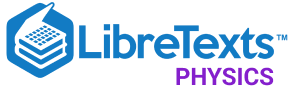
- school Campus Bookshelves
- menu_book Bookshelves
- perm_media Learning Objects
- login Login
- how_to_reg Request Instructor Account
- hub Instructor Commons

Margin Size
- Download Page (PDF)
- Download Full Book (PDF)
- Periodic Table
- Physics Constants
- Scientific Calculator
- Reference & Cite
- Tools expand_more
- Readability
selected template will load here
This action is not available.
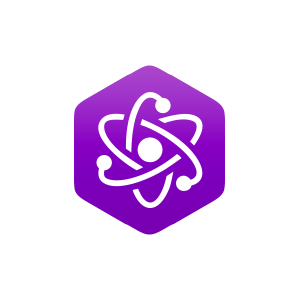
12.5: Kinetic Theory
- Last updated
- Save as PDF
- Page ID 17013
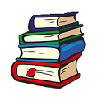
\( \newcommand{\vecs}[1]{\overset { \scriptstyle \rightharpoonup} {\mathbf{#1}} } \)
\( \newcommand{\vecd}[1]{\overset{-\!-\!\rightharpoonup}{\vphantom{a}\smash {#1}}} \)
\( \newcommand{\id}{\mathrm{id}}\) \( \newcommand{\Span}{\mathrm{span}}\)
( \newcommand{\kernel}{\mathrm{null}\,}\) \( \newcommand{\range}{\mathrm{range}\,}\)
\( \newcommand{\RealPart}{\mathrm{Re}}\) \( \newcommand{\ImaginaryPart}{\mathrm{Im}}\)
\( \newcommand{\Argument}{\mathrm{Arg}}\) \( \newcommand{\norm}[1]{\| #1 \|}\)
\( \newcommand{\inner}[2]{\langle #1, #2 \rangle}\)
\( \newcommand{\Span}{\mathrm{span}}\)
\( \newcommand{\id}{\mathrm{id}}\)
\( \newcommand{\kernel}{\mathrm{null}\,}\)
\( \newcommand{\range}{\mathrm{range}\,}\)
\( \newcommand{\RealPart}{\mathrm{Re}}\)
\( \newcommand{\ImaginaryPart}{\mathrm{Im}}\)
\( \newcommand{\Argument}{\mathrm{Arg}}\)
\( \newcommand{\norm}[1]{\| #1 \|}\)
\( \newcommand{\Span}{\mathrm{span}}\) \( \newcommand{\AA}{\unicode[.8,0]{x212B}}\)
\( \newcommand{\vectorA}[1]{\vec{#1}} % arrow\)
\( \newcommand{\vectorAt}[1]{\vec{\text{#1}}} % arrow\)
\( \newcommand{\vectorB}[1]{\overset { \scriptstyle \rightharpoonup} {\mathbf{#1}} } \)
\( \newcommand{\vectorC}[1]{\textbf{#1}} \)
\( \newcommand{\vectorD}[1]{\overrightarrow{#1}} \)
\( \newcommand{\vectorDt}[1]{\overrightarrow{\text{#1}}} \)
\( \newcommand{\vectE}[1]{\overset{-\!-\!\rightharpoonup}{\vphantom{a}\smash{\mathbf {#1}}}} \)
learning objectives
- Express the relationship between the pressure and the average kinetic energy of gas molecules in the form of equation
In Newtonian mechanics, if pressure is the force divided by the area on which the force is exerted, then what is the origin of pressure in a gas? What forces create the pressure? We can gain a better understanding of pressure (and temperature as well) from the kinetic theory of gases, which assumes that atoms and molecules are in continuous random motion.
Microscopic Origin of Pressure
Pressure is explained by kinetic theory as arising from the force exerted by molecules or atoms impacting on the walls of a container, as illustrated in the figure below. Consider a gas of N molecules, each of mass m, enclosed in a cubical container of volume V=L 3 . When a gas molecule collides with the wall of the container perpendicular to the x coordinate axis and bounces off in the opposite direction with the same speed (an elastic collision), then the momentum lost by the particle and gained by the wall (\(\mathrm{Δp}\)) is:
Translational Motion of Helium : Real gases do not always behave according to the ideal model under certain conditions, such as high pressure. Here, the size of helium atoms relative to their spacing is shown to scale under 1950 atmospheres of pressure.
\[\begin{align} \mathrm{Δp} & \mathrm{=p_{i,x}−p_{f,x}=p_{i,x}−(−p_{i,x})} \\ & \mathrm{=2p_{i,x}=2mv_x} \end{align}\]
where v x is the x-component of the initial velocity of the particle.
The particle impacts one specific side wall once every \(\mathrm{Δt=\frac{2L}{v_x}}\),
(where L is the distance between opposite walls). The force due to this particle is:
\[\mathrm{F=\dfrac{Δp}{Δt}=\dfrac{mv_x^2}{L}.}\]
The total force on the wall, therefore, is:
\[\mathrm{F=\dfrac{Nm\bar{v^2_x}}L}\]
where the bar denotes an average over the N particles. Since the assumption is that the particles move in random directions, if we divide the velocity vectors of all particles in three mutually perpendicular directions, the average value of the squared velocity along each direction must be same. (This does not mean that each particle always travel in 45 degrees to the coordinate axes. )
Pressure : Pressure arises from the force exerted by molecules or atoms impacting on the walls of a container.
This gives \(\mathrm{\bar{v_x^2}=\frac{\bar{v^2}}{3}}\). We can rewrite the force as \(\mathrm{F=\frac{Nm\bar{v^2}}{3L}}\).
This force is exerted on an area L 2 . Therefore the pressure of the gas is:
\[\mathrm{P=\dfrac{F}{L^2}=\dfrac{Nm\bar{v^2}}{3V}=\dfrac{nm \bar{v^2}}{3},}\]
where V=L 3 is the volume of the box. The fraction n=N/V is the number density of the gas. This is a first non-trivial result of the kinetic theory because it relates pressure (a macroscopic property) to the average (translational) kinetic energy per molecule which is a microscopic property.
Speed Distribution of Molecules
A gas of many molecules has a predictable distribution of molecular speeds, known as the Maxwell-Boltzmann distribution.
- Describe the shape and temperature dependence of the Maxwell-Boltzmann distribution curve
The motion of molecules in a gas is random in magnitude and direction for individual molecules, but a gas of many molecules has a predictable distribution of molecular speeds, known as the Maxwell-Boltzmann distribution (illustrated in ). The distribution has a long tail because some molecules may go several times the rms speed. The most probable speed v p (at the peak of the curve) is less than the rms speed v rms . As shown in, the curve is shifted to higher speeds at higher temperatures, with a broader range of speeds.
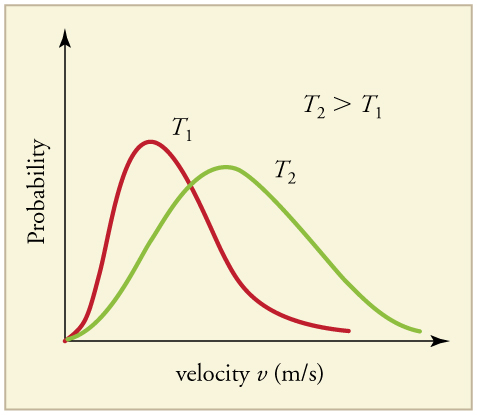
Maxwell-Boltzmann Distribution at Higher Temperatures : The Maxwell-Boltzmann distribution is shifted to higher speeds and is broadened at higher temperatures.
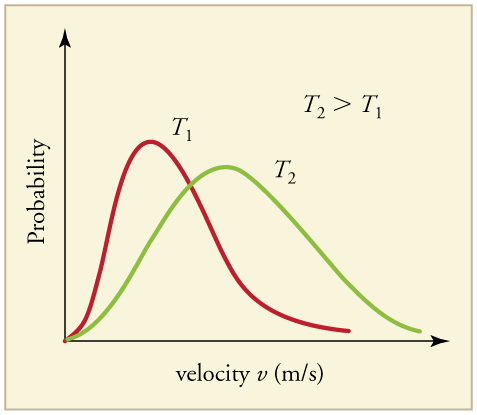
Maxwell-Boltzmann Distribution : The Maxwell-Boltzmann distribution of molecular speeds in an ideal gas. The most likely speed v_p is less than the rms speed v_rms. Although very high speeds are possible, only a tiny fraction of the molecules have speeds that are an order of magnitude greater than v_rms.
Maxwell-Boltzmann Distribution
Maxwell-Boltzmann distribution is a probability distribution. It applies to ideal gases close to thermodynamic equilibrium, and is given as the following equation:
\[\mathrm{f_v(v_x,v_y,v_z)=(\dfrac{m}{2πkT})^{3/2} exp[−\dfrac{m(v_x^2+v_y^2+v_z^2)}{2kT}],}\]
where fv is the velocity probability density function. (Derivation of the formula goes beyond the scope of introductory physics. ) The formula calculates the probability of finding a particle with velocity in the infinitesimal element [ dv x , dv y , dv z ] about velocity v = [ v x , v y , v z ] is:
\[\mathrm{f_v(v_x,v_y,v_z)dv_xdv_ydv_z.}\]
It can also be shown that the Maxwell–Boltzmann velocity distribution for the vector velocity [ v x , v y , v z ] is the product of the distributions for each of the three directions:
\[\mathrm{f_v(v_x,v_y,v_z)=f_v(v_x)f_v(v_y)f_v(v_z)}\]
where the distribution for a single direction is,
\[\mathrm{f_v(v_i)=\sqrt{\dfrac{m}{2πkT}} \; exp[ \dfrac{−mv_i^2}{2kT}].}\]
This makes sense because particles are moving randomly, meaning that each component of the velocity should be independent.
Distribution for the Speed
Usually, we are more interested in the speeds of molecules rather than their component velocities. The Maxwell–Boltzmann distribution for the speed follows immediately from the distribution of the velocity vector, above. Note that the speed is:
\[\mathrm{v=\sqrt{v_x^2+v_y^2+v_z^2}}\]
and the increment of volume is:
\[\mathrm{dv_x \; dv_y \; dv_z=v^2 \sin ϕ \; dv \; dθ \; dϕ,}\]
where \(\mathrm{θ}\) and \(\mathrm{ϕ}\) are the “course” (azimuth of the velocity vector) and “path angle” (elevation angle of the velocity vector). Integration of the normal probability density function of the velocity, above, over the course (from 0 to \(\mathrm{2π}\)) and path angle (from 0 to \(\mathrm{π}\)), with substitution of the speed for the sum of the squares of the vector components, yields the following probability density function (known simply as the Maxwell distribution):
\(\mathrm{f(v)=\sqrt{(\frac{m}{2πkT})^3}4πv^2 \; exp(\frac{−mv^2}{2kT})}\) for speed v.
Temperature
Temperature is directly proportional to the average translational kinetic energy of molecules in an ideal gas.
- Describe relationship between temperature and energy of molecules in an ideal gas
Intuitively, hotter air suggests faster movement of air molecules. In this atom, we will derive an equation relating the temperature of a gas (a macroscopic quantity) to the average kinetic energy of individual molecules (a microscopic quantity). This is a basic and extremely important relationship in the kinetic theory of gases.
Microscopic View
We assume that a molecule is small compared with the separation of molecules in the gas (confined in a three dimensional container), and that its interaction with other molecules can be ignored. Also, we assume elastic collisions when molecules hit the wall of the container, as illustrated in.
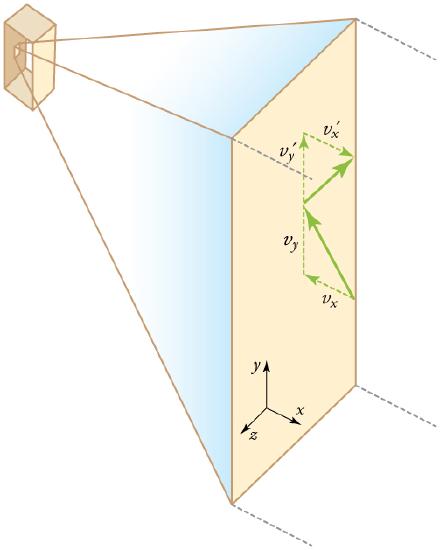
Elastic Collisions When Molecules Hit the Wall of the Container : Gas in a box exerts an outward pressure on its walls. A molecule colliding with a rigid wall has the direction of its velocity and momentum in the x-direction reversed. This direction is perpendicular to the wall. The components of its velocity momentum in the y- and z-directions are not changed, which means there is no force parallel to the wall.
We have seen in the Atom on “Origin of Pressure ” that, for an ideal gas under our assumptions:
\[\mathrm{P=\dfrac{Nm \bar{v^2}}{3V},}\]
where P is the pressure, N is the number of molecules, m is the mass of the molecule, v is the speed of molecules, and V is the volume of the gas. From the equation, we get:
\[\mathrm{PV=\dfrac{1}{3}Nm \bar{v^2} \; (Eq. 1).}\]
What can we learn from this atomic and molecular version of the ideal gas law ? We can derive a relationship between temperature and the average translational kinetic energy of molecules in a gas. Recall the macroscopic expression of the ideal gas law:
\[\mathrm{PV=NkT \; (Eq. 2),}\]
where N is the number of molecules, T is the temperature of the gas, and k is the Boltzmann constant.
Equating the right hand sides of the macroscopic and microscopic versions of the ideal gas law (Eq. 1 & 2) gives:
\[\mathrm{\dfrac{1}{3}m\bar{v^2}=kT.}\]
Thermal Energy
Note that the average kinetic energy (KE) of a molecule in the gas is:
\[\mathrm{\dfrac{1}{2}m\bar{v^2}.}\]
Therefore, we derive the relation between average KE and temperature as follows:
\[\mathrm{\bar{KE}=\dfrac{1}{2}m\bar{v^2}=\dfrac{3}{2}kT, \; (Eq. 3).}\]
The average translational kinetic energy of a molecule is called thermal energy.
Eq. 3 is a molecular interpretation of temperature. It has been found to be valid for gases and reasonably accurate in liquids and solids. It is another definition of temperature based on an expression of the molecular energy. It is sometimes useful to know the average speed of molecules in a gas in terms of temperature:
\[\mathrm{\bar{v^2}=v_{rms}=\sqrt{\dfrac{3kT}{m}},}\]
where v rms stands for root-mean-square (rms) speed.
Internal Energy of an Ideal Gas
Internal energy is the total energy contained by a thermodynamic system, and has two major components: kinetic energy and potential energy.
- Determine the number of degrees of freedom and calculate the internal energy for an ideal gas molecule
In thermodynamics, internal energy is the total energy contained by a thermodynamic system. Internal energy has two major components: kinetic energy and potential energy. The kinetic energy is due to the motion of the system’s particles (e.g., translations, rotations, vibrations). In ideal gases, there is no inter-particle interaction. Therefore, we will disregard potential energy and only focus on the kinetic energy contribution to the internal energy.
Monatomic Gases
A monatomic gas is one in which atoms are not bound to each other. Noble gases (He, Ne, etc.) are typical examples. A helium balloon is shown in the following figure. In this case, the kinetic energy consists only of the translational energy of the individual atoms. Monoatomic particles do not vibrate, and their rotational energy can be neglected because atomic moment of inertia is so small. Also, they are not electronically excited to higher energies except at very high temperatures. Therefore, practical internal energy changes in an ideal gas may be described solely by changes in its translational kinetic energy.
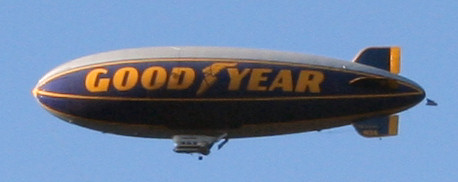
Helium Blimp : Helium, like other noble gases, is a monatomic gas, which often can be described by the ideal gas law. It is the gas of choice to fill airships such as the Goodyear blimp.
The average kinetic energy (KE) of a particle in an ideal gas is given as:
\[\mathrm{\bar{KE}=\dfrac{1}{2}m\bar{v^2}=\dfrac{3}{2}kT,}\]
where k is the Boltzmann’s constant. (See the Atom on “Temperature” in kinetic theory. ) With N atoms in the gas, its total internal energy U is given as:
\[\mathrm{U=\dfrac{3}{2}NkT}\]
where N is the number of atoms in the gas. Note that there are three degrees of freedom in monatomic gases: translation in x, y and z directions.
Since atomic motion is random (and therefore isotropic), each degrees of freedom contribute \(\mathrm{\frac{1}{2}kT}\) per atom to the internal energy.
Diatomic gases
A diatomic molecule (H 2 , O 2 , N 2 , etc.) has 5 degrees of freedom (3 for translation in x, y and z directions, and 2 for rotation). Therefore, the internal energy for diatomic gases is \(\mathrm{U=\frac{5}{2}NkT.}\)
- We can gain a better understanding of pressure (and temperature as well) from the kinetic theory of gases, which assumes that atoms and molecules are in continuous random motion.
- Pressure, a macroscopic property, can be related to the average (translational) kinetic energy per molecule which is a microscopic property by \(\mathrm{P=\frac{nm\bar{v^2}}{3}}\).
- Since the assumption is that the particles move in random directions, the average value of velocity squared along each direction must be same. This gives: \(\mathrm{\bar{v_x^2}=\bar{v_y^2}=\bar{v_z^2}=\bar{v^2}/3}\).
- The Maxwell-Boltzmann distribution has a long tail, and the most probable speed v p is less than the rms speed v rms . The distribution curve is shifted to higher speeds at higher temperatures, with a broader range of speeds.
- Maxwell-Boltzmann distribution is given as follows: \(\mathrm{f_v(v_x,v_y,v_z)=(\frac{m}{2πkT})^{3/2} \; exp[−\frac{m(v_x^2+v_y^2+v_z^2)}{2kT}]}\). It is a product of three independent 1D Maxwell-Boltzmann distributions.
- Molecular speed distribution is given as \(\mathrm{f(v)=\sqrt{(\frac{m}{2πkT})^3}4πv^2 \; exp(\frac{−mv^2}{2kT})}\). This is simply called Maxwell distribution.
- The average translational kinetic energy of a molecule is equivalent to \(\mathrm{\frac{3}{2}kT}\) and is called thermal energy.
- In kinematic theory of gases, macroscopic quantities (such as press and temperature) are explained by considering microscopic (random) motion of molecules.
- The rms speed of molecules in a gas is given as \(\mathrm{\sqrt{\frac{3kT}{m}}}\).
- In ideal gases, there is no inter-particle interaction. Therefore, only the kinetic energy contribute to the internal energy.
- Each degrees of freedom contribute \(\mathrm{\frac{1}{2}kT}\) per atom to the internal energy.
- For monatomic ideal gases with N atoms, its total internal energy U is given as \(\mathrm{U=\frac{3}{2}NkT}\). For diatomic gases, \(\mathrm{U=\frac{5}{2}NkT}\).
- kinetic theory of gases : The kinetic theory of gases describes a gas as a large number of small particles (atoms or molecules), all of which are in constant, random motion.
- Newtonian mechanics : Early classical mechanics as propounded by Isaac Newton, especially that based on his laws of motion and theory of gravity.
- rms : Root mean square: a statistical measure of the magnitude of a varying quantity.
- ideal gas : A hypothetical gas whose molecules exhibit no interaction and undergo elastic collision with each other and with the walls of the container.
- Boltzmann’s constant : The physical constant relating energy at the particle level with temperature observed at the bulk level. It is the gas constant R divided by Avogadro’s number, NA.
- moment of inertia : A measure of a body’s resistance to a change in its angular rotation velocity
- noble gas : Any of the elements of group 18 of the periodic table, being monatomic and (with very limited exceptions) inert.
LICENSES AND ATTRIBUTIONS
CC LICENSED CONTENT, SHARED PREVIOUSLY
- Curation and Revision. Provided by : Boundless.com. License : CC BY-SA: Attribution-ShareAlike
CC LICENSED CONTENT, SPECIFIC ATTRIBUTION
- Kinetic theory. Provided by : Wikipedia. Located at : en.Wikipedia.org/wiki/Kinetic_theory%23Pressure_and_kinetic_energy . License : CC BY-SA: Attribution-ShareAlike
- kinetic theory of gases. Provided by : Wikipedia. Located at : en.Wikipedia.org/wiki/kinetic%20theory%20of%20gases . License : CC BY-SA: Attribution-ShareAlike
- Newtonian mechanics. Provided by : Wiktionary. Located at : en.wiktionary.org/wiki/Newtonian_mechanics . License : CC BY-SA: Attribution-ShareAlike
- Kinetic theory. Provided by : Wikipedia. Located at : en.Wikipedia.org/wiki/Kinetic_theory . License : Public Domain: No Known Copyright
- Maxwellu2013Boltzmann distribution. Provided by : Wikipedia. Located at : en.Wikipedia.org/wiki/Maxwell%E2%80%93Boltzmann_distribution . License : CC BY-SA: Attribution-ShareAlike
- OpenStax College, Kinetic Theory: Atomic and Molecular Explanation of Pressure and Temperature. September 17, 2013. Provided by : OpenStax CNX. Located at : http://cnx.org/content/m42217/latest/ . License : CC BY: Attribution
- rms. Provided by : Wikipedia. Located at : en.Wikipedia.org/wiki/rms . License : CC BY-SA: Attribution-ShareAlike
- OpenStax College, Kinetic Theory: Atomic and Molecular Explanation of Pressure and Temperature. February 4, 2013. Provided by : OpenStax CNX. Located at : http://cnx.org/content/m42217/latest/ . License : CC BY: Attribution
- OpenStax College, College Physics. September 17, 2013. Provided by : OpenStax CNX. Located at : http://cnx.org/content/m42217/latest/?collection=col11406/1.7 . License : CC BY: Attribution
- ideal gas. Provided by : Wiktionary. Located at : en.wiktionary.org/wiki/ideal_gas . License : CC BY-SA: Attribution-ShareAlike
- noble gas. Provided by : Wiktionary. Located at : en.wiktionary.org/wiki/noble_gas . License : CC BY-SA: Attribution-ShareAlike
- Internal energy. Provided by : Wikipedia. Located at : en.Wikipedia.org/wiki/Internal_energy%23Internal_energy_of_the_ideal_gas . License : CC BY-SA: Attribution-ShareAlike
- moment of inertia. Provided by : Wiktionary. Located at : en.wiktionary.org/wiki/moment_of_inertia . License : CC BY-SA: Attribution-ShareAlike
- Boltzmann's constant. Provided by : Wiktionary. Located at : en.wiktionary.org/wiki/Boltzmann's_constant . License : CC BY-SA: Attribution-ShareAlike
- Helium. Provided by : Wikipedia. Located at : http://en.Wikipedia.org/wiki/Helium . License : CC BY: Attribution
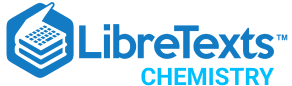
- school Campus Bookshelves
- menu_book Bookshelves
- perm_media Learning Objects
- login Login
- how_to_reg Request Instructor Account
- hub Instructor Commons
Margin Size
- Download Page (PDF)
- Download Full Book (PDF)
- Periodic Table
- Physics Constants
- Scientific Calculator
- Reference & Cite
- Tools expand_more
- Readability
selected template will load here
This action is not available.
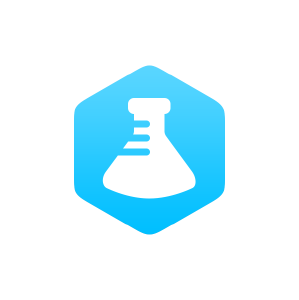
2.6: Kinetic Theory of Gases
- Last updated
- Save as PDF
- Page ID 120466
\( \newcommand{\vecs}[1]{\overset { \scriptstyle \rightharpoonup} {\mathbf{#1}} } \)
\( \newcommand{\vecd}[1]{\overset{-\!-\!\rightharpoonup}{\vphantom{a}\smash {#1}}} \)
\( \newcommand{\id}{\mathrm{id}}\) \( \newcommand{\Span}{\mathrm{span}}\)
( \newcommand{\kernel}{\mathrm{null}\,}\) \( \newcommand{\range}{\mathrm{range}\,}\)
\( \newcommand{\RealPart}{\mathrm{Re}}\) \( \newcommand{\ImaginaryPart}{\mathrm{Im}}\)
\( \newcommand{\Argument}{\mathrm{Arg}}\) \( \newcommand{\norm}[1]{\| #1 \|}\)
\( \newcommand{\inner}[2]{\langle #1, #2 \rangle}\)
\( \newcommand{\Span}{\mathrm{span}}\)
\( \newcommand{\id}{\mathrm{id}}\)
\( \newcommand{\kernel}{\mathrm{null}\,}\)
\( \newcommand{\range}{\mathrm{range}\,}\)
\( \newcommand{\RealPart}{\mathrm{Re}}\)
\( \newcommand{\ImaginaryPart}{\mathrm{Im}}\)
\( \newcommand{\Argument}{\mathrm{Arg}}\)
\( \newcommand{\norm}[1]{\| #1 \|}\)
\( \newcommand{\Span}{\mathrm{span}}\) \( \newcommand{\AA}{\unicode[.8,0]{x212B}}\)
\( \newcommand{\vectorA}[1]{\vec{#1}} % arrow\)
\( \newcommand{\vectorAt}[1]{\vec{\text{#1}}} % arrow\)
\( \newcommand{\vectorB}[1]{\overset { \scriptstyle \rightharpoonup} {\mathbf{#1}} } \)
\( \newcommand{\vectorC}[1]{\textbf{#1}} \)
\( \newcommand{\vectorD}[1]{\overrightarrow{#1}} \)
\( \newcommand{\vectorDt}[1]{\overrightarrow{\text{#1}}} \)
\( \newcommand{\vectE}[1]{\overset{-\!-\!\rightharpoonup}{\vphantom{a}\smash{\mathbf {#1}}}} \)
The kinetic theory describes a gas as a large number of submicroscopic particles (atoms or molecules), all of which are in constant, random motion. The rapidly moving particles constantly collide with each other and with the walls of the container. Kinetic theory explains macroscopic properties of gases, such as pressure, temperature, viscosity, thermal conductivity, and volume, by considering their molecular composition and motion. The theory posits that gas pressure is due to the impacts, on the walls of a container, of molecules or atoms moving at different velocities.
The five basic tenets of the kinetic-molecular theory are as follows:
- A gas is composed of molecules that are separated by average distances that are much greater than the sizes of the molecules themselves. The volume occupied by the molecules of the gas is negligible compared to the volume of the gas itself .
- The molecules of an ideal gas exert no attractive forces on each other, or on the walls of the container.
- The molecules are in constant random motion , and as material bodies, they obey Newton's laws of motion. This means that the molecules move in straight lines (see demo illustration at the left) until they collide with each other or with the walls of the container.
- Collisions are perfectly elastic ; when two molecules collide, they change their directions and kinetic energies, but the total kinetic energy is conserved . Collisions are not “sticky" .
- The average kinetic energy of the gas molecules is directly proportional to the absolute temperature . Notice that the term “average” is very important here; the velocities and kinetic energies of individual molecules will span a wide range of values, and some will even have zero velocity at a given instant. This implies that all molecular motion would cease if the temperature were reduced to absolute zero.
According to this model, most of the volume occupied by a gas is empty space ; this is the main feature that distinguishes gases from condensed states of matter (liquids and solids) in which neighboring molecules are constantly in contact. Gas molecules are in rapid and continuous motion; at ordinary temperatures and pressures their velocities are of the order of 0.1-1 km/sec and each molecule experiences approximately 10 10 collisions with other molecules every second.
If gases do in fact consist of widely-separated particles, then the observable properties of gases must be explainable in terms of the simple mechanics that govern the motions of the individual molecules. The kinetic molecular theory makes it easy to see why a gas should exert a pressure on the walls of a container. Any surface in contact with the gas is constantly bombarded by the molecules.
Figure 2.6.1: When a molecule collides with a rigid wall, the component of its momentum perpendicular to the wall is reversed. A force is thus exerted on the wall, creating pressure. Image used with permisison from OpenSTAX
At each collision, a molecule moving with momentum mv strikes the surface. Since the collisions are elastic, the molecule bounces back with the same velocity in the opposite direction. This change in velocity Δ V is equivalent to a n accelerati o n a ; according to Newton's second law, a force f = ma is thus exerted on the surface of area A exerting a pressure P = f/A .
Kinetic Interpretation of Temperature
According to the kinetic molecular theory, the average kinetic energy of an ideal gas is directly proportional to the absolute temperature. Kinetic energy is the energy a body has by virtue of its motion:
\[ KE = \dfrac{mv^2}{2}\]
As the temperature of a gas rises, the average velocity of the molecules will increase; a doubling of the temperature will increase this velocity by a factor of four. Collisions with the walls of the container will transfer more momentum, and thus more kinetic energy, to the walls. If the walls are cooler than the gas, they will get warmer, returning less kinetic energy to the gas, and causing it to cool until thermal equilibrium is reached. Because temperature depends on the average kinetic energy, the concept of temperature only applies to a statistically meaningful sample of molecules. We will have more to say about molecular velocities and kinetic energies farther on.
Kinetic explanation of Boyle's law : Boyle's law is easily explained by the kinetic molecular theory. The pressure of a gas depends on the number of times per second that the molecules strike the surface of the container. If we compress the gas to a smaller volume, the same number of molecules are now acting against a smaller surface area, so the number striking per unit of area, and thus the pressure, is now greater.
Kinetic explanation of Charles' law : Kinetic molecular theory states that an increase in temperature raises the average kinetic energy of the molecules. If the molecules are moving more rapidly but the pressure remains the same, then the molecules must stay farther apart, so that the increase in the rate at which molecules collide with the surface of the container is compensated for by a corresponding increase in the area of this surface as the gas expands.
Kinetic explanation of Avogadro's law : If we increase the number of gas molecules in a closed container, more of them will collide with the walls per unit time. If the pressure is to remain constant, the volume must increase in proportion, so that the molecules strike the walls less frequently, and over a larger surface area.
Kinetic explanation of Dalton's law : "Every gas is a vacuum to every other gas". This is the way Dalton stated what we now know as his law of partial pressures. It simply means that each gas present in a mixture of gases acts independently of the others. This makes sense because of one of the fundamental tenets of KMT theory that gas molecules have negligible volumes. So Gas A in mixture of A and B acts as if Gas B were not there at all. Each contributes its own pressure to the total pressure within the container, in proportion to the fraction of the molecules it represents.
Derivation of the Ideal Gas Law
One of the triumphs of the kinetic molecular theory was the derivation of the ideal gas law from simple mechanics in the late nineteenth century. This is a beautiful example of how the principles of elementary mechanics can be applied to a simple model to develop a useful description of the behavior of macroscopic matter. We begin by recalling that the pressure of a gas arises from the force exerted when molecules collide with the walls of the container. This force can be found from Newton's law
\[f = ma = m\dfrac{dv}{dt} \label{2.1}\]
in which \(v\) is the velocity component of the molecule in the direction perpendicular to the wall and \(m\) is its mass.
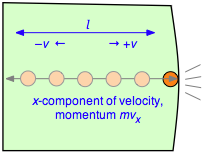
To evaluate the derivative, which is the velocity change per unit time, consider a single molecule of a gas contained in a cubic box of length l . For simplicity, assume that the molecule is moving along the x -axis which is perpendicular to a pair of walls, so that it is continually bouncing back and forth between the same pair of walls. When the molecule of mass m strikes the wall at velocity +v (and thus with a momentum mv ) it will rebound elastically and end up moving in the opposite direction with –v . The total change in velocity per collision is thus 2 v and the change in momentum is \(2mv\).
After the collision the molecule must travel a distance l to the opposite wall, and then back across this same distance before colliding again with the wall in question. This determines the time between successive collisions with a given wall; the number of collisions per second will be \(v/2l\). The force \(F\) exerted on the wall is the rate of change of the momentum, given by the product of the momentum change per collision and the collision frequency:
\[F = \dfrac{d(mv_x)}{dt} = (2mv_x) \times \left( \dfrac{v_x}{2l} \right) = \dfrac{m v_x^2}{l} \label{2-2}\]
Pressure is force per unit area, so the pressure \(P\) exerted by the molecule on the wall of cross-section \(l^2\) becomes
\[ P = \dfrac{mv^2}{l^3} = \dfrac{mv^2}{V} \label{2-3}\]
in which \(V\) is the volume of the box.
As noted near the beginning of this unit, any given molecule will make about the same number of moves in the positive and negative directions, so taking a simple average would yield zero. To avoid this embarrassment, we square the velocities before averaging them
\[\bar{v^2} = \dfrac{v_1^2 + v_2^2 + v_3^2 + v_4^2 .. . v_N^2 }{N}= \dfrac{\sum_i v_i^2}{N} \]
and then take the square root of the average. This result is known as the root mean square (rms) velocity.
\[v_{rms} = \sqrt{ \bar{v^2}}\]
We have calculated the pressure due to a single molecule moving at a constant velocity in a direction perpendicular to a wall. If we now introduce more molecules, we must interpret \(v^2\) as an average value which we will denote by \(\bar{v^2}\). Also, since the molecules are moving randomly in all directions, only one-third of their total velocity will be directed along any one Cartesian axis, so the total pressure exerted by \(N\) molecules becomes
\[ P=\dfrac{N}{3}\dfrac{m \bar{\nu}^2}{V} \label{2.4}\]
Recalling that \(m\bar{v^2}/2\) is the average translational kinetic energy \(\epsilon\), we can rewrite the above expression as
\[PV = \dfrac{1}{3} N m \bar{v^2} = \dfrac{2}{3} N \epsilon \label{2-5}\]
The 2/3 factor in the proportionality reflects the fact that velocity components in each of the three directions contributes ½ kT to the kinetic energy of the particle. The average translational kinetic energy is directly proportional to temperature:
\[\color{red} \epsilon = \dfrac{3}{2} kT \label{2.6}\]
in which the proportionality constant \(k\) is known as the Boltzmann constant . Substituting Equation \(\ref{2.6}\) into Equation \(\ref{2-5}\) yields
\[ PV = \left( \dfrac{2}{3}N \right) \left( \dfrac{3}{2}kT \right) =NkT \label{2.7}\]
The Boltzmann constant k is just the gas constant per molecule. For n moles of particles, the Equation \(\ref{2.7}\) becomes
\[ PV = nRT \label{2.8}\]
which is the Ideal Gas law.
Contributors and Attributions
Stephen Lower, Professor Emeritus ( Simon Fraser U. ) Chem1 Virtual Textbook
If you're seeing this message, it means we're having trouble loading external resources on our website.
If you're behind a web filter, please make sure that the domains *.kastatic.org and *.kasandbox.org are unblocked.
To log in and use all the features of Khan Academy, please enable JavaScript in your browser.
Physics library
Course: physics library > unit 5.
- Introduction to work and energy
- Work and energy (part 2)
- Conservation of energy
- What are energy and work?
What is kinetic energy?
- What is gravitational potential energy?
- What is conservation of energy?
- Work and the work-energy principle
- Work as the transfer of energy
- Work example problems
- Work as area under curve
- Thermal energy from friction
- What is thermal energy?
- Work/energy problem with friction
- Conservative forces
- What is power?
How can we calculate kinetic energy?
What is interesting about kinetic energy.
- Kinetic energy depends on the velocity of the object squared. This means that when the velocity of an object doubles, its kinetic energy quadruples. A car traveling at 60 mph has four times the kinetic energy of an identical car traveling at 30 mph, and hence the potential for four times more death and destruction in the event of a crash.
- Kinetic energy must always be either zero or a positive value. While velocity can have a positive or negative value, velocity squared is always positive.
- Kinetic energy is not a vector. So a tennis ball thrown to the right with a velocity of 5 m/s, has the exact same kinetic energy as a tennis ball thrown down with a velocity of 5 m/s.
Want to join the conversation?
- Upvote Button navigates to signup page
- Downvote Button navigates to signup page
- Flag Button navigates to signup page


- Cambridge Dictionary +Plus
Meaning of kinetic in English
Your browser doesn't support HTML5 audio
- body English
- kinetic energy
- kinetically
- recirculation
- repair to somewhere
kinetic | Intermediate English
Examples of kinetic, translations of kinetic.
Get a quick, free translation!
Word of the Day
hit the road
to leave a place or begin a journey

Searching out and tracking down: talking about finding or discovering things

Learn more with +Plus
- Recent and Recommended {{#preferredDictionaries}} {{name}} {{/preferredDictionaries}}
- Definitions Clear explanations of natural written and spoken English English Learner’s Dictionary Essential British English Essential American English
- Grammar and thesaurus Usage explanations of natural written and spoken English Grammar Thesaurus
- Pronunciation British and American pronunciations with audio English Pronunciation
- English–Chinese (Simplified) Chinese (Simplified)–English
- English–Chinese (Traditional) Chinese (Traditional)–English
- English–Dutch Dutch–English
- English–French French–English
- English–German German–English
- English–Indonesian Indonesian–English
- English–Italian Italian–English
- English–Japanese Japanese–English
- English–Norwegian Norwegian–English
- English–Polish Polish–English
- English–Portuguese Portuguese–English
- English–Spanish Spanish–English
- English–Swedish Swedish–English
- Dictionary +Plus Word Lists
- English Adjective
- Intermediate Adjective
- Translations
- All translations
To add kinetic to a word list please sign up or log in.
Add kinetic to one of your lists below, or create a new one.
{{message}}
Something went wrong.
There was a problem sending your report.
- More from M-W
- To save this word, you'll need to log in. Log In
Definition of kinetics
Examples of kinetics in a sentence.
These examples are programmatically compiled from various online sources to illustrate current usage of the word 'kinetics.' Any opinions expressed in the examples do not represent those of Merriam-Webster or its editors. Send us feedback about these examples.

Word History
circa 1859, in the meaning defined at sense 1a
Dictionary Entries Near kinetics
kinetic potential
kinetic theory
Cite this Entry
“Kinetics.” Merriam-Webster.com Dictionary , Merriam-Webster, https://www.merriam-webster.com/dictionary/kinetics. Accessed 20 May. 2024.
Medical Definition
Medical definition of kinetics, more from merriam-webster on kinetics.
Nglish: Translation of kinetics for Spanish Speakers
Britannica.com: Encyclopedia article about kinetics
Subscribe to America's largest dictionary and get thousands more definitions and advanced search—ad free!
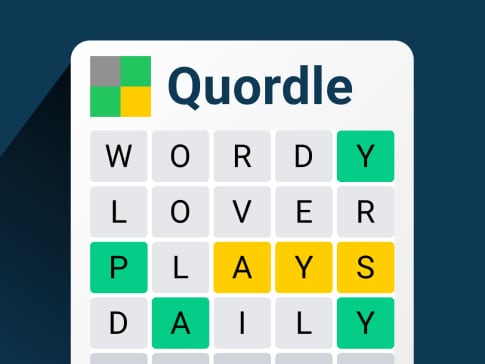
Can you solve 4 words at once?
Word of the day.
See Definitions and Examples »
Get Word of the Day daily email!
Popular in Grammar & Usage
More commonly misspelled words, your vs. you're: how to use them correctly, every letter is silent, sometimes: a-z list of examples, more commonly mispronounced words, how to use em dashes (—), en dashes (–) , and hyphens (-), popular in wordplay, the words of the week - may 17, birds say the darndest things, a great big list of bread words, 10 scrabble words without any vowels, 12 more bird names that sound like insults (and sometimes are), games & quizzes.
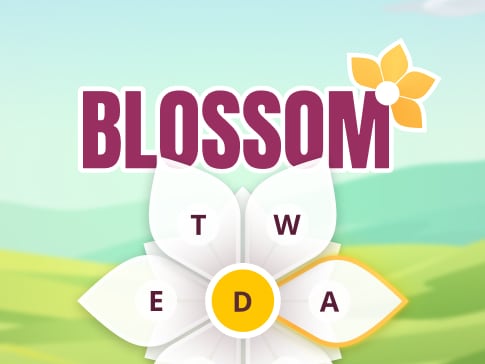
Words and phrases
Personal account.
- Access or purchase personal subscriptions
- Get our newsletter
- Save searches
- Set display preferences
Institutional access
Sign in with library card
Sign in with username / password
Recommend to your librarian
Institutional account management
Sign in as administrator on Oxford Academic
kinetic adjective & noun
- Hide all quotations
What does the word kinetic mean?
There are nine meanings listed in OED's entry for the word kinetic . See ‘Meaning & use’ for definitions, usage, and quotation evidence.
kinetic has developed meanings and uses in subjects including
Entry status
OED is undergoing a continuous programme of revision to modernize and improve definitions. This entry has not yet been fully revised.
How common is the word kinetic ?
How is the word kinetic pronounced, british english, u.s. english, where does the word kinetic come from.
Earliest known use
The earliest known use of the word kinetic is in the 1850s.
OED's earliest evidence for kinetic is from 1855, in the writing of Robert Mayne.
kinetic is a borrowing from Greek.
Etymons: Greek κῑνητικός .
Nearby entries
- kinesipath, n. 1860–
- kinesipathy, n. 1855–
- kinesis, n. 1904–
- -kinesis, comb. form
- kinesiscope, n. 1893–
- kinesodic, adj. 1874–
- kinesopathy, n. 1864–
- kine-stool, n. Old English–1872
- kine-thede, n. c1275
- kinetheodolite, n. 1941–
- kinetic, adj. & n. 1855–
- kinetical, adj. 1882–
- kinetically, adv. 1909–
- kineticism, n. 1939–
- kineticist, n. 1960–
- kinetics, n. 1864–
- kinetin, n. 1955–
- kinetite, n. 1887–
- kineto-, comb. form
- kinetochore, n. 1934–
- kinetodesma, n. 1949–
Thank you for visiting Oxford English Dictionary
To continue reading, please sign in below or purchase a subscription. After purchasing, please sign in below to access the content.
Meaning & use
Pronunciation, compounds & derived words, entry history for kinetic, adj. & n..
kinetic, adj. & n. was first published in 1901; not yet revised.
kinetic, adj. & n. was last modified in September 2023.
Revision of the OED is a long-term project. Entries in oed.com which have not been revised may include:
- corrections and revisions to definitions, pronunciation, etymology, headwords, variant spellings, quotations, and dates;
- new senses, phrases, and quotations which have been added in subsequent print and online updates.
Revisions and additions of this kind were last incorporated into kinetic, adj. & n. in September 2023.
Earlier versions of this entry were published in:
OED First Edition (1901)
- Find out more
OED Second Edition (1989)
- View kinetic, a. (n.) in OED Second Edition
Please submit your feedback for kinetic, adj. & n.
Please include your email address if you are happy to be contacted about your feedback. OUP will not use this email address for any other purpose.
Citation details
Factsheet for kinetic, adj. & n., browse entry.
- Daily Crossword
- Word Puzzle
- Word Finder
- Word of the Day
- Synonym of the Day
- Word of the Year
- Language stories
- All featured
- Gender and sexuality
- All pop culture
- Writing hub
- Grammar essentials
- Commonly confused
- All writing tips
- Pop culture
- Writing tips
Advertisement
[ ki- net -ik , kahy- ]
- pertaining to motion.
- caused by motion.
Running and dancing are kinetic activities.
bradykinetic.
/ kaɪ-; kɪˈnɛtɪk /
- relating to, characterized by, or caused by motion
Discover More
Derived forms.
- kiˈnetically , adverb
Other Words From
- ki·neti·cal·ly adverb
- nonki·netic adjective
Word History and Origins
Origin of kinetic 1
Origin of kinetic 2
Example Sentences
What looks like filthy chaos at the moment is actually the kinetic energy that gives birth to modern metropolises.
And she's walking, head bobbing, with an odd kinetic purpose, behind other people's camera shots.
There was a kinetic energy, a vibrancy that leapt off the screen that did, indeed, dazzle.
An opening that features something kinetic seems to work pretty often.
In the early 2000s, Dutschke joined a Tupelo studio called Kinetic Kick owned by Noel McMichael.
The laws of gases, it is known, are in accord with the two simple assumptions of the kinetic theory.
This represents, therefore, the pressure of such a gas, as calculated on the basis of the assumptions of the kinetic theory.
It is therefore natural to look for the cause of osmotic pressure in kinetic phenomena and not in attractions.
When a pendulum is vibrating, there is a continual transformation of potential into kinetic energy, and vice versa.
This energy, potential or latent in the coal, becomes kinetic and evident in the heat of the boiler and the work of the engine.
Related Words
Words that use -kinetic, what does -kinetic mean.
The combining form -kinetic is used like a suffix meaning “of or relating to movement.” It is often used in medical terms, especially in pathology .
The form -kinetic comes from Greek kīnēt(ós), meaning “moving,” from the verb kīneîn, “to move.” The Latin cognate of kīneîn is ciēre (stem cit-), meaning “to move, set in motion,” which is the source of words such as cite and resuscitate . To learn more, check out our entries for both words.
What are variants of -kinetic?
While there are not any variants of -kinetic, it is related to the forms -kinesis and -kinesia , as in telekinesis and hyperkinesia . Want to know more? Read our Words That Use articles about these two forms.
Examples of -kinetic
An example of a scientific term that uses the form -kinetic is electrokinetic , “of or relating to the motion of charged particles and its effects.”
As you may have guessed, electro- is a form meaning “electric” or “electricity.” The form -kinetic means “or of relating to movement.” Electrokinetic literally translates to “of or relating to electrical movement.”
What are some words that use the combining form -kinetic?
- cytokinetic
- optokinetic
- psychokinetic
- telekinetic
What are some other forms that -kinetic may be commonly confused with?
Break it down!
The combining form cyto- means “cell.” With this in mind, what does cytokinetic literally mean?

IMAGES
VIDEO
COMMENTS
kinetic theory: [noun] either of two theories in physics based on the fact that the minute particles of a substance are in vigorous motion:. a theory that the temperature of a substance increases with an increase in either the average kinetic energy of the particles or the average potential energy of separation (as in fusion) of the particles ...
Kinetic theory definition: a theory of gases postulating that they consist of particles of negligible size moving at random and undergoing elastic collisions. See examples of KINETIC THEORY used in a sentence.
2.3 Pressure, Temperature, and RMS Speed. Kinetic theory is the atomic description of gases as well as liquids and solids. It models the properties of matter in terms of continuous random motion of molecules. The ideal gas law can be expressed in terms of the mass of the gas's molecules and v2¯ v 2 ¯, the average of the molecular speed ...
kinetic theory: 1 n (physics) a theory that gases consist of small particles in random motion Synonyms: kinetic theory of gases Types: kinetic theory of heat a theory that the temperature of a body increases when kinetic energy increases Type of: scientific theory a theory that explains scientific observations
kinetic: [adjective] of or relating to the motion of material bodies and the forces and energy associated therewith.
The mean free path (the distance a molecule can move on average between collisions) of molecules in air is very small, and so the molecules move rapidly but do not get very far in a second. The high value for rms speed is reflected in the speed of sound, however, which is about 340 m/s at room temperature. ... Kinetic theory models the ...
We have seen in the Atom on "Origin of Pressure " that, for an ideal gas under our assumptions: P = Nmv2¯ 3V, (12.5.12) (12.5.12) P = N m v 2 ¯ 3 V, where P is the pressure, N is the number of molecules, m is the mass of the molecule, v is the speed of molecules, and V is the volume of the gas.
The kinetic theory of matter is the explanation for how or why matter comes in many forms or states of matter, and what makes these states different, and the changes between them possible. More ...
The theory helps explain observable properties and behaviors of solids, liquids, and gases. However, the theory is most easily understood as it applies to gases. The theory applies specifically to a model of a gas called an ideal gas. An ideal gas is an imaginary gas whose behavior perfectly fits all the assumptions of the kinetic-molecular ...
Kinetic theory of gases, a theory based on a simplified molecular or particle description of a gas, from which many gross properties of the gas can be derived. Such a model describes a perfect gas and its properties and is a reasonable approximation to a real gas. Kinetic theory of gases, a theory based on a simplified molecular or particle ...
According to the kinetic molecular theory, the average kinetic energy of an ideal gas is directly proportional to the absolute temperature. Kinetic energy is the energy a body has by virtue of its motion: KE = mv2 2 (2.6.1) (2.6.1) K E = m v 2 2. As the temperature of a gas rises, the average velocity of the molecules will increase; a doubling ...
Kinetic theory of matter definition: a theory that matter is composed of small particles, all in random motion.. See examples of KINETIC THEORY OF MATTER used in a sentence.
Kinetic theory. The kinetic particle model explains the properties of the different states of matter. Particles in solids, liquids and gases have different amounts of energy. The particles are ...
According to the kinetic theory, molecules in a liquid are in constant motion, which is an expression of their thermal energy. These molecules collide frequently, and occasionally one or another at the surface absorbs sufficient momentum to leap out of the liquid and into the atmosphere above it.
Kinetic energy is the energy an object has because of its motion. If we want to accelerate an object, then we must apply a force. Applying a force requires us to do work. After work has been done, energy has been transferred to the object, and the object will be moving with a new constant speed.
Kinetic energy is a property of a moving object or particle and depends not only on its motion but also on its mass. The kind of motion may be translation (or motion along a path from one place to another), rotation about an axis, vibration, or any combination of motions. Translational kinetic energy of a body is equal to one-half the product ...
KINETIC definition: 1. involving or producing movement: 2. involving or producing movement: 3. involving or producing…. Learn more.
KINETIC meaning: 1. involving or producing movement: 2. involving or producing movement: 3. involving or producing…. Learn more.
kinetics: [noun, plural in form but singular or plural in construction] a branch of science that deals with the effects of forces upon the motions of material bodies or with changes in a physical or chemical system. the rate of change in such a system.
History and etymology. The adjective kinetic has its roots in the Greek word κίνησις kinesis, meaning "motion".The dichotomy between kinetic energy and potential energy can be traced back to Aristotle's concepts of actuality and potentiality.. The principle in classical mechanics that E ∝ mv 2 was first developed by Gottfried Leibniz and Johann Bernoulli, who described kinetic energy ...
What does the word kinetic mean? There are nine meanings listed in OED's entry for the word kinetic. See 'Meaning & use' for definitions, usage, and quotation evidence. kinetic has developed meanings and uses in subjects including. physiology (1850s) dynamics (1860s) chemistry (1880s) cell biology (1890s) genetics (1890s) life sciences ...
Kinetic definition: pertaining to motion.. See examples of KINETIC used in a sentence.
kinetic theory of heat: 1 n a theory that the temperature of a body increases when kinetic energy increases Type of: kinetic theory , kinetic theory of gases (physics) a theory that gases consist of small particles in random motion