronavirus outbreak, reduced the infection rate and the correlation of domestic air traffic with COVID-19 cases [ 17 ]. The observations were supported by Sun & Zhai [ 88 ] and Sun et al. [ 94 ], who noted that travel restrictions diminished the number of COVID-19 reports by 75.70% compared to baseline scenarios without restrictions. Furthermore, example in Malaysia, lockdowns improved the air quality of polluted areas especially in primarily at main cities [ 95 ]. As additional, Martins et al. [ 70 ] measure the Human Development Index (HDI) with the specific of socio-economic variables as income, education and health. In their study, the income and education levels are the main relevant factors that affect the socio-economic.
A mandatory lockdown is an area under movement control as a preventive measure to stop the coronavirus from spreading to other areas. Numerous governments worldwide enforced the policy to restrict public movements outside their homes during the pandemic. Resultantly, human-to-human transmission of the virus was effectively reduced. The lockdown and movement control order were also suggested for individuals aged 80 and above or with low or compromised immunities, as these groups possess a higher risk of contracting the disease [ 44 ].
Governments still enforced movement orders even after the introduction of vaccines by Pfizer, Moderna, and Sinovac, as the vaccines only protect high-risk individuals from the worst effects of COVID-19. Consequently, in most countries, after receiving the first vaccine dose, individuals were allowed to resume life as normal but were still required to follow the standard operating procedures (SOP) outlined by the government.
The government attempted to balance preventing COVID-19 spread and recovering economic activities, for example, local businesses, maritime traders, shipping activities, oil and gas production and economic trades [ 22 , 96 ]. Nonetheless, the COVID-19 cases demonstrated an increasing trend during the summer due to the higher number of people travelling and on vacation, primarily to alleviate stress from lockdowns. Several new variants were discovered, including the Delta and Omicron strains, which spread in countries such as the USA and the United Kingdom. The high number of COVID-19 cases prompted the WHO to suggest booster doses to ensure full protection.
As mentioned in this manuscript, the COVID-19 still uncertain for any kind factors that can be affected on spreading of this virus. However, regarding many sources of COVID-19 study, the further assessment on this factor need to be continue to be sure, that we ready to facing probably in 10 years projection of solar minimum phase can be held in same situation for another pandemic.
The sun has an eleven-year cycle known as the solar cycle, related to its magnetic field, which controls the activities on its surface through sunspots. When the magnetic fields are active, numerous sunspots are formed on its surface, hence the sun produces more radiation energy emitted to the earth. The condition is termed solar maximum (see Fig. 6 , denoted by the yellow boxes). Alternatively, as the magnetic field of the sun weakens, the number of sunspots decreases, resulting in less radiation energy being emitted to the earth. The phenomenon is known as the solar minimum (see Fig. 6 , represented by the blue boxes).
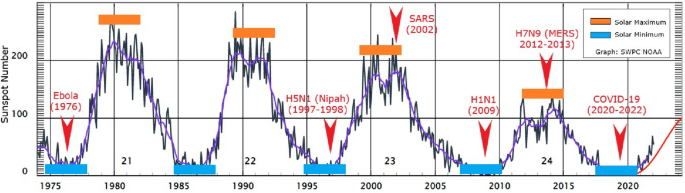
The emergence and recurrence of pandemics every 5 years in relation to solar activities ( Source: www.swpc.noaa.gov/ ). Note: The yellow boxes indicate the solar maximum, while the blue boxes represent the solar minimum
The magnetic field of the sun protects the earth from cosmic or galactic cosmic rays emitted by supernova explosions, stars, and gamma-ray bursts [ 97 ]. Nevertheless, galactic cosmic rays could still reach the earth during the solar minimum, the least solar radiation energy period. In the 20th and early 21st centuries, several outbreaks of viral diseases that affected the respiratory system (pneumonia or influenza), namely the Spanish (1918–1919), Asian (1957–1958) and Hong Kong (1968) flu, were documented. Interestingly, the diseases that claimed numerous lives worldwide occurred at the peak of the solar maximum.
Figure 6 illustrates the correlation between the number of sunspots and disease outbreaks from 1975 to 2021, including COVID-19, that began to escalate in December 2019. Under the solar minimum conditions, the spread of Ebola (1976), H5N1 (1997–1998), H1N1 (2009), and COVID-19 (2019-2020) were documented, while the solar maximum phenomenon recorded SARS (2002) and H7N9 (2012–2013) or MERS outbreaks. Nonetheless, solar activity through the production of solar sunspots began to decline since the 22nd solar cycle. Accordingly, further studies are necessary to investigate the influence such solar variations could impart or not on pandemic development.
Despite the findings mentioned above, the sun and cosmic radiations could influence the distribution or outspread of disease-spreading viruses. The rays could kill the viruses via DNA destruction or influence their genetic mutations, which encourage growth and viral evolution. Nevertheless, the connection between radiation and the evolutionary process requires further study by specialists in the field it is become true or not.
The spread of viral diseases transpires naturally in our surroundings and occurs unnoticed by humans. According to records, the spread of pandemic diseases, including the Black Death (fourteenth century) and the Spanish flu (1919), was significantly influenced by the decline and peak of solar activities. Furthermore, in the past 20 years, various diseases related to the influenza virus have been recorded. According to the pattern observed, if all diseases were related to the solar cycle (solar maximum and minimum), the viral diseases would reoccur every 5 to 6 years since they first appeared between 1995 and 2020. Accordingly, the next pandemic might occur around 2024 or 2025 and need to have a proper study for prove these statements. Nonetheless, the activities on the surface of the sun have been weakening since the 23rd solar cycle and it can be proven later after the proper study can be make it.
The beginning of the COVID-19 spread, only several countries with the same winter climate with an average temperature of 5–11 °C and an average humidity rate of 47–79% located at latitudes 30–50 N reported cases. The areas included Wuhan distribution centres in China, the United Kingdom, France, Spain, South Korea, Japan, and the USA (see Fig. 5 ). Other than biological aspects, the higher number of confirmed cases recorded in colder environments was due to the human body secreting less lymphoproliferative hormone, leading to decreased immunogenicity effects and increased risk of infection [ 24 ]. Consequently, the virus could attack and rapidly infect humans during the period [ 1 , 54 ].
The lymphoproliferative response is a protective immune response that plays a vital role in protecting and eradicating infections and diseases. On the other hand, staying in warm conditions or being exposed to more sunlight would lower the risks of infection. According to Asyary and Veruswati [ 98 ], sunlight triggers vitamin D, which increases immunity and increases the recovery rates of infected individuals.
Researchers believe that viruses could survive in the environment for up to 3 to 4 years or even longer. The survival rate of the microorganisms is relatively high, which is related to their biological structures, adaptability on any surfaces, and transmission medium to spread diseases. Viruses possess simple protein structures, namely the spike, membrane, and envelope protein; therefore, when they enter living organisms (such as through the respiratory system), the viruses are easily transmitted.
Once they have entered a host, the viruses duplicate exponentially and swarm the lungs. Subsequently, after the targeted organs, such as the lungs, are invaded, the viruses attack the immune system and create confusion in protective cells to destroy healthy cells. The situation is still considered safe in younger and healthy individuals as their immune systems could differentiate and counter-attack the viruses, curing them. Nonetheless, in elders and individuals with several chronic diseases, most of their protective cells are dead, hence their immune system is forced to work hard to overcome the infection. Pneumonia and death tend to occur when the situation is overwhelming [ 85 ]. Consequently, the viruses are harmful to humans as they could multiply in a short period, enter the blood, and overrun the body.
The coronavirus could attach to surfaces without a host, including door knobs and steel and plastic materials. The microorganisms could survive alone, but virologists have yet to determine how long. If someone touches any surface with the virus, the individual would then be infected. The situation would worsen if the infected person contacted numerous people and became a super spreader. A super spreader does not exhibit any symptoms and continuously transmits the virus without realising it. An infected individual transmits the coronavirus via droplets from coughs or sneezes. Nevertheless, scientists have yet to determine if coronavirus is spread via airborne or droplets, hence requiring thorough evaluation [ 99 ].
The COVID-19 virus mutates over time, and it can be changing any times. Mutations alter the behaviour and genetic structure of the virus, resulting in a new strain. Numerous research have been conducted to procure vaccines and anti-viral medications, but mutations have led to evolutionary disadvantages. The novel strains are more infectious than the original ones. As of November 2020, approximately six new coronavirus strains have been detected, each displaying different transmission behaviours [ 100 ].
Recent studies demonstrated that the mutated viruses exhibit little variability, allowing scientists to produce viable vaccines [ 71 ]. Furthermore, different types of vaccines are manufactured by different countries, which could be advantageous. Currently, most countries also recommend booster doses to attain extra protection after receiving the mandatory two vaccine doses. In same time, the social and physical interactions between humans also necessitate to be aware.
The COVID-19 virus is primarily transmitted through droplets produced by an infected person. Accordingly, physical distancing, a one-metre minimum distance between individuals [ 19 ], and following the SOP might prevent or avoid spreading the disease. Moreover, self-quarantine, school closures, working from home, cancelling large events, limiting gatherings, and avoiding spending long periods in crowded places are essential strategies in enforcing physical distancing at a community level. The policies are essential precautions that could reduce the further spreading of coronavirus and break the chain of transmission.
Government support also need to control the spread of COVID-19 with the strict SOP. The SOP enforcement in public places would enhance adherence to the new practice among the public and the community, aiding in curbing disease transmission. Practising limited meetings and social gatherings, avoiding crowded places, workplace distancing, preventing non-necessary travels of high-risk family members, especially those with chronic disease, and adhering to the recommended SOP could reduce coronavirus outbreaks. Nonetheless, individual awareness is also necessary to achieve COVID-19 spread prevention.
Many researchers are focused on identifying the primary drivers of pandemic outbreaks. Seasonal, temperature, and humidity differences significantly impacted COVID-19 growth rate variations. It is crucial to highlight the potential link between the recurrence of pandemics every 5 years and solar activities, which can influence temperature and humidity variations. Notable variations in COVID-19 mortality rates were observed between northern and southern hemisphere countries, with the former having higher rates. One hypothesis suggests that populations in the northern hemisphere may receive insufficient sunlight to maintain optimal vitamin D levels during winter, possibly leading to higher mortality rates.
The first COVID-19 case was detected in Wuhan, China, which is in the northern hemisphere. The number of cases rapidly propagated in December during the winter season. At the time, the temperature in Wuhan was recorded at 13–18 °C. Accordingly, one theory proposes that the survival and transmission of the coronavirus were due to meteorological conditions, namely temperatures between 13 and 18 °C and 50–80% humidity.
Daily rainfall directly impacts humidity levels. The coronavirus exhibited superior survival rates in cold and dry conditions. Furthermore, transmissible gastroenteritis (TGEV) suspensions and possibly other coronaviruses remain viable longer in their airborne states, which are more reliably collected in low relative humidity than in high humidity. Consequently, summer rains would effectively reduce COVID-19 transmission in southern hemisphere regions.
In southern hemisphere regions, the summer seasons are accompanied by a high average temperature at the end and beginning of the year. Countries with temperatures exceeding 24 °C reported fewer infections. As temperatures rise from winter to summer, virus transmission is expected to decline. Nonetheless, the activities and transmission of the virus were expected to decrease during winter to summer transitions, when the countries would be warmer. The peak intensity of infections strongly depends on the level of seasonal transmissions.
Social distancing plays a critical role in preventing the overload of healthcare systems. Many respiratory pathogens, including those causing mild common cold-like syndromes, show seasonal fluctuations, often peaking in winter. This trend can be attributed to increased indoor crowding, school reopening, and climatic changes during autumn.
The spread of COVID-19 to neighbouring regions can be attributed to population interactions. Migration patterns, such as the movement from northern to southern regions during the warmer months, have significant epidemiological impacts. This trend mirrors the behavior of influenza pandemics where minor outbreaks in spring or summer are often followed by major waves in autumn or winter.
Availability of data and materials
Not applicable.
Abbreviations
Novel coronavirus
Coronavirus disease 2019
Deoxyribonucleic acid
Swine influenza
Influenza A virus subtype H5N1
Asian Lineage Avian Influenza A(H7N9) Virus
Middle East respiratory syndrome
Middle East respiratory syndrome Coronavirus
Particulate matter
Preferred Reporting Items for Systematic Reviews and Meta-Analyses
RepOrting standards for Systematic Evidence Syntheses
Severe Acute Respiratory Syndrome
Severe Acute Respiratory Syndrome Coronavirus
Syndrome coronavirus 2
Systematic literature review
Standard operating procedure
Transmissible gastroenteritis Virus
United States of America
World Health Organization
Apanovich I. Climate and man. Opposition or natural stage of the earth’s evolution? Norwegian journal of development of the international. Science. 2019;26(25):12–27.
Google Scholar
Borah P, Singh MK, Mahapatra S. Estimation of degree-days for different climatic zones of north-East India. Sustain Cities Soc. 2015;14(1):70–81.
Article Google Scholar
Chen D, Chen HW. Using the Köppen classification to quantify climate variation and change: an example for 1901-2010. Environmental Development. 2013;6(1):69–79.
Trenberth KE, Fasullo JT. Global warming due to increasing absorbed solar radiation. Geophys Res Lett. 2009;36
Hauschild MZ, Huijbregts MAJ, Guinée L, Lane J, Fantke P, Zelm v R, et al. Life Cycle Impact Assessment – The Complete World of Life Cycle Assessment; 2015. p. 345.
Book Google Scholar
Nakada LYK, Urban RC. COVID-19 pandemic: environmental and social factors influencing the spread of SARS-CoV-2 in São Paulo. Brazil Environmental Science and Pollution Research. 2021;28(30):40322–8.
Article CAS PubMed Google Scholar
Lagtayi, R., Lairgi, L., Daya, A., & Khouya, A. (2021). The impact of the average temperature, humidity, wind speed, altitude and population density on daily COVID-19 infections’ evolution. January, 9094.
Majumder MS, Liu D, Poirier C, Mandl KD, Lipsitch M, The MS. The role of absolute humidity on transmission rates of the COVID-19 outbreak; 2020.
Wiersinga WJ, Rhodes A, Cheng AC, Peacock SJ, Prescott HC. Pathophysiology, transmission, diagnosis, and treatment of coronavirus disease 2019 (COVID-19): A review. JAMA - Journal of the American Medical Association. 2020;324(8):782–93.
Zhang X, Maggioni V, Houser P, Xue Y, Mei Y. The impact of weather condition and social activity on COVID-19 transmission in the United States. J Environ Manag. 2022;302:114085.
Article CAS Google Scholar
Mäkinen TM, Juvonen R, Jokelainen J, Harju TH, Peitso A, Bloigu A, et al. Cold temperature and low humidity are associated with increased occurrence of respiratory tract infections. Respir Med. 2009;103(3):456–62.
Article PubMed Google Scholar
Carvalho FRS, Henriques DV, Correia O, Schmalwieser AW. Potential of solar UV radiation for inactivation of Coronaviridae family. Photochem Photobiol. 2021;97:213–20.
Ali I, Alharbi OM. COVID-19: disease, management, treatment, and social impact. Sci Total Environ. 2020;728:138861.
Article CAS PubMed PubMed Central Google Scholar
Jin Y, Yang H, Ji W, Wu W, Chen S, Zhang W, et al. Virology, epidemiology, pathogenesis, and control of covid-19. Viruses. 2020;12(4):1–17.
Sohrabi C, Alsafi Z, O’Neill N, Khan M, Kerwan A, Al-Jabir A, et al. World health organization declares global emergency: A review of the 2019 novel coronavirus (COVID-19). Int J Surg. 2020;76:71–6.
Article PubMed PubMed Central Google Scholar
Wang J, Tang K, Feng K, Lv W. High temperature and high humidity reduce the transmission of COVID-19; 2020a.
Wang L, Duan Y, Zhang W, Liang J, Xu J, Zhang Y, et al. Epidemiologic and clinical characteristics of 26 cases of covid-19 arising from patient-to-patient transmission in Liaocheng, China. Clinical Epidemiology. 2020b;12:387–91.
Xie J, Zhu Y. Science of the Total environment association between ambient temperature and COVID-19 infection in 122 cities from China. Sci Total Environ. 2020;724:138201.
World Health Organization. (2020a). Director-General’s opening remarks at the media briefing on COVID-19-10April 2020. https://www.who.int/dg/speeches/detail/who-director-general-s-opening-remarks-at-the-media-briefing-on-covid-19—10- april-2020.
Bostock B. South Korea is testing 200,000 members of a doomsday church linked to more than 60% of its coronavirus cases; 2020.
World Health Organization. (2020b). Health topics/coronavirus. https://www.who.int/health-topics/coronavirus#tab=tab_1.
Menhat M, Mohd Zaideen IM, Yusuf Y, Salleh NHM, Zamri MA, Jeevan J. The impact of Covid-19 pandemic: A review on maritime sectors in Malaysia. Ocean Coast Manag. 2021;209:105638.
Byun WS, Heo SW, Jo G, Kim JW, Kim S, Lee S, et al. Is coronavirus disease (COVID-19) seasonal? A critical analysis of empirical and epidemiological studies at global and local scales. Environ Res. 2021;196:110972.
Dhakal P, Pokhrel P, B. Seasonal variation and COVID-19 pandemic in Nepal. Nepal Medical Journal. 2020;3(2):77–80.
Mehmet Ş. Science of the Total environment impact of weather on COVID-19 pandemic in Turkey. 728; 2020.
Xu H, Yan C, Fu Q, Xiao K, Yu Y, Han D, et al. Science of the Total environment possible environmental effects on the spread of COVID-19 in China. Sci Total Environ. 2020;731:139211.
Rosario DKA, Mutz YS, Bernardes PC, Conte-Junior CA. Relationship between COVID-19 and weather: case study in a tropical country. Int J Hyg Environ Health. 2020;229:113587.
Wang J, Tang K, Feng K, Lin X, Lv W, Chen K, et al. Impact of temperature and relative humidity on the transmission of COVID-19: A modelling study in China and the United States. BMJ Open. 2021;11(2):1–16.
Wu Y, Jing W, Liu J, Ma Q, Yuan J, Wang Y, et al. Effects of temperature and humidity on the daily new cases and new deaths of COVID-19 in 166 countries. Sci Total Environ. 2020;729:1–7.
Casanova LM, Jeon S, Rutala WA, Weber DJ, Sobsey MD. Effects of air temperature and relative humidity on coronavirus survival on surfaces. Appl Environ Microbiol. 2010;76(9):2712–7.
Islam N, Bukhari Q, Jameel Y, Shabnam S, Erzurumluoglu AM, Siddique MA, et al. COVID-19 and climatic factors: A global analysis. Environ Res. 2021;193:110355.
Diao Y, Kodera S, Anzai D, Gomez-Tames J, Rashed EA, Hirata A. Influence of population density, temperature, and absolute humidity on spread and decay durations of COVID-19: A comparative study of scenarios in China, England, Germany, and Japan. One Health. 2021;12:100203.
Haddaway NR, Macura B, Whaley P, Pullin AS. ROSES reporting standards for systematic evidence syntheses: pro forma, flow-diagram and descriptive summary of the plan and conduct of environmental systematic reviews and systematic maps. Environ Evid. 2018;7(1):4–11.
Sharif N, Dey SK. Impact of population density and weather on COVID-19 pandemic and SARS-CoV-2 mutation frequency in Bangladesh. Epidemiol Infect. 2021:1–10.
Kraus S, Breier Dasí-Rodríguez S. El arte de elaborar una revisión bibliográfica sistemática en la investigación sobre el espíritu empresarial. Int Entrep Manag J. 2020;16:1023–42.
Xiao Y, Watson M. Guidance on conducting a systematic literature review. J Plan Educ Res. 2019;39(1):93–112.
Gusenbauer M, Haddaway NR. Which academic search systems are suitable for systematic reviews or meta-analyses? Evaluating retrieval qualities of Google scholar, PubMed, and 26 other resources. Res Synth Methods. 2020;11(2):181–217.
Halevi G, Moed H, Bar-Ilan J. Suitability of Google scholar as a source of scientific information and as a source of data for scientific evaluation—review of the literature. Journal of Informetrics. 2017;11(3):823–34.
Haddaway NR, Collins AM, Coughlin D, Kirk S. The role of google scholar in evidence reviews and its applicability to grey literature searching. PLoS One. 2015;10(9):1–17.
Littlewood C, Chance-Larsen K, McLean S. Quality appraisal as a part of the systematic review. International Journal of Physiotherapy and Rehabilitation. 2010;1(1):53–8.
Vaismoradi M, Turunen H, Bondas T. Content analysis and thematic analysis: implications for conducting a qualitative descriptive study. Nurs Health Sci. 2013;15(3):398–405.
Braun V, Clarke V. Using thematic analysis in psychology. Qual Res Psychol. 2006;3:77–101.
Ahmadi M, Sharifi A, Dorosti S, Jafarzadeh Ghoushchi S, Ghanbari N. Investigation of effective climatology parameters on COVID-19 outbreak in Iran. Sci Total Environ. 2020;729
Gupta A, Banerjee S, Das S. Significance of geographical factors to the COVID-19 outbreak in India. Modeling Earth Systems and Environment. 2020;6(4):2645–53.
Pequeno P, Mendel B, Rosa C, Bosholn M, Souza JL, Baccaro F, et al. Air transportation, population density and temperature predict the spread of COVID-19 in Brazil. PeerJ. 2020;2020(6):1–15.
Hoyle F, Wickramasinghe NC. Sunspots and influenza [6]. Nature. 1990;343(6256):304.
Wickramasinghe NC, Rocca MC, Tokoro G, Temple R. Journal of infectious diseases. Scienctific Research and Community. 2020;1(4):1–10.
Wickramasinghe NC, Steele EJ, Wainwright M, Tokoro G, Fernando M, Qu J. Sunspot cycle minima and pandemics : A case for vigilance at the present time. Journal of Astrobiology & Outreach. 2017;5:2332–519.
Wickramasinghe NC, Qu J. Are we approaching a new influenza pandemic. Virol Curr Res. 2018;2(107):2.
Guan W, Ni Z, Hu Y, Liang W, Ou C, He J, et al. Disease 2019 in China; 2020.
Bashir MF, Ma B, Bilal K, Bashir MA, Tan D, Bashir M. Correlation between climate indicators and COVID-19 pandemic in New York, USA. Sci Total Environ. 2020;728:138835.
Cucinotta D, Vanelli M. WHO declares COVID-19 a pandemic. Acta Biomed. 2020;91:157–60.
PubMed PubMed Central Google Scholar
Lin C, Lau AKH, Fung JCH, Guo C, Chan JWM, Yeung DW, et al. A mechanism-based parameterisation scheme to investigate the association between transmission rate of COVID-19 and meteorological factors on plains in China. Sci Total Environ. 2020;737:140348.
Coşkun H, Yıldırım N, Gündüz S. The spread of COVID-19 virus through population density and wind in Turkey cities. Sci Total Environ. 2021;751
Yang HY, Lee JKW. The impact of temperature on the risk of covid-19: A multinational study. Int J Environ Res Public Health. 2021;18(8)
Candido DD, Watts A, Abade L, Kraemer MUG, Pybus OG, Croda J, et al. Routes for COVID-19 importation in Brazil. Journal of Travel Medicine. 2020;27(3):1–3.
Hardy JP, Groffman PM, Fitzhugh RD, Henry KS, Welman AT, Demers JD, et al. Snow depth manipulation and its influence on soil frost and water dynamics in a northern hardwood forest. Biogeochemistry. 2001;56(2):151–74.
Gunthe SS, Swain B, Patra SS, Amte A. On the global trends and spread of the COVID-19 outbreak: preliminary assessment of the potential relation between location-specific temperature and UV index. Journal of Public Health (Germany). 2020:1–10.
Rosenthal PJ, et al. COVID-19: shining the light on Africa. Am J Trop Med Hyg. 2020;102(6):1145–8.
Kraemer MUG, et al. The effect of human mobility and control measures on the COVID-19 epidemic in China. Science. 2020;368:493–7.
Dalziel BD, Kissler S, Gog JR, Viboud C, Bjørnstad ON, Metcalf CJE, et al. Urbanization and humidity shape the intensity of influenza epidemics in U.S. cities. Science. 2018;362:75–9.
Sahoo PK, Powell MA, Mittal S, Garg VK. Is the transmission of novel coronavirus disease (COVID-19) weather dependent? J Air Waste Manage Assoc. 2020;70(11):1061–4.
Selcuk M, Gormus S, Guven M. Impact of weather parameters and population density on the COVID-19 transmission: evidence from 81 provinces of Turkey. Earth Syst Environ. 2021;5(1):87–100.
Abraham J, Turville C, Dowling K, Florentine S. Does climate play any role in covid-19 spreading?—an Australian perspective. Int J Environ Res Public Health. 2021;18(17)
Sehra ST, Salciccioli JD, Wiebe DJ, Fundin S, Baker JF. Maximum daily temperature, precipitation, ultraviolet light, and rates of transmission of severe acute respiratory syndrome coronavirus 2 in the United States. Clin Infect Dis. 2020;71(9):2482–7.
CAS PubMed Google Scholar
Rubin D, Huang J, Fisher BT, Gasparrini A, Tam V, Song L, et al. Association of Social Distancing, population density, and temperature with the instantaneous reproduction number of SARS-CoV-2 in counties across the United States. JAMA Netw Open. 2020;3(7):1–12.
Comunian S, Dongo D, Milani C, Palestini P. Air pollution and covid-19: The role of particulate matter in the spread and increase of covid-19’s morbidity and mortality. Int J Environ Res Public Health. 2020;17(12):1–22.
Tosepu R, Gunawan J, Effendy DS, Ahmad LOAI, Lestari H, Bahar H, et al. Correlation between weather and Covid-19 pandemic in Jakarta, Indonesia. Sci Total Environ. 2020;725
Chen and Shakhnovich. Thermal adaptation of viruses and Bacteria. Biophys J. 2010;98:1109–18.
Martins LD, da Silva I, Batista WV, Andrade MF, Freitas ED, Jorge Alberto Martins JA. How socio-economic and atmospheric variables impact COVID-19 and influenza outbreaks in tropical and subtropical regions of Brazil. Environ Res. 2020;191:110184.
Kulkarni H, Khandait H, Narlawar UW, Rathod P, Mamtani M. Independent association of meteorological characteristics with initial spread of Covid-19 in India. Sci Total Environ. 2021;764:142801.
Menebo MM. Science of the Total environment temperature and precipitation associate with Covid-19 new daily cases : A correlation study between weather and Covid-19 pandemic in. Sci Total Environ. 2020;737:139659.
Gupta S, Patel KK. Global Epidemiology of First 90 Days into COVID-19 Pandemic :Disease Incidence , Prevalence , Case Fatality Population Density, Urbanisation. J Health Manag. 2020;22(2):117–28.
Haque SE, Rahman M. Association between temperature, humidity, and COVID-19 outbreaks in Bangladesh. Environ Sci Pol. 2020;114:253–5.
Sharma P, Singh AK, Agrawal B, Sharma A. Correlation between weather and COVID-19 pandemic in India: an empirical investigation. J Public Aff. 2020;20(4)
Fu S, Wang B, Zhou J, Xu X, Liu J, Ma Y, et al. Meteorological factors, governmental responses and COVID-19: evidence from four European countries. Environ Res. 2021;194:110596.
Mecenas P, Bastos RT, Vallinoto AC, Normando D. Effects of temperature and humidity on the spread of COVID-19: A systematic review. PLoS One. 2020;15:1–21.
Malki Z, Atlam ES, Hassanien AE, Dagnew G, Elhosseini MA, Gad I. Association between weather data and COVID-19 pandemic predicting mortality rate: machine learning approaches. Chaos, Solitons Fractals. 2020;138:110137.
Sasikumar K, Nath D, Nath R, Chen W. Impact of extreme hot climate on COVID-19 outbreak in India. GeoHealth. 2020;4(12)
Kodera S, Rashed EA, Hirata A. Correlation between COVID-19 morbidity and mortality rates in Japan and local population density, temperature, and absolute humidity. Int J Environ Res Public Health. 2020;17(15):1–14.
Sobral MFF, Duarte GB, da Penha Sobral AIG, Marinho MLM, de Souza Melo A. Association between climate variables and global transmission oF SARS-CoV-2. Sci Total Environ. 2020;729:138997.
Patel SK, Pathak M, Tiwari R, Yatoo MI, Malik YS. A vaccine is not too far for COVID-19 coronavirus pandemic A vaccine is not too far for COVID-19. May; 2020.
Nicola M, Neill NO, Sohrabi C, Khan M, Agha M, Agha R. Evidence based management guideline for the COVID-19 pandemic - review article. Int J Surg. 2020;77:206–16.
Atangana E, Atangana A. Facemasks simple but powerful weapons to protect against COVID-19 spread: can they have sides effects? Results in Physics. 2020;19:103425.
Sarmadi M, Moghanddam VK, Dickerson AS, Martelletti L. Association of COVID-19 distribution with air quality, sociodemographic factors, and comorbidities: an ecological study of US states. Air Qual Atmos Health. 2021;14(4):455–65.
Chung CJ, Nazif TM, Wolbinski M, Hakemi E, Lebehn M, Brandwein R, et al. The restructuring of structural heart disease practice during The Covid-19 pandemic. J Am Coll Cardiol. 2020; InPress
Bukhari Q, Massaro JM, D’agostino RB, Khan S. Effects of weather on coronavirus pandemic. Int J Environ Res Public Health. 2020;17(15):1–12.
Sun C, Zhai Z. The efficacy of social distance and ventilation effectiveness in preventing COVID-19 transmission. Sustain Cities Soc. 2020;62:102390.
Nair N, Selvaraj P. Using a cultural and social identity lens to understand pandemic responses in the US and India. Int J Cross-cult Manag. 2021;21(3):545–68.
Cetron M, Landwirth J. Public health and ethical considerations in planning for quarantine. Yale J Biol Med. 2005;78(5):325–30.
Jernigan DB. Update: public health response to the coronavirus disease 2019 outbreak—United States, February 24, 2020. MMWR. Morbidity and mortality weekly report, 69. 2020.
Stasi C, Fallani S, Voller F, Silvestri C. Treatment for COVID-19: an overview. Eur J Pharmacol. 2020;889:173644.
Foad CAKK, Xun N, Pejman J, Nataraj RC. Nonlinear dynamic analysis of an epidemiological model for COVID-19 including public behavior and government action. Nonlinear Dynamics. 2020;101(3):1545–59.
Sun Z, Zhang H, Yang Y, Wan H, Wang Y. Science of the Total environment impacts of geographic factors and population density on the COVID-19 spreading under the lockdown policies of China. Sci Total Environ. 2020;746(666):141347.
Abdullah S, Mansor AA, Napi NNLM, Mansor WNW, Ahmed AN, Ismail M, et al. Air quality status during 2020 Malaysia movement control order (MCO) due to 2019 novel coronavirus (2019-nCoV) pandemic. Sci Total Environ. 2020;729:139022.
Menhat M, Yusuf Y. Factors influencing the choice of performance measures for the oil and gas supply chain - exploratory study. IOP Conference Series: Materials Science and Engineering. 2018;342(1)
Ćirkovića MM, Vukotića B. Long-term prospects: mitigation of supernova and gamma-ray burst threat to intelligent beings. Acta Astronautica. 2016;129:438–46.
Asyary A, Veruswati M. Science of the Total environment sunlight exposure increased Covid-19 recovery rates : A study in the central pandemic area of Indonesia. Sci Total Environ. 2020;729:139016.
Jayaweeraa M, Pererab H, Gunawardanaa B, Manatungea J. Transmission of COVID-19 virus by droplets and aerosols: A critical review on the unresolved dichotomy. Environ Res. 2020;188:1–18.
Leung K, Shum MHH, Leung GM, Lam TTY, Wu JT. Early transmissibility assessment of the N501Y mutant strains of SARS-CoV-2 in the United Kingdom, October to November 2020. Euro Surveill. 2020;26(1)
Download references
Acknowledgements
The authors would also like to acknowledge the Editors and an anonymous reviewer, who contributed immensely to improving the quality of this publication and a special thanks to Muhammad Hafiy Nauwal Effi Helmy, that contributed an excellent idea through singing during the COVID-19 lockdown period.
This research did not receive any specific grant from funding agencies in the public, commercial, or not-for-profit sectors.
Author information
Authors and affiliations.
Faculty of Maritime Studies, Universiti Malaysia Terengganu, 21030, Kuala Nerus, Terengganu, Malaysia
Masha Menhat
Institute of Oceanography and Environment, Universiti Malaysia Terengganu, 21030, Kuala Nerus, Terengganu, Malaysia
Effi Helmy Ariffin, Junainah Zakaria & Mohd Fadzil Akhir
Faculty of Science and Marine Environment, Universiti Malaysia Terengganu, 21030, Kuala Nerus, Terengganu, Malaysia
Wan Shiao Dong & Aminah Ismailluddin
Institute for Social Science Studies, Universiti Putra Malaysia, 43400, Serdang, Selangor, Malaysia
Hayrol Azril Mohamed Shafril
Social, Environmental and Developmental Sustainability Research Center, Faculty of Social Sciences and Humanities, Universiti Kebangsaan Malaysia, 43600, Bangi, Selangor, Malaysia
Mahazan Muhammad
Institute of Geology Malaysia, Board of Geologists, 62100, Putrajaya, Malaysia
Ahmad Rosli Othman
Executive Office, Proofreading By A UK PhD, 51-1, Biz Avenue II, 63000, Cyberjaya, Malaysia
Thavamaran Kanesan
Faculty of Engineering, Universiti Malaya, 50603, Kuala Lumpur, Malaysia
Suzana Pil Ramli
Faculty of Applied Sciences, Uva Wellassa University, Badulla, 90000, Sri Lanka
Amila Sandaruwan Ratnayake
You can also search for this author in PubMed Google Scholar
Contributions
All authors have been involved in writing this editorial and contributing to the review of the manuscript. MM and EHA contribute to conceptualization. IA and ARO have made the figure.
Corresponding author
Correspondence to Effi Helmy Ariffin .
Ethics declarations
Ethics approval and consent to participate.
Not Applicable.
Consent for publication
Competing interests.
The authors declare that they have no competing interests.
Additional information
Publisher’s note.
Springer Nature remains neutral with regard to jurisdictional claims in published maps and institutional affiliations.
Rights and permissions
Open Access This article is licensed under a Creative Commons Attribution 4.0 International License, which permits use, sharing, adaptation, distribution and reproduction in any medium or format, as long as you give appropriate credit to the original author(s) and the source, provide a link to the Creative Commons licence, and indicate if changes were made. The images or other third party material in this article are included in the article's Creative Commons licence, unless indicated otherwise in a credit line to the material. If material is not included in the article's Creative Commons licence and your intended use is not permitted by statutory regulation or exceeds the permitted use, you will need to obtain permission directly from the copyright holder. To view a copy of this licence, visit http://creativecommons.org/licenses/by/4.0/ . The Creative Commons Public Domain Dedication waiver ( http://creativecommons.org/publicdomain/zero/1.0/ ) applies to the data made available in this article, unless otherwise stated in a credit line to the data.
Reprints and permissions
About this article
Cite this article.
Menhat, M., Ariffin, E.H., Dong, W.S. et al. Rain, rain, go away, come again another day: do climate variations enhance the spread of COVID-19?. Global Health 20 , 43 (2024). https://doi.org/10.1186/s12992-024-01044-w
Download citation
Received : 27 July 2023
Accepted : 22 April 2024
Published : 14 May 2024
DOI : https://doi.org/10.1186/s12992-024-01044-w
Share this article
Anyone you share the following link with will be able to read this content:
Sorry, a shareable link is not currently available for this article.
Provided by the Springer Nature SharedIt content-sharing initiative
- Coronavirus
- Solar radiation
- Temperature
- Social distancing
Globalization and Health
ISSN: 1744-8603
- Submission enquiries: [email protected]
- General enquiries: [email protected]
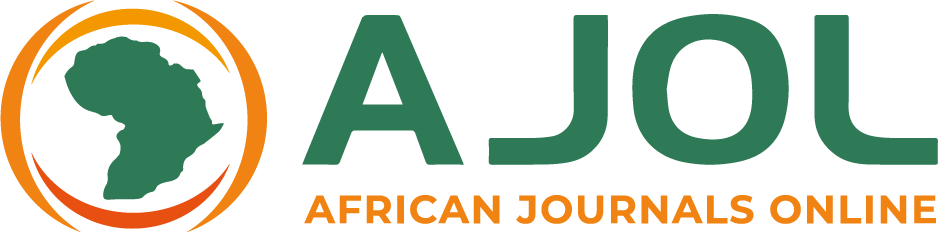
East African Medical Journal Journal / East African Medical Journal / Vol. 101 No. 2 (2024) / Articles (function() { function async_load(){ var s = document.createElement('script'); s.type = 'text/javascript'; s.async = true; var theUrl = 'https://www.journalquality.info/journalquality/ratings/2405-www-ajol-info-eamj'; s.src = theUrl + ( theUrl.indexOf("?") >= 0 ? "&" : "?") + 'ref=' + encodeURIComponent(window.location.href); var embedder = document.getElementById('jpps-embedder-ajol-eamj'); embedder.parentNode.insertBefore(s, embedder); } if (window.attachEvent) window.attachEvent('onload', async_load); else window.addEventListener('load', async_load, false); })();
Article sidebar, article details, main article content, treatment outcomes and associated epidemiological characteristics among patients hospitalized with covid-19 in critical care and isolation wards at a tertiary hospital in kiambu county, kenya, n.o. ochoki, g.n. kilonzo, e.g. mwangi, g.w. mbuthia, c.o. omondi, r.n. gitonga.
Background : COVID-19 was a novel infection whose outcomes were not well established to be associated with any factors during the start of the pandemic that it caused globally.
Objective : To establish epidemiological characteristics associated with mortality among patients hospitalized with covid-19 at a tertiary hospital in Kiambu County Kenya
Methods : This was a retrospective Analytical cross-sectional study done at Avenue Hospital Isolation and Intensive care wards. Polymerase chain reaction (PCR) positively identified Covid-19 adult patients who were admitted were recruited into the study. The mortality rate from COVID-19 infection and epidemiological characteristics associated with the mortalities among the COVID-19 hospitalized patients were the measures of outcomes being investigated.
Results : A total of 154 patients were recruited in the study. Almost two thirds of the patients had comorbidities (n=96; 62.3%). Out of 154 patients, 23.4% (n=36) died and 76.6% n= (118) were discharged alive. Kaplan Meier statistics revealed, the median survival time was 22 days (95% CI: 16.626 - 27.374) among patents who died. The epidemiological factor predicting death of a patient after adjusting for odds was increase in age (AOR: 1.138; 95% CI: 1.009 - 1.285; p-value- 0.036).
Conclusion and Recommendations : Majority of the patients admitted with COVID-19 were discharged alive compared to those with mortalities. The mortalities which occurred were associated with an increase in age among the patients. The study recommends healthcare professionals to prioritize older demographic patients diagnosed with COVID-19 during triage and routine care.
AJOL is a Non Profit Organisation that cannot function without donations. AJOL and the millions of African and international researchers who rely on our free services are deeply grateful for your contribution. AJOL is annually audited and was also independently assessed in 2019 by E&Y.
Your donation is guaranteed to directly contribute to Africans sharing their research output with a global readership.
- For annual AJOL Supporter contributions, please view our Supporters page.
Journal Identifiers
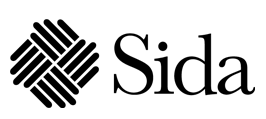
Disclaimer: Early release articles are not considered as final versions. Any changes will be reflected in the online version in the month the article is officially released.
Volume 30, Number 6—June 2024
Electronic Health Record–Based Algorithm for Monitoring Respiratory Virus–Like Illness
Suggested citation for this article
Viral respiratory illness surveillance has traditionally focused on single pathogens (e.g., influenza) and required fever to identify influenza-like illness (ILI). We developed an automated system applying both laboratory test and syndrome criteria to electronic health records from 3 practice groups in Massachusetts, USA, to monitor trends in respiratory viral–like illness (RAVIOLI) across multiple pathogens. We identified RAVIOLI syndrome using diagnosis codes associated with respiratory viral testing or positive respiratory viral assays or fever. After retrospectively applying RAVIOLI criteria to electronic health records, we observed annual winter peaks during 2015–2019, predominantly caused by influenza, followed by cyclic peaks corresponding to SARS-CoV-2 surges during 2020–2024, spikes in RSV in mid-2021 and late 2022, and recrudescent influenza in late 2022 and 2023. RAVIOLI rates were higher and fluctuations more pronounced compared with traditional ILI surveillance. RAVIOLI broadens the scope, granularity, sensitivity, and specificity of respiratory viral illness surveillance compared with traditional ILI surveillance.
Respiratory viral illnesses place an enormous burden on human health and the healthcare system ( 1 – 3 ). Although multiple pathogenic respiratory viruses circulate, often simultaneously, public health has traditionally dedicated most of its attention to monitoring trends in laboratory-confirmed influenza and influenza-like illness (ILI). Illness and death associated with seasonal respiratory syncytial virus (RSV) spikes, the SARS-CoV-2 pandemic, and occasional clusters of infection from other respiratory pathogens, however, illustrate the importance of expanding monitoring to include all respiratory viral–like illness activity. Relying on laboratory testing alone will not accomplish this goal because most persons with respiratory viral illnesses do not seek care, many who do seek care are not tested, and not everyone tested is tested for all respiratory viruses.
Public health agencies have traditionally relied on syndromic surveillance to monitor conditions for which testing rates are low and variable ( 4 ). The Centers for Disease Control and Prevention’s outpatient Influenza-like Illness Surveillance Network and emerging systems designed to monitor COVID-19–like illness are prime examples ( 5 – 9 ). However, syndromic surveillance systems tend to provide little or no information about which particular pathogens are circulating, and most jurisdictions require fever to define ILI, a requirement that increases specificity but lowers sensitivity (fever occurs in fewer than half of persons with laboratory-confirmed influenza) ( 10 ). Surveillance focusing on single pathogens (e.g., influenza, SARS-CoV-2), viral testing alone, or syndromic definitions alone provides an incomplete picture of respiratory illness activity and can miss critical trends and developments ( 11 , 12 ). Extending surveillance to include multiple pathogens, using both laboratory testing and syndromes, and decreasing reliance on fever as a gatekeeper symptom are necessary to provide public health agencies and healthcare institutions with the data needed to improve situational awareness for planning, resource use, internal and external communications, and targeted prevention activities.
To regularly monitor overall respiratory viral illness activity associated with multiple pathogens, we developed an integrated surveillance strategy using a combination of laboratory and syndromic indicators, incorporating logic to identify the relative contributions of different individual pathogens. We describe our data-driven approach to developing a routine, automated respiratory virus-like illness (RAVIOLI) algorithm for syndromic surveillance in Massachusetts using live electronic health record (EHR) data drawn from 3 large practice groups. Our work was performed as public health surveillance and therefore not subject to institutional review board oversight.
We used the Electronic Medical Record Support for Public Health (ESP, https://www.esphealth.org ) public health surveillance platform to develop the RAVIOLI algorithm. ESP is open-source software that uses automated daily extracts of EHR data to identify and report conditions of public health interest to health departments ( 13 – 17 ). ESP maps raw data to common terms and then applies algorithms to identify conditions using diagnosis codes, prescriptions, laboratory tests, and vital signs. In Massachusetts, ESP is used for automated reporting of infectious disease cases to the Massachusetts Department of Public Health, aggregate reporting of chronic diseases, and continuum-of-care assessments ( 18 – 21 ).
Three multisite clinical practice groups that use ESP for infectious disease reporting, Atrius Health, Cambridge Health Alliance, and Boston Medical Center, contributed data for our project. Atrius Health ( https://www.atriushealth.org ) is an ambulatory care group with >30 locations in eastern Massachusetts that provides clinical services for a population of ≈700,000. Cambridge Health Alliance ( https://www.challiance.org ) is a safety-net system that provides ambulatory and inpatient care to >140,000 patients in communities north of Boston. Boston Medical Center ( https://www.bmc.org ) is a 514-bed academic medical center and safety-net hospital that provides ambulatory and inpatient care to ≈220,000 persons. We combined data from those 3 sites for this analysis.
We sought to develop an evidence-based set of diagnosis codes to identify respiratory virus–like illnesses and assess whether a subset of those codes might be predictive of specific pathogens. To identify codes associated with respiratory viral illness syndrome, we identified all patients tested for respiratory viruses ( Table 1 ) during October 3, 2015–July 30, 2022. Among patients who tested positive for > 1 virus, we identified all International Classification of Diseases, 10th Revision (ICD-10), diagnosis codes recorded within 2 days before or after the specimen collection date. For patients without a recorded specimen collection date, we used the test order date; if that was unavailable, we used the result date. We manually removed ICD-10 codes unrelated to respiratory viral illness (e.g., trauma, cancer, chronic disease management). The list of >7,000 excluded codes is available upon request from the authors.
We calculated the positive predictive value (PPV) for each ICD-10 code associated with positive respiratory virus test results. We also calculated the PPV for measured temperature >100°F within 2 days before or after a positive respiratory virus test. We calculated the PPV for each ICD-10 code and fever as the number of encounters with the diagnosis code within 2 days of a positive test divided by the total number of times the diagnosis code occurred across all clinical encounters during the study period. We defined a clinical encounter as a patient receiving a relevant diagnosis code, immunization, vital sign measure, laboratory test, or prescription.
We included in the final algorithm diagnosis codes with a PPV ≥10% for any respiratory virus (all viruses combined) or for a specific individual respiratory virus. We also included encounters with positive respiratory virus tests in the total count of respiratory virus encounters as well as in virus-specific categories of RAVIOLI. We counted each viral encounter only 1 time if the patient had both a positive respiratory virus assay result and > 1 suggestive diagnosis code. We classified measured fever alone and diagnosis codes with a PPV of ≥10% for any positive respiratory virus test but <10% for any specific respiratory virus in a category referred to as nonspecific for respiratory viral illness syndrome. In summary, we categorized positive cases within RAVIOLI as virus-specific (e.g., influenza, adenovirus), based on a positive test or a diagnosis code with a PPV ≥10% for the specific virus, or nonspecific, based on fever or a diagnosis code with a PPV ≥10% for any positive test of interest.
To better understand the underlying data included in the final RAVIOLI algorithm, we examined the proportion of patients in each virus-specific category of the algorithm with a positive laboratory test and the proportion of patients in the nonspecific category with a fever. We generated weekly counts during October 3, 2015–January 13, 2024, for clinical encounters with patients meeting the RAVIOLI algorithm, overall and stratified by the probable etiology when possible. For comparison, we also identified the proportion of patients that met the definition of ILI: fever and a diagnosis code for any influenza-like symptom or diagnosis; fever was identified by either a measured fever >100°F or diagnosis code for fever ( Appendix Table 1 ).
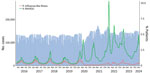
Figure 1 . Numbers of patients with a clinical encounter for respiratory virus–like illness and the percentages that met the requirements for influenza-like illness versus those of the RAVIOLI algorithm for monitoring respiratory...
Forty-two diagnosis codes ( Table 2 ) and measured fever (>100°F) had a PPV ≥10% for either any positive respiratory virus test (nonspecific) or > 1 virus-specific positive test; those diagnosis codes and fever are included in the RAVIOLI algorithm. We recorded weekly counts of patients with clinical encounters and calculated the proportion that met the definition for RAVIOLI overall (diagnosis code, fever, or positive respiratory virus test) and, for comparison, the proportion that met the ILI criteria ( Figure 1 ). The percentage of encounters that met the RAVIOLI algorithm showed clear seasonal trends of annual winter spikes during 2015–2019 followed by periodic increases during spring 2020–early 2024, corresponding to emergence or surges of SARS-CoV-2, RSV, and influenza in Massachusetts. RAVIOLI was identified in a much larger proportion of encounters than ILI after March 2020 and, at times (e.g., fall 2021, August–November 2023), ILI did not detect an increase in respiratory virus illness while RAVIOLI did.
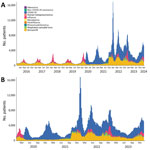
Figure 2 . Numbers of patients that met the requirements for the RAVIOLI algorithm for monitoring respiratory virus–like illness, by pathogen category and week, Massachusetts, USA, October 2015–January 2024. A) October 2015–January 2024;...
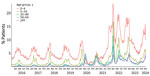
- Figure 3 . Percentage of patients meeting the RAVIOLI algorithm for monitoring respiratory virus–like illness, by age group, Massachusetts, USA, October 2015–January 2024.
We estimated weekly counts of patients with clinical encounters meeting the RAVIOLI algorithm stratified by encounters with virus-specific or nonspecific encounters without a classified virus. We calculated those data for the full study period, October 2015–January 2024 ( Figure 2 , panel A), and for January 2020–January 2024 ( Figure 2 , panel B). Before March 2020, most RAVIOLI encounters came from the influenza or unclassified categories. SARS-CoV-2 subsequently dominated until fall 2021, when the nonspecific category reemerged, along with influenza and RSV. When we examined trends by patient age groups, the highest proportion of encounters that met the RAVIOLI algorithm were among children 0–4 years of age, followed by young persons 5–24 years of age ( Figure 3 ).
Data from January 2023–January 2024 show the proportions of patients in the COVID-19, influenza, and RSV categories with a positive laboratory test versus diagnosis code, as well as the proportion in the nonspecific with fever category ( Appendix Table 2 ). The proportion with a positive test varied by virus and time; patients in the COVID-19 category were least likely and those in the RSV category most likely to have a positive laboratory test. Among patients in the nonspecific category, one third or fewer had evidence of fever, and most were identified by a diagnosis code. We also determined the proportion of RAVIOLI patients identified on the basis of > 1 positive laboratory test, diagnosis code, or fever during January 2021–January 2024 ( Appendix Figure 1 ); RAVIOLI patients can meet > 1 criterion (e.g., have both a positive laboratory test and a diagnosis code). Diagnosis codes were the most common element contributing to identification in most weeks, followed by positive laboratory tests and fever.
Respiratory viruses continue to impose a high burden on patients, healthcare providers, and society, and multiple pathogens, including SARS-CoV-2, influenza, RSV, and others, contribute to the burden of respiratory illnesses. Both healthcare providers and public health agencies therefore have an interest in having access to timely and granular data on trends in respiratory viral illnesses and contributing pathogens. We developed an EHR-based algorithm for integrated surveillance of respiratory virus illness syndromes and associated pathogens using historical data to identify diagnosis codes and other characteristics of healthcare visits most predictive of confirmed respiratory viral illnesses. The RAVIOLI algorithm comprises positive laboratory tests, evidence-based diagnosis codes, and measured fever.
We have implemented RAVIOLI surveillance within the ESP automated public health surveillance platform to provide the Massachusetts Department of Public Health and participating practices with weekly reports on RAVIOLI incidence and contributing pathogens. RAVIOLI provides the department and practices with granular insight into evolving trends in respiratory viral illness rates that both retains the best features of traditional syndromic surveillance (capacity to monitor changes in disease incidence in near real time regardless of whether persons get tested) and simultaneously broadens the scope of surveillance to include multiple pathogens, not just influenza and SARS-CoV-2. The data provide insight into the relative proportions of contributing pathogens across multiple clinical facilities using both test results and diagnosis codes to identify organisms.
When implemented well, syndromic surveillance provides a picture of the frequency, intensity, and trends in indicators of infectious and noninfectious conditions at local and extended scales. Integrating available viral pathogen test results, even if only in a subset of the population under surveillance, as we have done with the RAVIOLI algorithm, can add information about what is or is not contributing to observed increases in respiratory viral activity. Although Influenza-like Illness Surveillance Network–defined and COVID-like illness surveillance have been critical components for monitoring influenza and COVID-19 activity, reliance on fever as a required component of syndromic definitions is problematic because fever occurs only in a minority of laboratory-confirmed influenza and SARS-CoV-2 cases ( 22 – 24 ). Syndromic surveillance algorithms that require fever can therefore miss critical trends in the incidence of illnesses ( 9 ). The RAVIOLI algorithm, in contrast, does not require fever as a criterion and uses both laboratory test results and an evidence-based set of diagnosis codes to increase both sensitivity and specificity.
Limitations of RAVIOLI surveillance include its development in a single region of the country using data from just 3 practice groups. Generalizability to other practice groups and regions need to be assessed. Changes in testing practices or coding practices over time and between practices might change the future performance of the RAVIOLI algorithm. The algorithm will require periodic revalidation and possibly modification. Furthermore, the breadth of pathogen capture using the RAVIOLI algorithm depends on the range and frequency of respiratory viral testing by clinicians; greater use of multiplex testing platforms will provide more granular and robust results. RAVIOLI surveillance is limited to patients who seek care, which likely biases the data toward pathogens associated with more severe disease. The PPV of algorithm components may vary by season; whether and how this affects surveillance should be considered. We used a 10% PPV threshold to select diagnosis codes for inclusion. This threshold was arbitrary, but we found using higher thresholds dramatically reduced the number of eligible diagnosis codes. We also found that the terms associated with diagnosis codes with a PPV of ≥10% were specific in their descriptions and not indicative of broad health conditions. However, the PPV threshold for including diagnosis codes should be considered in future revalidation of the algorithm.
The healthcare site data included in developing the algorithm and whose data are part of the weekly reports came from both ambulatory and inpatient care facilities. We observed variation in which RAVIOLI categories (e.g., influenza, RSV) of the algorithm were detected at each site (data not shown). The limited number of sites makes it difficult to know if apparent differences between ambulatory and inpatient sites resulted from differences in catchment populations, illness severity associated with different viruses, or testing platforms. As the network expands to include a greater number and variety of sites, we plan to examine this question further.
The Massachusetts Department of Public Health has used data from the underlying EHR-based system for infectious disease reporting and surveillance for more than a decade ( 18 – 21 , 25 – 28 ). This system has been sustained and enhanced over time to meet MDPH needs. As public health agencies consider what they need for the monitoring of current, emerging, and as-yet unidentified pathogens, we have found that a robust EHR data platform is a critical complement to traditional surveillance data.
In conclusion, we developed an integrated, routine, automated EHR-based system for respiratory virus surveillance in Massachusetts. As experience with this approach expands, the hope is that this system will provide early indications of emerging infection trends and prevailing pathogens that render a fuller picture of respiratory viral activity beyond ILI and COVID-like illnesses. A broader view of circulating pathogens will provide public health agencies and healthcare institutions with more precise information useful for informing testing guidance, optimizing health communications; developing more targeted prevention activities, including vaccination; initiating enhanced infection control measures, such as masking and posting of notices in facilities; and generating other policies optimized to minimize the effect on population health of specific circulating pathogens.
Dr. Cocoros is a principal research scientist at the Harvard Pilgrim Health Care Institute and a principal associate in the Department of Population Medicine. Her primary research interests include infectious disease epidemiology, pharmacoepidemiology, and the use of electronic health data for research and surveillance, from pandemic response to pragmatic trials.
Acknowledgments
The authors thank the following colleagues for their contributions: Kelly O’Keefe, Myfanwy Callahan, Dan Flanagan, Jonathan Bress, Brian Herrick, and Michelle Weiss.
This work was supported with funding from the Massachusetts Department of Public Health.
- Hansen CL , Chaves SS , Demont C , Viboud C . Mortality associated with influenza and respiratory syncytial virus in the US, 1999–2018. JAMA Netw Open . 2022 ; 5 : e220527 . DOI PubMed Google Scholar
- Ackerson B , Tseng HF , Sy LS , Solano Z , Slezak J , Luo Y , et al. Severe morbidity and mortality associated with respiratory syncytial virus versus influenza infection in hospitalized older adults. Clin Infect Dis . 2019 ; 69 : 197 – 203 . DOI PubMed Google Scholar
- Troeger CE , Blacker BF , Khalil IA , Zimsen SRM , Albertson SB , Abate D , et al. ; GBD 2017 Influenza Collaborators . Mortality, morbidity, and hospitalisations due to influenza lower respiratory tract infections, 2017: an analysis for the Global Burden of Disease Study 2017. Lancet Respir Med . 2019 ; 7 : 69 – 89 . DOI PubMed Google Scholar
- Centers for Disease Control and Prevention . Overview of the National Syndromic Surveillance Program [ cited 2022 Aug 9 ]. https://www.cdc.gov/nssp/documents/NSSP-overview.pdf
- Boehmer TK , DeVies J , Caruso E , van Santen KL , Tang S , Black CL , et al. Changing age distribution of the COVID-19 pandemic—United States, May–August 2020. MMWR Morb Mortal Wkly Rep . 2020 ; 69 : 1404 – 9 . DOI PubMed Google Scholar
- Elliot AJ , Harcourt SE , Hughes HE , Loveridge P , Morbey RA , Smith S , et al. The COVID-19 pandemic: a new challenge for syndromic surveillance. Epidemiol Infect . 2020 ; 148 : e122 . DOI PubMed Google Scholar
- Güemes A , Ray S , Aboumerhi K , Desjardins MR , Kvit A , Corrigan AE , et al. A syndromic surveillance tool to detect anomalous clusters of COVID-19 symptoms in the United States. Sci Rep . 2021 ; 11 : 4660 . DOI PubMed Google Scholar
- Willis SJ , Eberhardt K , Randall L , DeMaria A , Brown CM , Madoff LC , et al. The evolving nature of syndromic surveillance during the COVID-19 pandemic in Massachusetts. Open Forum Infect Dis . 2021 ; 8 ( Suppl 1 ): S695 . DOI Google Scholar
- Cocoros NM , Willis SJ , Eberhardt K , Morrison M , Randall LM , DeMaria A , et al. Syndromic surveillance for COVID-19, Massachusetts, February 2020–November 2022: the impact of fever and severity on algorithm performance. Public Health Rep . 2023 ; 138 : 756 – 62 . DOI PubMed Google Scholar
- Cohen C , Kleynhans J , Moyes J , McMorrow ML , Treurnicht FK , Hellferscee O , et al. ; PHIRST group . Asymptomatic transmission and high community burden of seasonal influenza in an urban and a rural community in South Africa, 2017-18 (PHIRST): a population cohort study. Lancet Glob Health . 2021 ; 9 : e863 – 74 . DOI PubMed Google Scholar
- Elson W , Zambon M , de Lusignan S . Integrated respiratory surveillance after the COVID-19 pandemic. Lancet . 2022 ; 400 : 1924 – 5 . DOI PubMed Google Scholar
- Ziegler T , Moen A , Zhang W , Cox NJ . Global Influenza Surveillance and Response System: 70 years of responding to the expected and preparing for the unexpected. Lancet . 2022 ; 400 : 981 – 2 . DOI PubMed Google Scholar
- Centers for Disease Control and Prevention (CDC) . Automated detection and reporting of notifiable diseases using electronic medical records versus passive surveillance—massachusetts, June 2006-July 2007. MMWR Morb Mortal Wkly Rep . 2008 ; 57 : 373 – 6 . PubMed Google Scholar
- Klompas M , McVetta J , Lazarus R , Eggleston E , Haney G , Kruskal BA , et al. Integrating clinical practice and public health surveillance using electronic medical record systems. Am J Public Health . 2012 ; 102 ( Suppl 3 ): S325 – 32 . DOI PubMed Google Scholar
- Klompas M , Cocoros NM , Menchaca JT , Erani D , Hafer E , Herrick B , et al. State and local chronic disease surveillance using electronic health record systems. Am J Public Health . 2017 ; 107 : 1406 – 12 . DOI PubMed Google Scholar
- Lazarus R , Klompas M , Campion FX , McNabb SJ , Hou X , Daniel J , et al. Electronic Support for Public Health: validated case finding and reporting for notifiable diseases using electronic medical data. J Am Med Inform Assoc . 2009 ; 16 : 18 – 24 . DOI PubMed Google Scholar
- Vogel J , Brown JS , Land T , Platt R , Klompas M . MDPHnet: secure, distributed sharing of electronic health record data for public health surveillance, evaluation, and planning. Am J Public Health . 2014 ; 104 : 2265 – 70 . DOI PubMed Google Scholar
- Willis SJ , Cocoros NM , Callahan M , Herrick B , Brown CM , Kruskal BA , et al. Assessment of antibiotic prescriptions for Lyme disease after modification of reporting language for positive screening test results. JAMA Netw Open . 2022 ; 5 : e2144928 . DOI PubMed Google Scholar
- Willis SJ , Elder H , Cocoros NM , Callahan M , Hsu KK , Klompas M . Impact of an electronic medical record best practice alert on expedited partner therapy for chlamydia infection and reinfection. Open Forum Infect Dis. 2022 ;9:ofab574.
- Willis SJ , Elder H , Cocoros N , Young J , Marcus JL , Eberhardt K , et al. More screening or more disease? Gonorrhea testing and positivity patterns among men in 3 large clinical practices in Massachusetts, 2010–2017. Clin Infect Dis . 2020 ; 71 : e399 – 405 . DOI PubMed Google Scholar
- Dee EC , Hsu KK , Kruskal BA , Menchaca JT , Zambarano B , Cocoros N , et al. Temporal patterns in chlamydia repeat testing in Massachusetts. Am J Prev Med . 2019 ; 56 : 458 – 63 . DOI PubMed Google Scholar
- Sutton D , Fuchs K , D’Alton M , Goffman D . Universal screening for SARS-CoV-2 in women admitted for delivery. N Engl J Med . 2020 ; 382 : 2163 – 4 . DOI PubMed Google Scholar
- Ma Q , Liu J , Liu Q , Kang L , Liu R , Jing W , et al. Global percentage of asymptomatic SARS-CoV-2 infections among the tested population and individuals with confirmed COVID-19 diagnosis: a systematic review and meta-analysis. JAMA Netw Open . 2021 ; 4 : e2137257 . DOI PubMed Google Scholar
- Struyf T , Deeks JJ , Dinnes J , Takwoingi Y , Davenport C , Leeflang MM , et al. ; Cochrane COVID-19 Diagnostic Test Accuracy Group . Signs and symptoms to determine if a patient presenting in primary care or hospital outpatient settings has COVID-19 disease. Cochrane Database Syst Rev . 2020 ; 7 : CD013665 . PubMed Google Scholar
- Elder HR , Gruber S , Willis SJ , Cocoros N , Callahan M , Flagg EW , et al. Can machine learning help identify patients at risk for recurrent sexually transmitted infections? Sex Transm Dis . 2021 ; 48 : 56 – 62 . DOI PubMed Google Scholar
- Gruber S , Krakower D , Menchaca JT , Hsu K , Hawrusik R , Maro JC , et al. Using electronic health records to identify candidates for human immunodeficiency virus pre-exposure prophylaxis: An application of super learning to risk prediction when the outcome is rare. Stat Med . 2020 ; 39 : 3059 – 73 . DOI PubMed Google Scholar
- Yih WK , Cocoros NM , Crockett M , Klompas M , Kruskal BA , Kulldorff M , et al. Automated influenza-like illness reporting—an efficient adjunct to traditional sentinel surveillance. Public Health Rep . 2014 ; 129 : 55 – 63 . DOI PubMed Google Scholar
- Klompas M , Haney G , Church D , Lazarus R , Hou X , Platt R . Automated identification of acute hepatitis B using electronic medical record data to facilitate public health surveillance. PLoS One . 2008 ; 3 : e2626 . DOI PubMed Google Scholar
- Figure 1 . Numbers of patients with a clinical encounter for respiratory virus–like illness and the percentages that met the requirements for influenza-like illness versus those of the RAVIOLI algorithm for monitoring...
- Figure 2 . Numbers of patients that met the requirements for the RAVIOLI algorithm for monitoring respiratory virus–like illness, by pathogen category and week, Massachusetts, USA, October 2015–January 2024. A) October 2015–January...
- Table 1 . Respiratory pathogens and test types included in RAVIOLI algorithm for monitoring respiratory virus–like illness
- Table 2 . ICD-10 diagnosis codes that met the positive predictive value threshold for confirmed respiratory viral illnesses and are included in the RAVIOLI algorithm for monitoring respiratory virus–like illness
Suggested citation for this article : Cocoros NM, Eberhardt K, Nguyen V-T, Brown CM, DeMaria A, Madoff LC, et al. Electronic health record–based algorithm for monitoring respiratory virus–like illness. Emerg Infect Dis. 2024 Jun [ date cited ]. https://doi.org/10.3201/eid3006.230473
DOI: 10.3201/eid3006.230473
Original Publication Date: May 13, 2024
Table of Contents – Volume 30, Number 6—June 2024
Please use the form below to submit correspondence to the authors or contact them at the following address:
Noelle M. Cocoros, Harvard Pilgrim Health Care Institute, Department of Population Medicine, 401 Park Dr, Ste 401 East, Boston, MA 02215, USA
Comment submitted successfully, thank you for your feedback.
There was an unexpected error. Message not sent.
Exit Notification / Disclaimer Policy
- The Centers for Disease Control and Prevention (CDC) cannot attest to the accuracy of a non-federal website.
- Linking to a non-federal website does not constitute an endorsement by CDC or any of its employees of the sponsors or the information and products presented on the website.
- You will be subject to the destination website's privacy policy when you follow the link.
- CDC is not responsible for Section 508 compliance (accessibility) on other federal or private website.
Metric Details
Article views: 158.
Data is collected weekly and does not include downloads and attachments. View data is from .
What is the Altmetric Attention Score?
The Altmetric Attention Score for a research output provides an indicator of the amount of attention that it has received. The score is derived from an automated algorithm, and represents a weighted count of the amount of attention Altmetric picked up for a research output.

OpenSAFELY: Effectiveness of COVID-19 vaccination in children and adolescents.
- Find this author on Google Scholar
- Find this author on PubMed
- Search for this author on this site
- ORCID record for Colm D Andrews
- For correspondence: [email protected]
- ORCID record for Edward P K Parker
- ORCID record for Andrea L Schaffer
- ORCID record for Amelia CA Green
- ORCID record for Helen J Curtis
- ORCID record for Alex J Walker
- ORCID record for Lucy Bridges
- ORCID record for Christopher Wood
- ORCID record for Christopher Bates
- ORCID record for Jonathan Cockburn
- ORCID record for Amir Mehrkar
- ORCID record for Brian MacKenna
- ORCID record for Sebastian CJ Bacon
- ORCID record for Ben Goldacre
- ORCID record for Jonathan AC Sterne
- ORCID record for William J Hulme
- Info/History
- Supplementary material
- Preview PDF
Background Children and adolescents in England were offered BNT162b2 as part of the national COVID-19 vaccine roll out from September 2021. We assessed the safety and effectiveness of first and second dose BNT162b2 COVID-19 vaccination in children and adolescents in England. Methods With the approval of NHS England, we conducted an observational study in the OpenSAFELY-TPP database, including a) adolescents aged 12-15 years, and b) children aged 5-11 years and comparing individuals receiving i) first vaccination with unvaccinated controls and ii) second vaccination to single-vaccinated controls. We matched vaccinated individuals with controls on age, sex, region, and other important characteristics. Outcomes were positive SARS-CoV-2 test (adolescents only); COVID-19 A&E attendance; COVID-19 hospitalisation; COVID-19 critical care admission; COVID-19 death, with non-COVID-19 death and fractures as negative control outcomes and A&E attendance, unplanned hospitalisation, pericarditis, and myocarditis as safety outcomes. Results Amongst 820,926 previously unvaccinated adolescents, the incidence rate ratio (IRR) for positive SARS-CoV-2 test comparing vaccination with no vaccination was 0.74 (95% CI 0.72-0.75), although the 20-week risks were similar. The IRRs were 0.60 (0.37-0.97) for COVID-19 A&E attendance, 0.58 (0.38-0.89) for COVID-19 hospitalisation, 0.99 (0.93-1.06) for fractures, 0.89 (0.87-0.91) for A&E attendances and 0.88 (0.81-0.95) for unplanned hospitalisation. Amongst 441,858 adolescents who had received first vaccination IRRs comparing second dose with first dose only were 0.67 (0.65-0.69) for positive SARS-CoV-2 test, 1.00 (0.20-4.96) for COVID-19 A&E attendance, 0.60 (0.26-1.37) for COVID-19 hospitalisation, 0.94 (0.84-1.05) for fractures, 0.93 (0.89-0.98) for A&E attendance and 0.99 (0.86-1.13) for unplanned hospitalisation. Amongst 283,422 previously unvaccinated children and 132,462 children who had received a first vaccine dose, COVID-19-related outcomes were too rare to allow IRRs to be estimated precisely. A&E attendance and unplanned hospitalisation were slightly higher after first vaccination (IRRs versus no vaccination 1.05 (1.01-1.10) and 1.10 (0.95-1.26) respectively) but slightly lower after second vaccination (IRRs versus first dose 0.95 (0.86-1.05) and 0.78 (0.56-1.08) respectively). There were no COVID-19-related deaths in any group. Fewer than seven (exact number redacted) COVID-19-related critical care admissions occurred in the adolescent first dose vs unvaccinated cohort. Among both adolescents and children, myocarditis and pericarditis were documented only in the vaccinated groups, with rates of 27 and 10 cases/million after first and second doses respectively. Conclusion BNT162b2 vaccination in adolescents reduced COVID-19 A&E attendance and hospitalisation, although these outcomes were rare. Protection against positive SARS-CoV-2 tests was transient.
Competing Interest Statement
BG has received research funding from the Laura and John Arnold Foundation, the NHS National Institute for Health Research (NIHR), the NIHR School of Primary Care Research, NHS England, the NIHR Oxford Biomedical Research Centre, the Mohn-Westlake Foundation, NIHR Applied Research Collaboration Oxford and Thames Valley, the Wellcome Trust, the Good Thinking Foundation, Health Data Research UK, the Health Foundation, the World Health Organisation, UKRI MRC, Asthma UK, the British Lung Foundation, and the Longitudinal Health and Wellbeing strand of the National Core Studies programme; he is a Non-Executive Director at NHS Digital; he also receives personal income from speaking and writing for lay audiences on the misuse of science. BMK is also employed by NHS England working on medicines policy and clinical lead for primary care medicines data. IJD has received unrestricted research grants and holds shares in GlaxoSmithKline (GSK).
Funding Statement
The OpenSAFELY Platform is supported by grants from the Wellcome Trust (222097/Z/20/Z); MRC (MR/V015757/1, MC_PC-20059, MR/W016729/1); NIHR (NIHR135559, COV-LT2-0073), and Health Data Research UK (HDRUK2021.000, 2021.0157). In addition, this research used data assets made available as part of the Data and Connectivity National Core Study, led by Health Data Research UK in partnership with the Office for National Statistics and funded by UK Research and Innovation (grant ref MC_PC_20058). BG has also received funding from: the Bennett Foundation, the Wellcome Trust, NIHR Oxford Biomedical Research Centre, NIHR Applied Research Collaboration Oxford and Thames Valley, the Mohn-Westlake Foundation; all Bennett Institute staff are supported by BG's grants on this work. The views expressed are those of the authors and not necessarily those of the NIHR, NHS England, UK Health Security Agency (UKHSA) or the Department of Health and Social Care. Funders had no role in the study design, collection, analysis, and interpretation of data; in the writing of the report; and in the decision to submit the article for publication.
Author Declarations
I confirm all relevant ethical guidelines have been followed, and any necessary IRB and/or ethics committee approvals have been obtained.
The details of the IRB/oversight body that provided approval or exemption for the research described are given below:
This study was approved by the Health Research Authority (REC reference 20/LO/0651) and by the London School of Hygeine and Tropical Medicine Ethics Board (reference 21863).
I confirm that all necessary patient/participant consent has been obtained and the appropriate institutional forms have been archived, and that any patient/participant/sample identifiers included were not known to anyone (e.g., hospital staff, patients or participants themselves) outside the research group so cannot be used to identify individuals.
I understand that all clinical trials and any other prospective interventional studies must be registered with an ICMJE-approved registry, such as ClinicalTrials.gov. I confirm that any such study reported in the manuscript has been registered and the trial registration ID is provided (note: if posting a prospective study registered retrospectively, please provide a statement in the trial ID field explaining why the study was not registered in advance).
I have followed all appropriate research reporting guidelines, such as any relevant EQUATOR Network research reporting checklist(s) and other pertinent material, if applicable.
Data Availability
All data were linked, stored and analysed securely using the OpenSAFELY platform, https://www.opensafely.org/, as part of the NHS England OpenSAFELY COVID-19 service. Data include pseudonymised data such as coded diagnoses, medications and physiological parameters. No free text data was included. All code is shared openly for review and re-use under MIT open license [https://github.com/opensafely/vaccine-effectiveness-in-kids]. Detailed pseudonymised patient data is potentially re-identifiable and therefore not shared. Primary care records managed by the GP software provider, TPP were linked to ONS death data and the Index of Multiple Deprivation through OpenSAFELY.
View the discussion thread.
Supplementary Material
Thank you for your interest in spreading the word about medRxiv.
NOTE: Your email address is requested solely to identify you as the sender of this article.

Citation Manager Formats
- EndNote (tagged)
- EndNote 8 (xml)
- RefWorks Tagged
- Ref Manager
- Tweet Widget
- Facebook Like
- Google Plus One
- Addiction Medicine (324)
- Allergy and Immunology (628)
- Anesthesia (165)
- Cardiovascular Medicine (2384)
- Dentistry and Oral Medicine (289)
- Dermatology (207)
- Emergency Medicine (380)
- Endocrinology (including Diabetes Mellitus and Metabolic Disease) (839)
- Epidemiology (11778)
- Forensic Medicine (10)
- Gastroenterology (703)
- Genetic and Genomic Medicine (3752)
- Geriatric Medicine (350)
- Health Economics (635)
- Health Informatics (2401)
- Health Policy (935)
- Health Systems and Quality Improvement (900)
- Hematology (341)
- HIV/AIDS (782)
- Infectious Diseases (except HIV/AIDS) (13324)
- Intensive Care and Critical Care Medicine (769)
- Medical Education (366)
- Medical Ethics (105)
- Nephrology (398)
- Neurology (3513)
- Nursing (198)
- Nutrition (528)
- Obstetrics and Gynecology (675)
- Occupational and Environmental Health (665)
- Oncology (1825)
- Ophthalmology (538)
- Orthopedics (219)
- Otolaryngology (287)
- Pain Medicine (233)
- Palliative Medicine (66)
- Pathology (446)
- Pediatrics (1035)
- Pharmacology and Therapeutics (426)
- Primary Care Research (422)
- Psychiatry and Clinical Psychology (3181)
- Public and Global Health (6152)
- Radiology and Imaging (1281)
- Rehabilitation Medicine and Physical Therapy (749)
- Respiratory Medicine (829)
- Rheumatology (379)
- Sexual and Reproductive Health (372)
- Sports Medicine (323)
- Surgery (402)
- Toxicology (50)
- Transplantation (172)
- Urology (146)
- Download PDF
- Share X Facebook Email LinkedIn
- Permissions
Mortality in Patients Hospitalized for COVID-19 vs Influenza in Fall-Winter 2023-2024
- 1 Clinical Epidemiology Center, VA St Louis Health Care System, St Louis, Missouri
In the first year of the COVID-19 pandemic, risk of death in people hospitalized for COVID-19 was substantially higher than in people hospitalized for seasonal influenza. 1 , 2 The risk of death due to COVID-19 has since declined. In fall-winter 2022-2023, people hospitalized for COVID-19 had a 60% higher risk of death compared with those hospitalized for seasonal influenza. 3 New variants of SARS-CoV-2 have continued to appear, including the emergence of JN.1, the predominant variant in the US since December 24, 2023. 4 This study evaluated the risk of death in a cohort of people hospitalized for COVID-19 or seasonal influenza in fall-winter 2023-2024.
Read More About
Xie Y , Choi T , Al-Aly Z. Mortality in Patients Hospitalized for COVID-19 vs Influenza in Fall-Winter 2023-2024. JAMA. Published online May 15, 2024. doi:10.1001/jama.2024.7395
Manage citations:
© 2024
Artificial Intelligence Resource Center
Cardiology in JAMA : Read the Latest
Browse and subscribe to JAMA Network podcasts!
Others Also Liked
Select your interests.
Customize your JAMA Network experience by selecting one or more topics from the list below.
- Academic Medicine
- Acid Base, Electrolytes, Fluids
- Allergy and Clinical Immunology
- American Indian or Alaska Natives
- Anesthesiology
- Anticoagulation
- Art and Images in Psychiatry
- Artificial Intelligence
- Assisted Reproduction
- Bleeding and Transfusion
- Caring for the Critically Ill Patient
- Challenges in Clinical Electrocardiography
- Climate and Health
- Climate Change
- Clinical Challenge
- Clinical Decision Support
- Clinical Implications of Basic Neuroscience
- Clinical Pharmacy and Pharmacology
- Complementary and Alternative Medicine
- Consensus Statements
- Coronavirus (COVID-19)
- Critical Care Medicine
- Cultural Competency
- Dental Medicine
- Dermatology
- Diabetes and Endocrinology
- Diagnostic Test Interpretation
- Drug Development
- Electronic Health Records
- Emergency Medicine
- End of Life, Hospice, Palliative Care
- Environmental Health
- Equity, Diversity, and Inclusion
- Facial Plastic Surgery
- Gastroenterology and Hepatology
- Genetics and Genomics
- Genomics and Precision Health
- Global Health
- Guide to Statistics and Methods
- Hair Disorders
- Health Care Delivery Models
- Health Care Economics, Insurance, Payment
- Health Care Quality
- Health Care Reform
- Health Care Safety
- Health Care Workforce
- Health Disparities
- Health Inequities
- Health Policy
- Health Systems Science
- History of Medicine
- Hypertension
- Images in Neurology
- Implementation Science
- Infectious Diseases
- Innovations in Health Care Delivery
- JAMA Infographic
- Law and Medicine
- Leading Change
- Less is More
- LGBTQIA Medicine
- Lifestyle Behaviors
- Medical Coding
- Medical Devices and Equipment
- Medical Education
- Medical Education and Training
- Medical Journals and Publishing
- Mobile Health and Telemedicine
- Narrative Medicine
- Neuroscience and Psychiatry
- Notable Notes
- Nutrition, Obesity, Exercise
- Obstetrics and Gynecology
- Occupational Health
- Ophthalmology
- Orthopedics
- Otolaryngology
- Pain Medicine
- Palliative Care
- Pathology and Laboratory Medicine
- Patient Care
- Patient Information
- Performance Improvement
- Performance Measures
- Perioperative Care and Consultation
- Pharmacoeconomics
- Pharmacoepidemiology
- Pharmacogenetics
- Pharmacy and Clinical Pharmacology
- Physical Medicine and Rehabilitation
- Physical Therapy
- Physician Leadership
- Population Health
- Primary Care
- Professional Well-being
- Professionalism
- Psychiatry and Behavioral Health
- Public Health
- Pulmonary Medicine
- Regulatory Agencies
- Reproductive Health
- Research, Methods, Statistics
- Resuscitation
- Rheumatology
- Risk Management
- Scientific Discovery and the Future of Medicine
- Shared Decision Making and Communication
- Sleep Medicine
- Sports Medicine
- Stem Cell Transplantation
- Substance Use and Addiction Medicine
- Surgical Innovation
- Surgical Pearls
- Teachable Moment
- Technology and Finance
- The Art of JAMA
- The Arts and Medicine
- The Rational Clinical Examination
- Tobacco and e-Cigarettes
- Translational Medicine
- Trauma and Injury
- Treatment Adherence
- Ultrasonography
- Users' Guide to the Medical Literature
- Vaccination
- Venous Thromboembolism
- Veterans Health
- Women's Health
- Workflow and Process
- Wound Care, Infection, Healing
- Register for email alerts with links to free full-text articles
- Access PDFs of free articles
- Manage your interests
- Save searches and receive search alerts

IMAGES
VIDEO
COMMENTS
Abstract. A novel coronavirus (CoV) named '2019-nCoV' or '2019 novel coronavirus' or 'COVID-19' by the World Health Organization (WHO) is in charge of the current outbreak of pneumonia that began at the beginning of December 2019 near in Wuhan City, Hubei Province, China [1-4]. ... COVID-19 has reached to more than 150 nations ...
Abstract. Since its emergence in December 2019, corona virus disease 2019 (COVID-19) has impacted several countries, affecting more than 90 thousand patients and making it a global public threat. The routes of transmission are direct contact, and droplet and possible aerosol transmissions. Due to the unique nature of dentistry, most dental ...
Introduction. COVID-19 is a growing pandemic with initial cases identified in Wuhan, Hubei province, China toward the end of December 2019. Labeled as Novel Coronavirus 2019 (2019-nCoV) initially by the Chinese Center for Disease Control and Prevention (CDC) which was subsequently renamed as Severe Acute Respiratory Syndrome-Coronavirus-2 (SARS-CoV-2) due to its homology with SARS-CoV by the ...
Abstract : COVID-19's impacts on workers and workplaces across the globe have been dramatic. We present a broad review of prior research rooted in work and organizational psychology, and related fields, for making sense of the implications for employees, teams, and work ...
We performed a systematic review to evaluate the methodological quality of currently available COVID-19 studies compared to historical controls. A total of 9895 titles and abstracts were screened ...
Download MP3. In the final Coronapod of 2020, we dive into the scientific literature to reflect on the COVID-19 pandemic. Researchers have discovered so much about SARS-CoV-2 - information that ...
Abstract Send to Citation Mgr. Add to Favorites. Email to a Friend. Track Citations. Selected Bibliography of Recent Research in COVID-19 ... Coronavirus (COVID-19) update: FDA authorizes additional oral antiviral for treatment of COVID-19 in certain adults. 2021 [accessed 2022 Jan 20]. Available from: ...
2. Read the abstract and look at the pictures. Research papers are long and dense with a very different structure compared with articles in the normal media. Media articles start with the most ...
Abstract. The appearance of a novel coronavirus in late 2019 radically changed the community of researchers working on coronaviruses since the 2002 SARS epidemic. In 2020, coronavirus-related publications grew by 20 times over the previous two years, with 130,000 more researchers publishing on related topics.
Students can choose to write a full-length college essay on the coronavirus or summarize their experience in a shorter form. To help students explain how the pandemic affected them, The Common App ...
Possible aerosol transmission of COVID-19 and special precautions in dentistry; Use of mouthwashes against COVID-19 in dentistry; What dentists need to know about COVID-19; COVID-19 and dentistry: prevention in dental practice, a literature review; COVID-19 pandemic-a focused review for clinicians
This project will examine the experiences faced by faculty and students in higher education during the COVID-19 pandemic. To understand the complexity of the situation we combine theory and best practices from the fields of I/O Psychology, Crisis Communication, Disaster Management, and Higher Education. Essentially, better understanding faculty ...
Links with this icon indicate that you are leaving the CDC website.. The Centers for Disease Control and Prevention (CDC) cannot attest to the accuracy of a non-federal website. Linking to a non-federal Website does not constitute an endorsement by CDC or any of its employees of the sponsors or the information and products presented on the website.
Abstract The spread of infectious diseases was further promoted due to busy cities, increased travel, and climate change, which led to outbreaks, epidemics, and even pandemics. The world experienced the severity of the 125 nm virus called the coronavirus disease 2019 (COVID-19), a pandemic declared by the World Health Organization (WHO) in 2019. Many investigations revealed a strong ...
Key Points. Question Was telehealth use associated with quality, access, and cost of care outcomes for fee-for-service Medicare beneficiaries during the COVID-19 telehealth expansion?. Findings This cohort study compared 3436 hospital service areas (including approximately 30 million Medicare beneficiaries) with different levels of telehealth use in a difference-in-differences analysis framework.
Background: COVID-19 was a novel infection whose outcomes were not well established to be associated with any factors during the start of the pandemic that it caused globally. Objective: To establish epidemiological characteristics associated with mortality among patients hospitalized with covid-19 at a tertiary hospital in Kiambu County Kenya Methods: This was a retrospective Analytical cross ...
Without the availability of a vaccine (at the time of writing) to control the spread of the COVID-19 virus, ... Abstract. Without the availability of a vaccine (at the time of writing) to control the spread of the COVID-19 virus, countries must rely largely on lockdown measures to limit population movement and community spread. ...
Abstract. Viral respiratory illness surveillance has traditionally focused on single pathogens (e.g., influenza) and required fever to identify influenza-like illness (ILI). ... The proportion with a positive test varied by virus and time; patients in the COVID-19 category were least likely and those in the RSV category most likely to have a ...
AN ANALYSIS OF THE COVID-19 PANDEMIC iii ABSTRACT An Analysis of the COVID-19 Pandemic on Students at The University of South Dakota Alexandra Buss Director: Shane Nordyke Ph.D. The COVID-19 pandemic rapidly took over the United States (US) in the beginning of 2020. Nationally, damages to finances, housing, and mental health have impacted many.
Background Children and adolescents in England were offered BNT162b2 as part of the national COVID-19 vaccine roll out from September 2021. We assessed the safety and effectiveness of first and second dose BNT162b2 COVID-19 vaccination in children and adolescents in England. Methods With the approval of NHS England, we conducted an observational study in the OpenSAFELY-TPP database, including ...
In the first year of the COVID-19 pandemic, risk of death in people hospitalized for COVID-19 was substantially higher than in people hospitalized for seasonal influenza. 1,2 The risk of death due to COVID-19 has since declined. In fall-winter 2022-2023, people hospitalized for COVID-19 had a 60% higher risk of death compared with those hospitalized for seasonal influenza. 3 New variants of ...
This study examined how perceived people-oriented culture (POC) was associated with burnout, depressive symptoms, and sleep problems during the COVID-19 pandemic among emergency medical service (EMS) providers in Korea. Methods . We conducted a cross-sectional survey among 693 EMS providers in 2021. Results
Analysing lecturers' perceptions on traditional vs. distance learning: A conceptual study of emergency transferring to distance learning during COVID-19 pandemic Abstract In recent years, and also due to the COVID-19 pandemic, education institutions worldwide have changed their education paradigm from a traditional to an online system.